Abstract:
We have developed a strategy to immobilize β-galactosidase as a model enzyme by using polymeric supports having Schiff bases, which were prepared from (aminomethyl)polystyrene and 2-phenlyindole-3-carboxaldehyde by condensation. β-galactosidase was immobilized onto the new polymer supports via covalent bonds. The influence of temperature, pH, reusability, and storage capacity on the free and immobilized β-galactosidase was investigated. Our results indicate that the (aminomethyl)polystyrene with Schiff bases is most suitable for the immobilization of β-galactosidase. These kinds of new supports can be used for the immobilization of β-galactosidase due to their strong storage capacity and reusability.
INTRODUCTION
The β-galactosidase is a hydrolase enzyme that catalyzes the hydrolysis of β-galactosides into monosaccharides [Citation1, Citation2]. β-galactosidases from different sources are currently used in the production of lactose-hydrolyzed milk, galactooligosaccharides (GOS) formation, and in carbohydrate synthetic chemistry [Citation3, Citation4, Citation5]. Although β-galactosidases have attracted more interests in the varying industrial process, these enzymes are unstable due to their complex molecular structure. Therefore, a number of immobilization techniques have recently been investigated to improve their stabilities [Citation6, Citation7].
Immobilization of enzymes onto solid carriers is the most useful strategy to improve the operational stability of biocatalysts. Other benefits are obtained as well, such as better operational control, flexibility of reactor design, and ease of product recovery without catalyst contamination [Citation3]. To date, different methods have been used for enzyme immobilization such as adsorption onto magnetite [Citation8], entrapment in fiber [Citation9], and covalent attachment onto solid carriers (i.e., polystyrene and granular polyamine) [Citation10, Citation11, Citation12]. One of the most useful immobilization methods is covalent attachment of enzyme onto a solid support. Generally, there are two types of covalent attachment strategies. One is one point covalent attechment; the other is multipoint covalent attachment. In the one point covalent attechment, only one (or two) functional groups of the enzyme are connected to the polymer support via covalent binding. In the multipoint case, all parts of the enzyme can be connected to the solid carrier via covalent bonds [Citation13, Citation14].
Some studies on modified support have been reported for immobilization of enzyme [Citation15, Citation16]. They have investigated immobilization properties of the epoxy-activated supports, glyoxyl supports, and aminated supports. Agostinelli et al. investigated a new support on polyketone polymers for enzyme immobilization [Citation17].
A lot of studies on polystyrene supported-Schiff base, oxidation [Citation18], and ion selectivity [Citation19] can be found in the literature. Studies on polymers having Schiff bases have been increasing in recent years. Schiff bases have –CH = N-group. –CH = N-group has sp2 hybridized. The presence of a lone pair of electrons in a sp2 hybridized orbital of nitrogen atom of the azomethine group is biologically important. The azomethine group of the Schiff bases is involved in many different biological processes such as decarboxylation, transamination, electron transfer, etc.
Herein, we developed strategy to immobilize β-galactosidese by using polymeric supports having Schiff bases which were prepared from (aminomethyl)polystyrene and 2-phenlyindole-3-carboxaldehyde by condensation. To the best of our knowledge there is no report in the literature about polymer support involving Schiff bases or Schiff bases-Ni(II) complexes for immobilization of enzyems. To prepare such a polymeric support, the (aminomethyl)polystyrene reacted with 2-phenlyindole-3-carboxaldehyde (APS-2PIn-Sch; ) and then prepared the metal-containing complex using NiCl2·6H2O salts (APS-2PIn-Sch-Ni; )
MATERIALS AND METHODS
Chemicals
The β-galactosidase (EC.3.2.1.23 from Escherichia coli), p-nitrophenyl-β-D-galactopiranosyde, (aminomethyl)polystyrene, 2-phenlyindole-3-carboxaldehyde, and NiCl2·6H2O were purchased from Sigma (St. Louis, MO). All the other chemicals used in this work were provided by Merck and Sigma and used without furher purification.
Apparatus for Characterization
Nitrogen analyses were carried out with an Elementar Micro Vario CHNS instrument. Nickel contents were determined by using a Coupling of Liquid Chromatography instrument. Mass spectrum was recorded on a Micro Mass-UK Platform II mass spectrometer. Electronic spectra were recorded with Unicam-UV2-100 spectrophotometer in dimethylformamide. IR spectra were recorded with a Mattson-5000 FT-IR instrument in KBr pellets. Thermogravimetric analyses (TGA) of (APS), (APS-2PIn-Sch), and (APS-2PIn-Sch-Ni) were carried out on a Dupont 951 Model equipment (Dupont, Boston, MA) at a temperature range of 0-600°C. A heating rate of 10°C/min under nitrogen atmosphere was maintained for TGA analyses. Scanning electron microscopy of (APS), (APS-2PIn-Sch), and (APS-2PIn-Sch-Ni) were done by using a JEOL JEM 100 CX II electron microscope (JEOL, Peabody, MA).
