Abstract
Abstract: The intraperitoneal instillation of perfluorocarbons augmented systemic oxygenation and was protective in mesenteric ischemia-reperfusion and experimental lung injury. To study biocompatibility and potential anti-inflammatory effects of intraperitoneal perfluorocarbons, we evaluated the influence of perfluorohexane and/or inflammatory stimuli on human mesothelial cells in vitro. Perfluorohexane exposure neither impaired cell viability nor induced cellular activation. TNFα enhanced ICAM-1 expression, which was not attenuated by simultaneous perfluorohexane treatment. Concentration of intracellular surfactant protein A tended to be higher in perfluorohexane treated cells compared to controls. Our in vitro data add further evidence that intraperitoneal perfluorocarbon application is feasible without adverse local effects.
INTRODUCTION
Liquid, aerosolized, and vaporized perfluorocarbons (PFC) have been successfully applied in experimental and human lung injury [Citation1–5]. In addition to positive effects on gas exchange and lung mechanics, PFC administration was also associated with attenuated lung pathology and a reduced inflammatory reaction [Citation5–7]. Trauma patients with acute lung injury had fewer neutrophils and lower levels of interleukin-1 and interleukin-6 in bronchoalveolar lavage fluid after liquid ventilation with PFC [Citation8]. In a surfactant-washout model of ARDS, PFC treated animals demonstrated a substantially decreased mRNA expression of proinflammatory cytokines and intercellular adhesion molecule-1 (ICAM-1) in lung tissue samples as well as in microdissected alveolar macrophages and pulmonary epithelium [Citation3, Citation9, Citation10]. These anti-inflammatory effects may be, at least in part, explained by a direct interference with leukocyte function and lung parenchymal cells that was previously demonstrated in vitro [Citation11–15]. Our group found that perfluorohexane (PFH) attenuates inflammatory activation of isolated human alveolar macrophages and mononuclear blood cells [Citation16, Citation17]. The mechanism of this interaction is not clear. There is evidence that PFC diffuse into the lipid bilayer of biological membranes and thus alter signal transduction and cellular activation [Citation18]. However, some authors favor a “barrier effect” meaning that water-insoluble, dense PFC cover adherent lung cells and prevent the contact with activated leukocytes and mediators physically [Citation19].
In addition to pulmonary PFC application for treatment of acute lung injury, these agents have also been administered intraperitoneally for augmentation of mesenteric and systemic oxygenation. Previous studies demonstrated an attenuated intestinal damage, reduced systemic inflammation, prevention of subsequent lung injury, and improved survival in models of mesenteric ischemia-reperfusion injury [Citation20–22]. Interestingly, intraperitoneal PFC was even found to diminish pulmonary neutrophil infiltration and lung injury after acid aspiration [Citation23]. In all of these cases, PFC had direct contact with the peritoneal mesothelium. Mesothelial cells (MCs) not only constitute the surface-lining of the abdominal cavity but also play a major role in local host defense [Citation24]. They produce surfactant protein A (SP-A) that aggregates microbial pathogens, enhances phagocytosis, has antimicrobicidal activity, and modulates mediator secretion by immune cells [Citation25, Citation26]. Furthermore, inflammatory stimulation of MCs induces expression of adhesion molecules and chemotaxins that mediate leukocyte recruitment to the site of infection [Citation24, Citation27, Citation28].
Despite a series of reports on peritoneal PFC application, so far there is no data regarding an interaction with MCs. To further study PFC biocompatibility and anti-inflammatory properties on the cellular level, we evaluated the effects of PFH on resting and stimulated human MCs in terms of cell viability and expression of ICAM-1, vascular cell adhesion molecule-1 (VCAM-1), and SP-A in an in vitro model.
METHODS
Obtaining Human Omental MCs
The study was approved by the local ethics committee. After written informed consent of patients was given, human omental adipose tissue (omentum majus) was obtained during abdominal surgery for gastric or colonic cancer. Only specimens of omentum derived from patients who showed no signs of peritoneal infection or tumor spreading were used for experiments.
