Abstract
Abstract: The objective of the present study is to evaluate the pharmacodynamic properties of polymerized porcine hemoglobin (pPolyHb) in an exchange transfusion model. Each of two groups of rats received a volume of pPolyHb or hetastarch that equalled 120–140% of estimated total blood volume (70 ml/kg) exchange transfusion. The results showed pPolyHb retained hemodynamic stability and exhibited superior volume expansion capability. Furthermore, pPolyHb effectively reverse anaerobic metabolism caused by a large amount of volume exchange. In comparison with hetastarch, pPolyHb increased blood oxygen content and tissue oxygenation. All these properties contribute to a higher effectiveness in sustaining the lives of rats in pPolyHb group.
INTRODUCTION
Hemoglobin based oxygen carriers (HBOCs), with their capacity of delivering oxygen, have been developed in the last several decades in an attempt to replace red blood cell (RBC) partially or produce resuscitative fluids that solve some problems caused by RBC, crystalloid or colloidal solutions[Citation1–3]. Many HBOCs have been brought into clinical trial due to their superiority to RBC and other resuscitative fluids, such as extended shelf life, no need to be typed and cross-matched, no risk of viral or bacterial infections, high oxygen carrying ability, and long retention in the circulation [Citation4,Citation5]. So far, the principle raw material for HBOCs’ manufacture comes from human and bovine hemoglobin [Citation6,Citation7]. However, due to the limited supply of human Hb and the possible threat of human blood transmitted diseases such as hepatitis and HIV and cross-species transmission of prion [Citation8], porcine Hb has been developed as a new source of HBOCs [Citation9]. Among different types of HBOCs, those based on the use of the glutaraldehyde polymerization method for hemoglobin and enzymes [Citation10] are the most promising products in commercial development and some of them have been tested clinically in patients [Citation11–13].
In this paper, we have developed a new product of glutaraldehyde polymerized porcine Hb (pPolyHb). The purpose of the study was to evaluate the pharmacodynamic properties of pPolyHb and determine whether pPolyHb administration during exchange transfusion in rat model would maintain hemodynamic stability and adequately deliver oxygen to tissue, thus providing for effective life-sustaining ability compared with transfusion with hetastarch.
MATERIALS
Reagents
6% Hetastarch 200–0.5 in sodium chloride solution (Fresenius Kabi), Pentobarbital Sodium (Sigma), Hepalean 1000U.S.P. units/ml (Organon), Sodium Chloride (Sigma), Potassium Chloride (Sigma), Calcium Chloride (Sigma), Sodium Phosphate Monobasic (Sigma), Disodium Hydrogen Phosphate (Sigma).
Animals
Male Sprague-Dawley rats (Xian Jiaotong University, China) weighing 240 ± 20g, were used in the study. The experiments described in this study were performed in adherence to National Institutes of Health guidelines on the use of experimental animals. Approval of the Animal Care Committee of Northwest University was obtained prior to initiating the experiments.
Test Solutions
pPolyHb (10.5 ± 0.5g/dl polymerized porcine hemoglobin, methemoglobin <5%, endotoxin <1.0EU/mL, osmolality 300–330 mOsm, pH 7.4 ± 0.05, average molecule of pPolyHb 600 ± 50 kD, 64kD tetramer <2%) was formulated in buffer consisting of Na+ 135–155mmol/L, K+ 3.0–5.0 mmol/L, Ca2+ 1–3 mmol/L, Cl− 140–160 mmol/L and stored at 4°C under nitrogen gas until use.
METHODS
Surgical Preparation
Rats were anesthetized with sodium pentobarbital (45mg/kg, intraperitoneally). The left jugular vein was cannulated (PE 50 tubing) for drug administration. The left femoral artery was cannulated (PE 50 tubing) and connected to a MP150 Data Acquisition System (BIOPAC, USA) for recording blood pressure, ECG, and heart rate. The right femoral artery was cannulated to induce controlled hemorrhage. The animals were allowed to stabilize for 60 min before starting the experiment. Blood gas analysis was performed on an ABL 800 FLEX (Radiometer, Copenhagen, Denmark).
Exchange Transfusion
Test solutions were filtered through a 0.22–μm filter immediately before infusion. Rats were heparinized before exchange transfusion through the venous catheter at 60 units/100 g body weight. The test solution was warmed to the body temperature of 37°C. Blood was removed from the femoral artery and exchange fluid (pPolyHb or hetastarch) was replaced simultaneously via the femoral vein [Citation14]. Exchange transfusions were done at a rate of 0.3 ml/min to a total volume of solution that equalled 120–140% of estimated total blood volume (70 ml/kg) and residual erythrocyte hemoglobin was less than 2g/dl.