Synthesis of Polymer-bound Schiff-base (APS-2PIn-Sch)
The polymers having Schiff bases were prepared dropwise with addition of (aminomethyl)polystyrene [(APS); 1g, 0.5-1.0 mmol/g NH2 loading] in hot methanol (15 mL) to a solution of 2-phenlyindole-3-carboxaldehyde (0.5 mmol, g) in a hot methanol (10 mL) while stirring. The stirring was continued until the solution was refluxed ca. two days later. Then the mixture was cooled, and a crystalline, clear yellow precipitate was obtained (APS-2PIn-Sch; ). The crystalline solid was filtered, washed with methanol and dried, and kept with desiccator over anhydrous CaCl2.
Synthesis of Polymer-bound Schiff-base-Ni(II) Complexes (APS-2PIn-Sch-Ni)
First, the nickel-aldehyde complexes (2PIn-Ni) were prepared. Methanol solutions of NiCl2•6H2O were mixed with methanolic solutions of 2-phenlyindole-3-carboxaldehyde (2PIn) in a 1:1 mole ratio. The resulting solutions were stirred for about 1 h and filtered. After standing for 8 h, yellow solid complexes formed; these were collected by filtration, washed with a small volume of diethyl ether, and dried in a desiccator over CaCl2 (Ni-Aldehyde complexes; ). The results obtained show that the structure of complex is the ML complex according to the LC-Mass spectroscopy [M+7H]+ 408 (m/z = 76%) for (Ni-aldehyde).
Next, the polymer-bound Schiff-base-Ni(II) complexes (APS-2PIn-Sch-Ni) was prepared by adding a solution of APS (1g, involving 0.5 mmole –NH2) in hot methanol (15 mL) was added to a solution of the (Ni-Ala) complexes (0.20 g, 0.5 mmol) in methanol (10 mL). The solution was stirred until its color changed (∼two days). After cooling, the complexes were washed repeatedly with methanol and then dried. The colored crystallines are soluble in DMF.
Immobilization of β-galactosidase on (APS-2PIn-Sch) and (APS-2PIn-Sch-Ni)
The (APS-2PIn-Sch) and (APS-2PIn-Sch-Ni) polymers (1 g) were placed in a 20 mL solution of 3,3 mg β-galactosidase at 30°C in a shaking water bath for 5 h. The polymers were separated and the free enzyme was removed by washing with phosphate buffer (pH: 4.3, 0.2 M, 15 mL). We calculated the total enzyme immobilization amount at 2.3 mg/g polymeric beads. The immobilized enzymes were stored at 4°C in buffer solution used in further experiments.
Enzyme Assay or Determination of β-galactosidase Activity
The β-galactosidase activities of both the soluble and immobilize enzyme were determined as described by Hernaiz and Crout [Citation20]. Briefly, a sample of enzyme (soluble enzyme, 20 μL, 0.0015 mM; immobilized enzyme 0.05 g) was added to 0.09 ml of Britton-Robinson (B-R) buffer (pH: 5.0) containing 5 mM p-nitrophenyl β-D-galactoside. The reaction mixture was incubated for 10 min at 30°C. Absorbency was measured at 398 nm upon addition of Na2CO3 solution (0.1 M). One unit of enzyme activity is defined as that quantity of enzyme hydrolyzing 1 mmol of p-nitrophenyl β-D-galactoside under the conditions stated above. The enzymatic activities were determined by the slope of the calibration curve.
Storage Stability and Reusability of Immobilized Enzyme
Storage stability experiments were carried out to determine the stabilities of free and immobilized enzymes after storage in B-R buffer at 4 C for 90 days. To evaluate the reusability, the β-galactosidase immobilized polymeric supports were also washed with B-R buffer solution after any run and re-introduced into a fresh solution. Ten-minute reaction cycles under the conditions described above were performed. The residual activities of free and immobilized enzymes were then determined as described in the above section. Observed results compared to the initial activities.