Preparation and Cell Culture of MCs
The MCs were prepared according to a modified method described by Pronk and coworkers [Citation29]. Within three hours after tissue removal, two pieces of omental adipose tissue (average size 2 × 2 cm) were given into 50 ml centrifugal tubes. 20 ml of 0.05% trypsin/0.02% EDTA-solution (GIBCO, Karlsruhe, Germany) were added and the tubes were shaken for seven minutes at 37°C. The enzymatic reaction was stopped by adding 20 ml of cell-culture medium (RPMI 1640 with 10% fetal calf serum (FCS), GIBCO). Supernatants were removed and centrifuged for 10 min at 280 x g and 20°C. The cell pellets were resuspended in 20 ml of RPMI 1640 with 10% FCS, penicillin (100 IE/ml), streptomycin (100 μg/ml), and Lglutamine (2 mM) (GIBCO). Cells were seeded in 75 cm2 culture flasks (Greiner Bio-One, Frickenhausen, Germany), incubated at 37°C in a 100% humidified atmosphere with 5 % CO2, and were grown to confluence as evaluated by phase-contrast light microscopy. Cells were passaged by trypsinization and were harvested for experiments after the second passage.
Characterization of MCs
MCs were characterized by light microscopy and immunocytochemistry with monoclonal mouse antibodies against human vimentin and cytokeratin (DAKO, Glostrup, Denmark). Cells (30.000 cells/cm2) were seeded on collagen-coated labtek chamber slides (Nunc, Wiesbaden, Germany), fixed with methanol-ethanol (1:1) for 20 minutes at −20°C, and stained by an indirect immunofluorescence according to a standard protocol. Slides were analyzed by fluorescence microscopy. Cell viability was assessed by trypan blue exclusion.
Experimental Protocol
150,000 cells of human omentum majus derived MCs (second passage) were seeded in gelatin-coated culture inserts with 0.4 μm pore size (Transwell Clear, Corning Costar, Bodenheim, Germany), which were placed in 6-well plates (Nunc). Cell culture medium (RPMI 1640) was added to the apical and basal compartments () and MCs were grown to confluency. For experiments, inserts were assigned to one of 7 groups (). Cells with culture medium on either side served as controls. To induce a cellular response to proinflammatory stimuli, MCs were exposed to medium containing 50 ng/ml LPS in the basal (LPS b) and 100 U/ml TNF-α in the apical (TNF a) and basal (TNF b) compartment, respectively. To study intrinsic PFH influence on MCs, PFH (C6F14, purity 99 %, ABCR, Karlsruhe, Germany, molecular weight 338 g/mol, density 1.672 g/ml, boiling point 57°C, vapor pressure (at 20°C) 177 mmHg, oxygen-carrying capacity 57 ml O2/100 ml) was layered over cells on the apical side with maintained medium supply from the lower compartment (PFH a). Finally, PFH effects on cell activation were evaluated using MCs that had contact to PFH apically and simultaneous stimulation from basal with 50 ng/ml LPS (PFH a/LPS b) and 100 U/ml TNF-α (PFH a/TNF b), respectively. Since PFH evaporates easily (boiling point of 57°C, vapor pressure of 177 mmHg at 20°C), it was added every three hours to guarantee a continuous PFH-layer on the cell surface. After an incubation time of 12 h, fresh medium was added to both compartments and cells were harvested immediately (t = 12 h) or cultured for another 24 h without PFH or inflammatory stimulants (t = 36 h).
Figure 1. Two-compartment cell culture system: MCs grown on gelatin-coated cell-culture inserts are in contact with basal and apical medium.
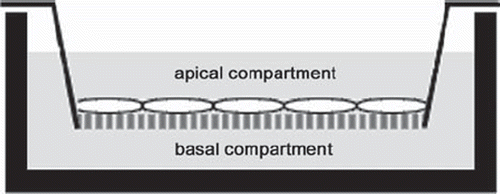
Table 1. Experimental groups.