Pharmacodynamics Monitoring
Mean arterial blood pressure (MAP), systolic blood pressure (SP), diastolic blood pressure (DP), heart rate (HR), and respiration rate were monitored every 5 min throughout the experiment. Blood samples were withdrawn before the start of blood exchange (baseline) and at different stages of exchange when erythrocyte hemoglobin was 10 ± 1 g/dl, 6 ± 1g/dl, 1.5 ± 0.5g/dl, to test PO2, PCO2, pH, SO2, CaO2, base excess, lactate, HCO3−, K+, Na+, Ca2+, and Cl−.
Statistical Analysis
Data was represented as means ± SD for replicate experiments. The differences between treatment groups were assessed by one-way ANOVA followed by unpaired Student's t-test. Statistical significance was defined as p < 0.05 to reject a null hypothesis. All statistical calculations were performed with JMP version 3.2 for the Macintosh (SAS Institute, Cary, NC).
RESULTS
Life Sustaining Ability
pPolyHb group showed a significantly higher effectiveness in sustaining the life of the pPolyHb exchange transfused animals (p < 0.05) than the hetastarch group (). With exchange transfusion down to erythrocyte hemoglobin level of 2g/dl, pPolyHb was effective in 100% of rats in the pPolyHb group for 13h, while the hetastarch group was only effective in 50% of the rats for up to 7h. Since the circulation half time of pPolyHb is 18h, beyond 13 hours pPolyHb was able to sustain the life of 40% as compared to 0% in the hetastarch group.
Figure 1. Life-sustaining ability of rats in exchange transfusion model. Rats received a total volume of pPolyHb or hetastarch (HES) that equalled 120–140% of estimated total blood volume (70 ml/kg) exchange transfusion and residual erythrocyte hemoglobin was less than 2g/dl. Life sustained ratio was calculated.
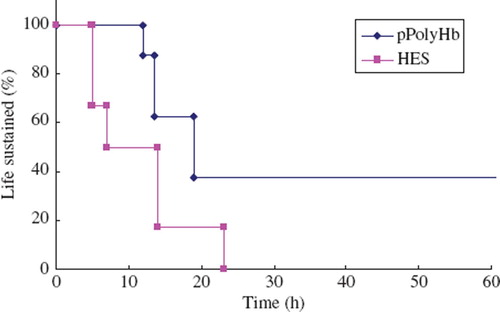
Blood Pressure and Heart Rate
Twelve SD male rats were entered into the study. Surgical preparation time averaged 60 min and was not different between groups. There was no significant difference in baseline physiological variables between two groups ().
Table 1. Baseline parameter comparison between groups.
Continuous mean arterial pressure (MAP), systolic pressure (SP), and diastolic pressure (DP) readings were obtained from exchange transfused rats. Six rats received pPolyHb, and six received hetastarch. The exchange transfusion lasted for 60–80 min. As shown in and , pPolyHb maintained a stable MAP, SP, and DP throughout the whole study period. In contrast, the animals treated with hetastarch displayed a significant decrease value of these hemodynamic parameters. Heart rate showed a positive correlation with MAP (, ). These results indicated the superiority of pPolyHb in retaining hemodynamic stability.
Figure 2. Blood pressure and heart rate in rat exchange transfusion model. Mean arterial blood pressure (MAP), systolic blood pressure (SP), diastolic blood pressure (DP), and heart rate (HR) were monitored every 5 min throughout the experiment. A: changes of MAP, SP, DP in pPolyHb group; B: changes of MAP, SP, DP in hetastarch group; C: changes of HR in pPolyHb group; D: changes of HR in hetastarch group.
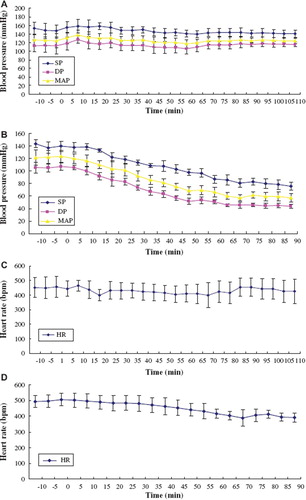
Respiration
As shown in and , animals transfused with pPolyHb could retain a stable respiration rate throughout exchange periods. However, respiration rate sped up following the continuation of exchange in hetastarch group, especially when erythrocyte hemoglobin was less than 2g/dl. This result suggested that the formation of anoxia in hetastarch group stimulated respiratory center of rats, leading to acceleration of respiration rate.