RESULTS AND DISCUSSION
Characterization Study
The spectral characterization and microanalytical data of the (APS-2PIn-Ald) and (APS-2PIn-Ald-Ni) complexes are given in and , respectively. The analytical data of the complexes in a good agrement with suggested structure. The mmol/g N loading are found to be 1.03 and 1.01 for (APS-2PIn-Ald) and (APS-2PIn-Ald-Ni), respectively.
Table 1. Analytical and physical data of the studied polymer.
Table 2. Ir bands (cm−1), electronic spectral (nm) (εmax, mol−1cm−1L), and thermal analysis data of the studied polymers.
IR Spectra and Electronic Absorption Spectra. summarizes the main IR bandsof (APS), (APS–2PIn-Sch), (APS–2PIn-Sch–Ni). IR bands in the 2963 cm−1 and 2872 cm−1 are characteristic of ν(CH)as and ν(CH)s for aliphatic –CH2 group. Bands appearing at 3004 cm−1 and 3110 cm−1 are assigned to ν(CH)as and ν(CH)s vibrations due to aromatic –CH for the (APS–2PIn-Sch) and (APS–2PIn-Sch–Ni). For (APS–2PIn-Sch), the new band showed that at 1587 cm−1 is assigned to –CH = N. The azomethine in the IR spectra of the complexes appear in the 1571 cm-1, somewhat lower than observed for the free ligands. For (APS–2PIn-Sch-Ni), the band at 410 cm-1 can be assigned to ν(Ni-N) [Citation21].
The electronic spectra of the (APS), (APS–2PIn-Sch), and (APS–2PIn-Sch–Ni) recorded in DMF are shown in . A strong absorption in the 254-302 nm range is due to an Intra-ligand-Charge-Transfer (ILCT) electronic transition. (APS–2PIn-Sch–Ni) complexes show two distinct broad absorption bands peaking at 320 and 416 nm, which were attributed to ligant-to-ligant and metal-to-ligant charge transfer, respectively (LLCT, MLCT). (APS–2PIn-Sch–Ni) complex shows two new d-d bands at 693 nm and 620 nm, indicating a tetrahedral or pseudotetrahedral structure () [Citation22, Citation23]. These results show that added of aldehyde to polymer.
Thermogravimetric Analysis and Scanning Electron Microscopy. Thermal data of the (APS), (APS–2PIn-Sch), and (APS–2PIn-Sch–Ni) are given in . As can be seen from , the decomposition temperatures (Ti, T½, Tm, Tf) of the polymers are different. The (APS)-polymers are thermally decomposed in a single step. According to the initial decomposition temperature (Ti), (APS–2PIn-Sch) has the highest thermal stability with a Ti 391°C. This decomposition occurs within the temperature range, 391-430°C. All of the synthesized polymer-metal complexes exhibit adequate thermal stability.
SEM micrographs of (APS), (APS–2PIn-Sch), and (APS–2PIn-Sch–Ni), as is shown in , indicate that the morphological structure of (APS–2PIn-Sch) and (APS–2PIn-Sch–Ni) are different from that of (APS). (APS–2PIn-Sch) and (APS–2PIn-Sch–Ni) show a more collapsed morphological structure than (APS). These states may result from deviation of H2O due to condensation. The immobilized polymers have different morphologies, which may result from the covalent attachment of the enzyme. Probably, an amide bond (), a hydrogen bond () and a coordinate covalent bond () form because of covalent attachment of the enzyme. The morphological structures of (APS–2PIn-Sch) and (APS–2PIn-Sch–Ni) are different from those of the immobilized polymers.
Immobilization Study
Effect of pH on Immobilization. The pH is one of the important parameters influencing enzymatic activities in aqueous solution [Citation24]. Immobilization of an enzyme is likely to result in conformational changes, which may affect its optimum pH. The effect of pH was studied over the pH range of 2.0-9.0 at 30°C. The pH dependence of the immobilized β-galactosidase activity was compared with that of the free enzyme in .
Figure 3. Effect of pH (A) and temperature (B) on enzyme activity and effect of reuse of immobilized β-galactosidase (C).
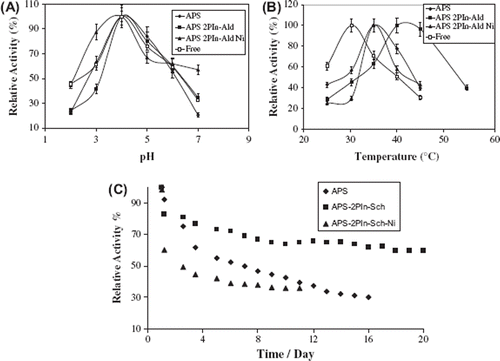
The maximum activities for the free and immobilized enzyme were at pH 4.0. Generally, a shift in activity after immobilization is expected, because the microenvironment of the free enzyme is different [Citation12, Citation25]. In this study, the optimal pH remained the same due to limited multiple covalent attachment.