Flow Cytometry
To prepare MCs for flow cytometry, cells were removed from inserts with trypsin/EDTA solution that, after cell detachment, was antagonized with phosphate buffered saline (PBS) containing 1% FCS and 0.1% NaN3 (Sigma, Deisenhofen, Germany). Cell viability was assessed by means of a standard trypan blue exclusion assay. For analyzing surface expression of cell adhesion molecules, MCs were washed repeatedly with PBS and incubated with primary mouse ICAM-1 and VCAM-1 antibodies (both from DAKO) for 20 minutes at 4°C. After two washing steps, a fluorescein isothiocyanate (FITC)-conjugated goat-anti-mouse secondary antibody (DAKO) was added for 15 minutes. Subsequently, cells were fixed in PBS containing 1% formaldehyde and stored at 4°C prior to analysis. To evaluate SP-A, MCs were fixed as described above, followed by two cycles of freezing and thawing to permeabilize cell membranes. Afterwards, cells were incubated for 3 h at 4°C with primary mouse antibodies against human SP-A (kind gift of Dr. M. Haase, Institute of Pathology, Technical University Dresden, Germany). Finally, cells were treated with an FITC-conjugated goat-anti-mouse antibody (DAKO) for one hour at 4°C. Additional pilot experiments evaluated SP-D expression that could be detected in only 10 – 20 % of the MCs, did not increase upon cell activation, and was hence not included in our study.
Single cell analysis was performed using a flowcytometer (EPICS XL-MCL, Beckman Coulter, Krefeld, Germany). FITC mean fluorescence intensity was measured in FL-1 and negative controls incubated with only the secondary antibody were used to correct for nonspecific fluorescence in all experiments.
Statistical Analysis
Data are presented as mean ± SEM of 6 independent experiments in all groups. To detect differences between groups, a one-way analysis of variance (ANOVA) followed by a Bonferroni post-hoc test for multiple comparison was applied. Calculations were performed using the statistical package SPSS 12.0 (SPSS, Chicago, IL) and significance was accepted at p < 0.05.
RESULTS
Characterization and Viability of MCs
Phase-contrast light microscopy of MCs revealed a characteristic cobblestone pattern and polygonal shape, whereas some single cells with an enlarged, flattened morphology were observed (). Typical for cells of mesothelial origin, all cells were strongly positive for both vimentin and cytokeratin in immunofluorescence microscopy ( and ). Untreated MCs in culture constitutionally expressed ICAM-1 and SP-A in about 90% of the cells and were also positive for VCAM-1 to a minor degree (). In flow cytometry two distinct MC populations could be discriminated in the forward vs. sideward scatter plot. About 15% of the cells had a larger diameter and higher granularity along with increased staining for cytokeratin and vimentin (not shown) that were identified as senescent MCs corresponding to flattened cells in phase-contrast microscopy [Citation30]. They were excluded from all analyses. MC viability was >90% at any time point irrespective of treatment and did not significantly differ between groups (not shown).
Figure 2. a) Light microscopy of confluent MCs with typical cobblestone pattern. The large cells are senescent MCs that accumulate with increasing passage number. b) Representative image of isolated human MCs staining positive for cytokeratin with a fluorescein isothiocyanate (FITC)-conjugated second antibody. c) Representative photomicrograph of vimentin immunocytochemistry of MCs (peroxidase-conjugated second antibody).
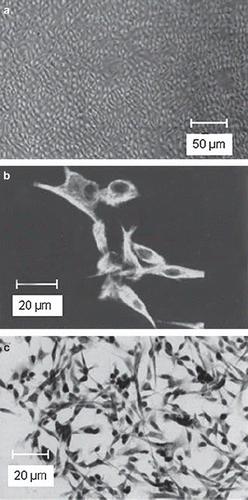
Figure 3. Representative flow cytometry histograms depicting negative controls (a, unspecific binding of FITC-conjugated secondary antibody) and constitutive expression of intercellular adhesion molecule (ICAM)-1 (b), vascular adhesion molecule (VCAM)-1 (c), and surfactant protein (SP)-A (d) in resting MCs.