Blood Measurement
Two groups were similar at the baseline on arterial pH, PCO2, PO2 value (). These parameters varied slightly in pPolyHb group during the exchange transfusion process. In contrast, animals in hetastarch group have markedly decreased pH and PCO2 value as well as increased PO2 value when erythrocyte hemoglobin was below 2g/dl. The decrease in PCO2 is due to hyperventilation, while decrease in pH due to increase in lactate and decrease in HCO3− and base excess (BE) as a result of anoxia.
Table 2. Changes of pH, PaCO2, PaO2 in exchange model (n = 6, .x ± s).
Electrolyte analysis was also performed through an ABL 800 FLEX blood gas analyzer. As shown in , concentration of K+, Na+, Ca2+, Cl− had no significant change during the study periods in both groups.
Table 3. Changes of K+, Na+, Ca2+, Cl− concentration in exchange model (n = 6, .x ± s).
Metabolic Markers
Lactate, base excess (BE), and HCO3− are the markers of anaerobic metabolism. According to , there is no significant difference for lactate level in pPolyHb group from the start of blood exchange to the end of exchange. However, lactic acidosis developed in the hetastarch group when erythrocyte hemoglobin fell below 2g/dl. Base excess (BE) and HCO3− level (, ) mirrored lactate change during the process of exchange when transfused with pPolyHb, whereas BE and HCO3− levels in the hetastarch group were significantly lower than those in the pPolyHb group at the end of exchange (P < 0.05), which means continual administration of hetastarch is ineffective in restoring lactate, BE, and HCO3− level. This ultimately led to a severe metabolic acidosis with resulting severe base deficit and death of the animal. In contrast, pPolyHb could reverse anaerobic metabolism due to its effectiveness in oxygen delivery when necessary.
Figure 4. Lactate, base excess (BE), and HCO3− level in rat exchange transfusion model. Blood samples were withdrawn before the start of blood exchange (baseline) and at different stages of exchange when erythrocyte hemoglobin was 10 ± 1g/dl, 6 ± 1g/dl, 1.5 ± 0.5g/dl, to test lactate, base deficit, HCO3− level. Blood gas analysis was performed on an ABL 725 (Radiometer, Copenhagen, Denmark). *P <0.05 in comparison to hetastarch group; #P < 0.05 in comparison to baseline. A: Comparison of lactate level in different study periods of pPolyHb group with that of hetastarch groups; B: comparison of BE level in different study period of pPolyHb group with that of hetastarch groups; C: comparison of HCO3− level in different study period of pPolyHb group with that of hetastarch groups.
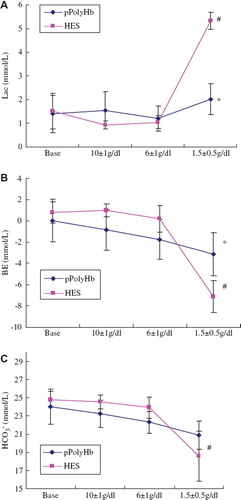
Oxygen Delivery and Extraction
In comparison with hetastarch, pPolyHb increased blood oxygen content and tissue oxygenation. showed the CaO2 level is higher in pPolyHb treated animals, suggesting that pPolyHb could transport oxygen more effectively. (SaO2-SvO2)/SaO2 ratio approximately indicates oxygen extraction of the tissue. indicated that (SaO2-SvO2)/SaO2 in pPolyHb group is lower than that in the hetastarch group when erythrocyte hemoglobin was under 2g/dl. This implied that oxygen delivery decreased dramatically in the hetastarch group as a result of erythrocyte hemoglobin lost and oxygen extraction increased correspondingly in order to maintain the normal metabolism. With respect to pPolyHb, since it was capable of transporting oxygen to tissues when necessary, (SaO2-SvO2)/SaO2 will decrease as a result of pPolyHb's efficient oxygen delivery.
Figure 5. (SaO2-SvO2)/SaO2 ratio in rat exchange transfusion model. Blood samples were withdrawn before the start of blood exchange (baseline) and at different stages of exchange when erythrocyte hemoglobin was 10 ± 1 g/dl, 6 ± 1g/dl, 1.5 ± 0.5g/dl, to test SaO2, SvO2. Blood gas analysis was performed on an ABL 725 (Radiometer, Copenhagen, Denmark). *P < 0.05 in comparison to hetastarch group; #P < 0.05 in comparison to baseline.
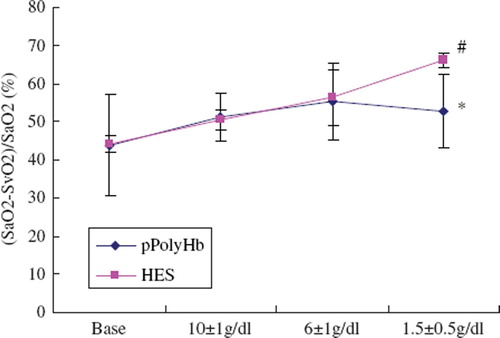
Table 4. Changes of CtO2 in exchange model (n = 6, .x ± s).