Effect of Temperature on Immobilization. The effect of temperature on the activity of free and immobilized β-galactosidase is shown in . Experiments were done over a temperature range of 25–55°C. The maximum activity was observed at 30°C for free enzyme, at 35°C for (APS), at 40°C for (APS–2PIn-Ald–Ni) and (APS–2PIn-Ald). Depending on the immobilization process, the thermal stability of an immobilized enzyme can be improved, diminished, or be unchanged relative to the free enzyme. In our case, we found that the optimum temperature for the immobilized β-galactosidase was higher than that of the free enzyme, due to the conformational limitations on the enzyme movement and limited multiple covalent interactions between the enzyme and the polymer. In addition to these, the shift in the temperature indicated a greater rigidity of the multi-attached immobilized β-galactosidase molecule that was more resistant to unfolding at higher temperatures than the free form [Citation26]. Several studies using different kinds of galactosidase from varying sources have been reported in which the thermal stability can be increased by several degrees based on immobilization [Citation27, Citation28]. The high thermostability of β-galactosidase would be a great advantage for industrial applications.
Storage Stability. The most important characteristic future of an enzyme is its stability. In general, enzymes often lose their activities during the storage. Free and immobilized enzymes of β-galactosidase were stored in Britton-Robinson (B-R) buffer solution at 4°C for 90 days. After 30 days, the free β-galactosidase retained 10% of its original activity. After 90 days of storage, covalently bonded (APS), (APS–2PIn-Ald), and (APS–2PIn-Ald–Ni) enzymes had retained 94%, 98%, and 99% of their original activities, respectively. Very high activities were found for all modified polymers, especially for the (APS–2PIn-Ald–Ni) support system. These results suggest that the (APS), (APS–2PIn-Ald), and (APS–2PIn-Ald–Ni) supports confer a higher conformational stability upon the immobilized enzyme due to amide bound, hydrogen bound, and coordination of metal bound, respectively (). The reason is not clear at present and there are no such similar observations reported in the literature.
Reuse of β-galactosidase. Reusability is advantageous for reducing the cost of the operation [Citation29, Citation30]. The immobilized samples were used repeatedly 10-20 times in a day; their relative activities are presented in . After the twentieth use of (APS–2PIn-Sch), the immobilized enzyme retained nearly 68% of its original activity. After the sixteenth use of (APS) and tenth use of (APS–2PIn-Sch–Ni), the immobilized enzyme had only 30% and 37% retained of its original activity. Our results indicate that the covalent binding of β-galactosidase using Schiff bases helped the enzyme to retain catalytic activity when it was repeatedly used in various cycles.
Kinetic Parameters. The activities of the free and immobilized enzymes with various substrate concentrations were plotted as Lineweaver-Burk graphs to calculate Vmax and Km values (). The Vmax value defines the maximum velocity when all of the enzyme is saturated with substrate. Km, the substrate concentration at which an enzyme reaches ½ Vmax, reflects the effective characteristics of the enzyme and depends upon both partitioning and diffusion [Citation31].
The effect of the substrate concentration on the reaction rate was studied using varying initial concentrations (0.015–0.25 mM) of p-nitrophenyl-β-D-galactopiranosyde substrate. The Michaelis–Menten constant (Km) and the maximum reaction rate (Vmax) of free and immobilized β-galactosidase were calculated from the Lineweaver–Burk plots (). The Km/Vmax values were calculated to be 0.343 mM/0,0259 mMmin−1 for free β-galactosidase and 0,681 mM/0.0716 mMmin−1, 0,0363 mM/0.0185 mMmin−1, and 0,380 mM/0.0718 mMmin−1 for immobilized β-galactosidase to the (APS), (APS–2PIn-Sch), and (APS–2PIn-Sch–Ni) supports, respectively. Km values of immobilized β-galactosidase to the (APS) and (APS–2PIn-Sch–Ni) are higer than free β-galactosidase due to amide band () and coordination structure (), respectively. Km values of immobilized enzyme were found to be higher than that of the free enzyme [Citation32]. However, lower Km values mean high enzyme affinity to the substrate, so we can infer that the immobilization using (APS–2PIn-Sch) enzymes show more affinity to the substrate than (APS) and (APS–2PIn-Sch–Ni) because the hydrogen band is weaker than than amid band and coordination band in the between (APS–2PIn-Sch) and enzyme. There is an apparent increase in the Vmax values upon immobilization. This can be explained because of an interaction between the substrate and the support or the conformational changes of the enzyme resulting in a lower possibility to form a substrate-enzyme complex [Citation33].