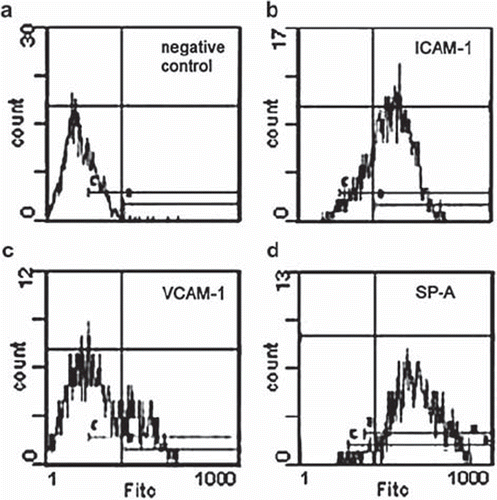
Expression of Cellular Adhesion Molecules on MCs’ Surface
Exposure of MCs to PFH (group PFH a), LPS (group LPS b) or a combination of both (group PFH a/LPS b) did not induce a significant ICAM-1 expression after 12 and 36 h, respectively (). At both time points, ICAM-1 was increased in all groups treated with TNFα (groups TNF a, TNF b, PFH a/PFH b) compared to controls and to LPS incubation (group LPS b), respectively (p < 0.05). Thereby, there was no difference between apical and basal TNFα stimulation. PFH coincubation had no influence on TNFα induced ICAM-1 expression (group PFH a/TNF b). ICAM-1 mean fluorescence in PFH exposed MCs (group PFH a) was significantly lower compared to group PFH a/TNF b after 12 h () and to all TNFα groups ((TNF a, TNF b, PFH a/PFH b) after 36 h ().
Figure 4. ICAM-1 expression after incubation for 12 (a) and 36 h (b) in percent of respective controls (= 100 %). Data represent mean ± SEM of 6 independent experiments. Respective groups are labeled according to . * p < 0.05 vs. controls; + p < 0.05 vs. TNF a, TNF b, and PFH a/TNF b; o p < 0.05 vs. PFH a; # p < 0.05 vs. PFH a/LPS b.
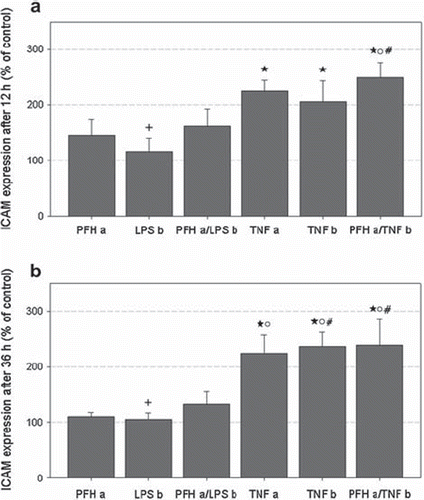
VCAM-1 expression after 12 h was weak in controls and increased by incubation with proinflammatory stimulants in a similar pattern to ICAM-1 (not shown). However, no significant differences were found between any groups. VCAM-1 values in LPS and PFH treated groups returned to control level after 36 h whereas VCAM-1 remained increased in groups TNF a and TNF b without differences reaching statistical significance (not shown).
Expression of SP-A in MCs
Compared to controls, SP-A was increased in all groups except for cells coincubated with PFH and TNFα (group PFH a/TNF b) after 12 h (). However, due to a high statistical variation of SP-A values, no significant differences could be detected between groups. Similarly, no significant alteration in SP-A expression was observed after 36 h.
Figure 5. SP-A expression after incubation for 12 (dark grey bars) and 36 h (light grey bars) in percent of respective controls (= 100 %). Data represent mean ± SEM of 6 independent experiments. Respective groups are denominated according to . No significant differences were detected between groups.
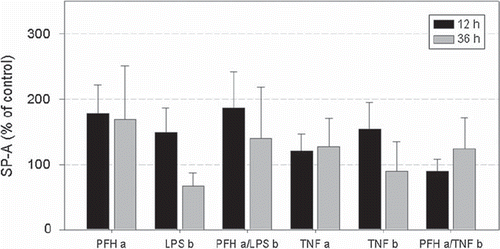
DISCUSSION
There is evidence that PFC exert beneficial effects in human and experimental acute lung injury that might be explained, at least in part, by their anti-inflammatory properties [Citation3, Citation8, Citation10]. Instillation of PFC into the abdominal cavity was associated with improved systemic oxygenation and attenuated intestinal ischemia-reperfusion injury [Citation20–22]. Rats treated with intraperitoneal PFC had decreased pulmonary permeability and neutrophil infiltration in an acid aspiration model [Citation23], most likely due to effects following systemic uptake and excretion via the lung. However, to date no study evaluated the influence of PFC on the cell population lining the peritoneal surface.