CONCLUSION
In this study, we have developed a novel strategy for the immobilization of β-galactosidase based on Schiff bases modified polymeric supports, which are synthesized from condensation of polymer having –NH2 with 2-phenlyindole-3-carboxaldehyde. The maximum activities for both free and immobized enzymes were found at pH = 4.0. We also observed that the immobilized form of enzyme compared to the free one is much more stable at high temperatures due to the creation of conformational limitation on the enzyme movement. Furthermore, operational and storage stabilities of β-galactosidase were dramatically improved based on immobilization. These kinds of superior properties of our developed immobilization strategy would be great advantages for various industrial applications, not only for β-galactosidase but other enzymes.
Declaration of interest: The authors report no conflicts of interest. The authors alone are responsible for the content and writing of the paper.
REFERENCES
- Distler, J.J., Jourdian, G.W. (1973). The purification and properties of β-galactosidase from bovine testes. J Biol Chem. 248:6772–6780.
- Albayrak, N., Yang, S.-T. (2002). Immobilization of β-galactosidase on fibrous matrix by polyethyleneimine for production of galacto-oligosaccharides from lactose. Biotechnology Progress. 18:240–251.
- Feng, Y., Chang, X., Wang, W., Ma, R. (2010). Stabilities of immobilized b-galactosidase of aspergillus sp. AF for the optimal production of galactooligosaccharides from lactose. Artificial Cells, Blood Substitutes, and Biotechnology. 38: 43–51.
- Neri, D.F.M., Balcão, V.M., Dourado, F.O.Q., Oliveira, J.M.B., Carvalho Jr., L.B., Teixeira, J.A. (2009). Galactooligosaccharides production by b-galactosidase immobilized onto magnetic polysiloxane–polyaniline particles. React and Funct Polym. 69:246–251.
- Mozaffer, Z., Nakanishi, K., Matsuno, R., Kamikubo, T. (1984). Purification and properties of β-galactosidase from Bacillus circulans. Agric. Biol. Chem. 48:3053–3061.
- Tanrıseven, A., Doğan, S. (2002). A novel method for the immobilization of β-galactosidase. Process Biochemistry. 38:27–30.
- Zhou, Q. Z. K., Chen, X. D. (2001). Immobilization of β-galactosidase on graphite surface by glutaraldehyde. Journal of Food Engineering; 48:69–74.
- Kubal, B.S., Sankaran, K., D'Souza, S.F. (1989). Immobilization of yeast by invertase adsorpstion on magnetite. J Microb Biotechnol. 4:98–102.
- Arruda, L.M., Vitolo, M. (1999). Characterization of invertase entrapped into calcium alginate beads. Appl Biochem Biotechnol. 1:23–33.
- Mansfeld, A., Schellenberger, A. (1987). Invertase immobilized on macroporous polystyrene: Propeties and kinetic characterization. Biotechnol Bioeng. 29:72–78.
- Dizge, N., Gunaydin, O., Yilmaz, F., Tanriseven, A. (2008). Immobilization of invertase onto poly(3-methylthienyl methacrylate)/poly(3-thiopheneacetic acid) matrix. Biochem Eng J. 40:64–71.
- Lopez-Gallego, F., Montes, T., Fuentes, M., Alonso, N., Grazu, V., Betancor, L., Guisan, J.M., Fernandez-Lafuente, R. (2005). Improved stabilization of chemically aminated enzymes via multipoint covalent attachment on glyoxyl suppports. J Biotechnol. 116:1–10.
- Blanco, R.M., Guisan, J.M. (1988). Stabilization of enzymes by multipoint covalent attachment to agarose-aldehyde gels: Borohydre reduction of trypsin-agarose derivatives. Enzyme Microb Technol. 11:360–366.
- Guisan, J.M., Bastida, A., Cuesta, C., Fernandez-Lafuante, R., Rosell, C.M. (1991). Immobilization-stabilization of chymotrypsin by covalent attachment to aldehyde agarose gels. Biotechnol Bioeng. 9:75–84.
- Mateo, C., Abian, O., Fernandez-Lafuante, R., Guisan, J.M. (2000). Increase in conformational stability of enzymes immobilized on epoxy-activated supports by favoring additional multipoint covalent attachment. Enzyme and Microbial Technology. 26:509–515.
- Alonso, N., Lopez-Gallego, F., Betancor, L., Hidalgo, A., Mateo, C., Guisan, J.M. Fernandez-Lafuente, R. (2005). Immobilization and stabilization of glutaryl acylase on aminated sepabeads supports by the glutaraldehyde crosslinking method. Journal of Moleculer Catalysis B: Enzymatic. 35:57–61.
- Agostinelli, E., Belli, F., Tempera, G., Mura, A., Floris, G., Toniolo, L., Vavasori, A., Fabris, S., Momo, F., Stevanato, R. (2007). Polyketone polymer: A new support for direct enzyme immobilization. J Biotechnol. 127: 670–678.
- Oliveira, P., Ramos, A.M., Fonseca, I., Botelho Do Rego, A., Vital, J. (2005). Oxidation of limonene over carbon anchored transition metal Schiff base complexes: Effect of the linking agent. Catal Today. 102–103:67–77.
- Krishnapillai, G.K., Konnully, S.J. (2007). Removal of Fe(III) using polystyrene anchored Schiff base. Environ Chem Lett. 5:19–21.
- Hernaiz, M.J., Crout, H.G. (2000). A highly selective synthesis of N-acetyllactosamine catalyzed by immobilised β-galactosidase from Bacillus circulans. J Mol Catal B: Enzym. 10:403–8.
- Sari, N. (2006). First example of Schiff bases containing poly(acryl amid) in the synthesis and characterization. J Macromol Sci Pure Appl Chem. 43:1609–1618.
- Lever, A.B.P. (1984). Inorganic Electronic Spectroscopy. New York: Elsevier Science Publishing Company Inc.
- Sarı, N., Özcan, Ö. (2009). Synthesis, characterization, and selectivity studies of poly(acrylamide) incorporating Schiff bases. Chinese Journal of Polymer Science. 27: 675–683.
- Ghamgui, H., Miled, N., Karra-Chaabouni, M., Gargouri, Y. (2007). Immobilization studies and biochemical properties of free and immobilized Rhizopus oryzae lipase onto CaCO3: A comparative study. Biochem Eng J. 37:34–41.
- Lopez-Gallego, F., Betancor, L., Hidalgo, Dellamora-Ortiz, G., Mateo, C., Fernandez-Lafuente, R., Guisan, M. (2007). Stabilization of different alcohol oxidases via immobilization and post immobilization technics. Enzyme Microb Tech. 40:278–284.
- Arslan, F., Tumturk, H., Caykara, T., Şen, M., Guven, O. (2000). The effect of gel composition on the adsorption of invertase on poly(acrylamide/maleic acid) hydrogels. Food Chem. 70:33–38.
- Okutucu, B., Celem, E. B., Önal, S. (2010). Immobilization of β-galactosidase on galactose-containing polymeric beads. Enzyme Microb Tech. 46:200–205.
- Thippeswamy, S., Mulimani, V.H. (2003). Enzymic degradation of raffinose family oligosaccharides in soymilk by immobilized β-galactosidase from Gibberella fujikuroi. Process Biochem. 38:635–40.
- Tumturk, H., Sahin, F., Demirel, G. (2007). A new method for immobilization of acetylcholinesterase. Bioproc Biosyst Eng. 30:141–145.
- Akkaya, B., Sahin, F., Demirel, G., Tumturk, H. (2009). Functional poymeric supports for immobilization of cholesterol oxidase. Biochem Eng J. 43:333–337.
- Anita, A., Sastry, C.A., Hashim, M.A. (1997). Immobilization of urease on vermiculite. Bioproc Biosyst Eng. 16:375–380.
- Tumturk, H., Karaca, N., Demirel, G., Şahin, F. (2007). Preparation and application of poly(N,N-dimethylacrylamide-co-acrylamide) and poly(N-isopropylacrylamide-co acrylamide)-Carrageenan hydrogels for immobilization of lipase. Int. J. Biol. Macromolecules. 40:281–285.
- Altinok, H., Aksoy, S., Tümtürk, H., Hasirci, N. (2006). Covalent immobilization of invertase on chemically activated poly(2_hydroxyethyl methacrylate) microbeads. Russian Chemical Bulletin, International Edition. 55: 1860–1864.