In order to further elucidate biocompatibility and anti-inflammatory properties of PFC on the cellular level we studied in an in vitro model the influence of PFH on resting and stimulated human MCs. Beside other functions, MCs have an outstanding role in local host defense against microbial pathogens and modulation of immune reactions. They express cell adhesion molecules, e. g. ICAM-1, and chemotactic mediators to control leukocyte recruitment [Citation24, Citation27, Citation28], qualifying them as an appropriate tool to analyze PFC effects on inflammatory cell activation. Primary MCs obtained from human omentum had typical cobblestone appearance at confluence and stained uniformly positive for both cytokeratin and vimentin in our experiments [Citation29, Citation30], altogether confirming their mesothelial origin. In accordance with the literature, untreated cells constitutively expressed SP-A and ICAM-1 whereas VCAM-1 expression was weak [Citation25, Citation31, Citation32]. MCs always had a viability > 90% after preparation and cell culture.
PFC are dense, hydrophobic substances and not soluble in cell culture medium. To analyze PFC interaction with adherent MCs we used cells grown on microporous membranes to guarantee constant PFH exposure from the apical side and a sufficient medium supply and cell stimulation from basal simultaneously. Furthermore, the use of this three-dimensional system prevents PFH to function as a barrier, separating inflammatory mediators and cell monolayers, and to bind/inactivate the respective stimulant unspecifically. Both mechanisms were proposed to be the cause of anti-inflammatory PFC effects [Citation19, Citation33].
The exposure of resting MCs with PFH did not alter the percentage of viable cells, thus demonstrating its good biocompatibility that was shown previously in human leukocytes [Citation16, Citation17]. PFH per se did not induce cellular adhesion molecules significantly and thus is not considered to have intrinsic proinflammatory activity in MCs. Likewise, exposure of human endothelial cells with emulsified and pure PFC (perfluorooctylbromide) in a similar model was not associated with cell activation as measured by means of ICAM-1 and E-selectin [Citation33, Citation34]. We found that weak constitutive VCAM-1 expression can be induced by LPS and TNFα treatment, but this did not reach statistical significance. In contrast, MC stimulation with TNFα significantly raised ICAM-1 irrespective of whether the cells were activated from apical or basal. However, PFH was not able to attenuate the increase in ICAM-1 stimulated by TNFα, and respective values were the highest of all groups. LPS in a concentration of 50 ng/ml was shown to promote homologous adhesion of mesothelial monolayers and enhance mesothelial permeability [Citation35], but did not induce an inflammatory response in our experiments. This is in contrast to a recent study that reported increased ICAM-1 mRNA and protein in rat MCs after LPS stimulation [Citation36].
SP-A is expressed in human peritoneum and isolated MCs [Citation25], and SP-A secretion plays a crucial role for pathogen agglutination, antigen presentation, opsonization, and modulation of peritoneal immune response [Citation26]. Constitutive SP-A expression in MCs was not significantly altered after treatment with LPS, TNFα, and PFH, given either alone or in combination. PFH exposure was associated with the highest SP-A levels of all groups, confirming biocompatibility and arguing against concerns about a compromised local immune defense during intraperitoneal PFC application in this regard. Furthermore, unchanged cellular SP-A content after proinflammatory stimulation is in contradiction to reports of substantial SP-A downregulation following TNFα stimulation [Citation37, Citation38]. However, so far all studies evaluated TNFα effects in alveolar epithelial cells. MCs share some similarities with Typ II pneumocytes, e. g. expression of cytokeratin and secretion of phosphatidylcholine and SP-A, but lack other surfactant components (SP-B and SP-C) [Citation25]. Therefore, results can not be compared directly between both cell populations. To our knowledge, this is the first report on SP-A expression in human MCs after proinflammatory activation.
Chemotaxis, secretion of cytokines, production of reactive oxygen species, and other leukocyte functions were decreased after PFC incubation in vitro [Citation11–14]. In recent studies we found PFH to attenuate the proinflammatory and procoagulatory response of stimulated human monocytes, alveolar macrophages, and lymphocytes [Citation16, Citation17]. However, there seems to be no intrinsic anti-inflammatory potential of PFH on activation of adherent MCs in terms of TNFα-induced ICAM-1 expression. Apical cell exposure with PFH and stimulation from basal precluded a direct interaction of the agents and guaranteed an unimpaired contact of TNFα with the cell surface. Our results support therefore the theory of a “physical barrier” for anti-inflammatory PFC effects on adherent cells that was stated previously [Citation19]. On the other hand, PFC preincubation of human endothelial cells inhibited ICAM-1 and E-selectin expression as well as neutrophil adhesion after stimulation with TNFα/Interleukin-1β and LPS in a similar two-compartment model [Citation34]. In this and in another study a time-dependent diffusion of PFC into cellular membranes and thus altered cell response to activation were noted [Citation18]. Simultaneous application of PFH and TNFα in our experiments might have resulted in PFC membrane concentrations too low to influence TNFα receptor binding or subsequent signal transduction.
CONCLUSION
In summary, we for the first time report PFC exposure of cultured human MCs that is associated with good biocompatibility and does not result in cell activation or alteration of cellular SP-A concentration. Our in vitro data add further evidence that intraperitoneal PFC application is feasible without adverse local effects. However, intrinsic anti-inflammatory effects were not observed since PFH did not influence TNFα-induced ICAM-1 expression.
Declaration of interest: The authors report no conflicts of interest. The authors alone are responsible for the content and writing of the paper. Funding came from the Department of Anesthesiology and Intensive Care Medicine.
REFERENCES
- Hirschl, R. B., Pranikoff, T., Wise, C., Overbeck, M. C., Gauger, P., Schreiner, R. J., Dechert, R., Bartlett, R. H. (1996). Initial experience with partial liquid ventilation in adult patients with the acute respiratory distress syndrome. JAMA, 275: 383–389.
- Ricard, J. D., Iserin, F., Dreyfuss, D., Saumon, G. (2007). Perflubron dosing affects ventilator-induced lung injury in rats with previous lung injury. Crit Care Med, 35: 561–567.
- von der Hardt, K., Schoof, E., Kandler, M. A., Dotsch, J., Rascher, W. (2002). Aerosolized perfluorocarbon suppresses early pulmonary inflammatory response in a surfactant-depleted piglet model. Pediatr Res, 51: 177–182.
- de Abreu, M. G., Quelhas, A. D., Spieth, P., Brauer, G., Knels, L., Kasper, M., Pino, A. V., Bleyl, J. U., Hubler, M., Bozza, F., Salluh, J., Kuhlisch, E., Giannella-Neto, A., Koch, T. (2006). Comparative effects of vaporized perfluorohexane and partial liquid ventilation in oleic acid-induced lung injury. Anesthesiology, 104: 278–289.
- Bleyl, J. U., Heller, A. R., Heintz, M., Schlemmer, M., Koch, R., de Abreu, M. G., Hubler, M., Spieth, P. M., Koch, T. (2010). Effects of perfluorohexane vapor in the treatment of experimental lung injury. Pulm Pharmacol Ther, 23: 450–455.
- Rotta, A. T., Steinhorn, D. M. (1998). Partial liquid ventilation reduces pulmonary neutrophil accumulation in an experimental model of systemic endotoxemia and acute lung injury. Crit Care Med, 26: 1707–1715.
- Jiang, L., Wang, Q., Liu, Y., Du, M., Shen, X., Xie, N., Wu, S. (2007). Effect of different ventilation modes with FC-77 on pulmonary inflammatory reaction in piglets after cardiopulmonary bypass. Pediatr Pulmonol, 42: 150–158.
- Croce, M. A., Fabian, T. C., Patton, J. H., Jr., Melton, S. M., Moore, M., Trenthem, L. L. (1998). Partial liquid ventilation decreases the inflammatory response in the alveolar environment of trauma patients. J Trauma, 45: 273–280.
- Schoof, E., von der Hardt, K., Kandler, M. A., Abendroth, F., Papadopoulos, T., Rascher, W., Dotsch, J. (2002). Aerosolized perfluorocarbon reduces adhesion molecule gene expression and neutrophil sequestration in acute respiratory distress. Eur J Pharmacol, 457: 195–200.
- von der Hardt, K., Kandler, M. A., Fink, L., Schoof, E., Dotsch, J., Bohle, R. M., Rascher, W. (2003). Laser-assisted microdissection and real-time PCR detect anti-inflammatory effect of perfluorocarbon. Am J Physiol Lung Cell Mol Physiol, 285: L55–L62.
- Smith, T. M., Steinhorn, D. M., Thusu, K., Fuhrman, B. P., Dandona, P. (1995). A liquid perfluorochemical decreases the in vitro production of reactive oxygen species by alveolar macrophages. Crit Care Med, 23: 1533–1539.
- Chang, H., Kuo, F. C., Lai, Y. S., Chou, T. C. (2005). Inhibition of inflammatory responses by FC-77, a perfluorochemical, in lipopolysaccharide-treated RAW 264.7 macrophages. Intensive Care Med, 31: 977–984.
- Rossman, J. E., Caty, M. G., Rich, G. A., Karamanoukian, H. L., Azizkhan, R. G. (1996). Neutrophil activation and chemotaxis after in vitro treatment with perfluorocarbon. J Pediatr Surg, 31: 1147–1150.
- Thomassen, M. J., Buhrow, L. T., Wiedemann, H. P. (1997). Perflubron decreases inflammatory cytokine production by human alveolar macrophages. Crit Care Med, 25: 2045–2047.
- Chang, L. P., Lai, Y. S., Wu, C. J., Chou, T. C. (2009). Liquid perfluorochemical inhibits inducible nitric oxide synthase expression and nitric oxide formation in lipopolysaccharide-treated RAW 264.7 macrophages. J Pharmacol Sci, 111: 147–154.
- Koch, T., Ragaller, M., Haufe, D., Hofer, A., Grosser, M., Albrecht, D. M., Kotzsch, M., Luther, T. (2001). Perfluorohexane attenuates proinflammatory and procoagulatory response of activated monocytes and alveolar macrophages. Anesthesiology, 94: 101–109.
- Haufe, D., Luther, T., Kotzsch, M., Knels, L., Koch, T. (2004). Perfluorocarbon attenuates response of concanavalin A-stimulated mononuclear blood cells without altering ligand-receptor interaction. Am J Physiol Lung Cell Mol Physiol, 287: L210–L216.
- Obraztsov, V. V., Neslund, G. G., Kornbrust, E. S., Flaim, S. F., Woods, C. M. (2000). In vitro cellular effects of perfluorochemicals correlate with their lipid solubility. Am J Physiol Lung Cell Mol Physiol, 278: L1018–L1024.
- Baba, A., Kim, Y. K., Zhang, H., Liu, M., Slutsky, A. S. (2000). Perfluorocarbon blocks tumor necrosis factor-alpha-induced interleukin-8 release from alveolar epithelial cells in vitro. Crit Care Med, 28: 1113–1118.
- Ohara, M., Unno, N., Mitsuoka, H., Kaneko, H., Nakamura, S. (2001). Peritoneal lavage with oxygenated perfluorochemical preserves intestinal mucosal barrier function after ischemia-reperfusion and ameliorates lung injury. Crit Care Med, 29: 782–788.
- Yamamoto, N., Unno, N., Mitsuoka, H., Uchiyama, T., Saito, T., Konno, H. (2005). Peritoneal lavage with oxygenated perfluorochemical improves hemodynamics, intestinal injury, and survival in a rat model of severe hemorrhagic shock and resuscitation. Shock, 24: 171–176.
- Saito, T., Unno, N., Yamamoto, N., Inuzuka, K., Sagara, D., Suzuki, M., Konno, H. (2006). Intraperitoneal administration of hyperbarically oxygenated perfluorochemical enhances preservation of intestinal mucosa against ischemia/reperfusion injury. Shock, 26: 620–624.
- Nader, N. D., Knight, P. R., Davidson, B. A., Safaee, S. S., Steinhorn, D. M. (2000). Systemic perfluorocarbons suppress the acute lung inflammation after gastric acid aspiration in rats. Anesth Analg, 90: 356–361.
- Mutsaers, S. E. (2002). Mesothelial cells: their structure, function and role in serosal repair. Respirology, 7: 171–191.
- Chailley-Heu, B., Rubio, S., Rougier, J. P., Ducroc, R., Barlier-Mur, A. M., Ronco, P., Bourbon, J. R. (1997). Expression of hydrophilic surfactant proteins by mesentery cells in rat and man. Biochem J, 328: 251–256.
- Wright, J. R. (2005). Immunoregulatory functions of surfactant proteins. Nat Rev Immunol, 5: 58–68.
- Jonjic, N., Peri, G., Bernasconi, S., Sciacca, F. L., Colotta, F., Pelicci, P., Lanfrancone, L., Mantovani, A. (1992). Expression of adhesion molecules and chemotactic cytokines in cultured human mesothelial cells. J Exp Med, 176: 1165–1174.
- Cannistra, S. A., Ottensmeier, C., Tidy, J., DeFranzo, B. (1994). Vascular cell adhesion molecule-1 expressed by peritoneal mesothelium partly mediates the binding of activated human T lymphocytes. Exp Hematol, 22: 996–1002.
- Pronk, A., Leguit, P., Hoynck van Papendrecht, A. A., Hagelen, E., van Vroonhoven, T. J., Verbrugh, H. A. (1993). A cobblestone cell isolated from the human omentum: the mesothelial cell; isolation, identification, and growth characteristics. In Vitro Cell Dev Biol, 29A: 127–134.
- Zhang, X. Y., Pettengell, R., Nasiri, N., Kalia, V., Dalgleish, A. G., Barton, D. P. (1999). Characteristics and growth patterns of human peritoneal mesothelial cells: comparison between advanced epithelial ovarian cancer and non-ovarian cancer sources. J Soc Gynecol Investig, 6: 333–340.
- Liang, Y., Sasaki, K. (2000). Expression of adhesion molecules relevant to leukocyte migration on the microvilli of liver peritoneal mesothelial cells. Anat Rec, 258: 39–46.
- Klein, C. L., Bittinger, F., Skarke, C. C., Wagner, M., Kohler, H., Walgenbach, S., Kirkpatrick, C. J. (1995). Effects of cytokines on the expression of cell adhesion molecules by cultured human omental mesothelial cells. Pathobiology, 63: 204–212.
- Lane, T., Smith, D., Wancewicz, E., Funk, R. (1993). Inhibition of endotoxin-mediated activation of endothelial cells by a perfluorocarbon emulsion. Biomater Artif Cells Immobilization Biotechnol, 21: 163–172.
- Woods, C. M., Neslund, G., Kornbrust, E., Flaim, S. F. (2000). Perflubron attenuates neutrophil adhesion to activated endothelial cells in vitro. Am J Physiol Lung Cell Mol Physiol, 278: L1008–L1017.
- Toh, H., Torisu, M., Shimura, H., Kitsuki, H., Uchiyama, A., Itoh, H., Ohsato, K. (1996). In vitro analysis of peritoneal adhesions in peritonitis. J Surg Res, 61: 250–255.
- Zhang, Y. F., Yang, X., Zhang, Y. J., Sun, Y. L., Zou, X. L., Kong, Q. Y., Dong, X. Q., Ye, X. Q., Yu, X. Q. (2006). Peroxisome proliferator-activated receptor-gamma is expressed by rat peritoneal mesothelial cells: its potential role in peritoneal cavity local defense. Am J Nephrol, 26: 602–611.
- Pryhuber, G. S., Khalak, R., Zhao, Q. (1998). Regulation of surfactant proteins A and B by TNF-alpha and phorbol ester independent of NF-kappa B. Am J Physiol, 274: L289–L295.
- Miakotina, O. L., Snyder, J. M. (2002). TNF-alpha inhibits SP-A gene expression in lung epithelial cells via p38 MAPK. Am J Physiol Lung Cell Mol Physiol, 283: L418–L427.