DISCUSSION
To evaluate the pharmacodynamics of pPolyHb, 120–140% exchange transfusion models of rats were used in our study. Life-sustaining ability in the current model is dependent on augmentation of oxygen-carrying capacity. This is demonstrated by the effectiveness in sustaining the life of the animals transfused with pPolyHb as compared to animals transfused with hetastarch. Hetastarch with little or no oxygen-carrying capabilities was not sufficient to sustain basic life. Animals in the hetastarch group couldn't turn over and move freely after the operation, and were not able to drink or eat by themselves. Their respirations were weak and even a simple action such as raising the head became a hard task for them. In contrast, animals transfused with pPolyHb restored their normal activities after the operation was finished. This suggested that pPolyHb contributed to oxygen carriage and that the delivery was adequate to sustain life.
MAP and HR are the indexes for demonstrating the function of cardiovascular system. MAP and HR of rats kept stable in the whole process of blood exchange when pPolyHb transfused, demonstrating that pPolyHb has excellent volume expansion capability and could maintain hemodynamic stability as well.
NO is continuously formed in the vascular endothelium and rapidly diffuses to adjacent smooth muscle beds where it mediates vascular relaxation by activation of the heme-dependent enzyme guanylate cyclase. The intercellular junctions of the endothelial lining of the vascular wall allow molecular dimension Hb to enter into the interstitial space binding and removing nitric oxide needed for maintaining the normal tone of smooth muscles [Citation15,Citation16]. This results in constriction of the blood vessels and other smooth muscles. Some hemoglobin-based oxygen carriers have been shown to produce dose-dependent vasoconstriction in isolated blood vessels [Citation17]. pPolyHb's large size may limit its passage into the extracellular subendothelial space where the major NO is released. Meanwhile, the minimal tetrameric hemoglobin content (less than 2%) of pPolyHb ensured it is free of inducing vasoconstriction.
Lactate, base excess (BE), and HCO3− are the markers of anaerobic metabolism. pPolyHb could effectively reverse anaerobic metabolism caused by a large amount of volume exchange and erythrocyte hemoglobin lost due to its superior oxygen-carrying and releasing capability, which help avoid deteriorating metabolic acidosis and sustain normal life. This was also supported by the comparison of different trends of pH and PCO2 change developed in two groups. pH and PCO2 value decreased markedly in hetastarch group whereas these parameters kept stable in pPolyHb group when erythrocyte hemoglobin was under 2g/dl.
CaO2 level and (SaO2-SvO2)/SaO2 ratio reflect oxygen delivery and oxygen extraction to some extent. The higher CaO2 level ensured the adequate oxygen supply, which in turn facilitated the improvement of anoxia state of tissue, therefore reducing the oxygen extraction in the pPolyHb group. Other parameters, such as CO and tPO2, which could directly reflect oxygen delivery and consumption, are still under investigation.
CONCLUSION
pPolyHb described in this study can adequately deliver oxygen to tissue and has excellent volume expansion capability, ensuring a higher effectiveness in sustaining the life of rats exchange transfused with 120–140% of estimated total blood volume. All the results indicate that pPolyHb is a potential new hemoglobin-based oxygen carrier for possible future clinical trial.
Declaration of interest: The authors report no conflicts of interest. The authors alone are responsible for the content and writing of the paper.
REFERENCES
- Bonegio, R.G., Fuhro, R., Ragno, G., Valeri, C., Lieberthal, W. (2006). A comparison of the acute hemodynamic and delayed effects of 50% exchange transfusion with two different cross-linked hemoglobin based oxygen carrying solutions and pentastarch. Artificial Cells, Blood Substitutes and Biotechnology 34: 145–157.
- Ernest, D., Belzberg, A.S., Dodek, P.M. (1999). Distribution of normal saline and 5% albumin infusions in septic patients. . Crit. Care Med. 27: 46–50.
- Roberts, J.S., Bratton, S.L. (1998). Colloid volume expanders. Problems, pitfalls and possibilities. Drugs 55: 621–630.
- Cohn, S.M. (1997). Is blood obsolete? J Trauma 42: 730–732.
- Nucci, M.L., Abuchowski, A. (1998). The search for blood substitutes. Scientic America 2: 72–77.
- Goodnough, L.H., Brecher, M.E., Kanter, M.H., Aubuchon, J.P. (1999). Transfusion medicine. First of two parts-blood transfusion. N Engl J Med. 340: 438–47.
- Stowell, C.P., Levin, J., Spiess, B.D., Winslow, R.M. (2001). Progress in the development of RBC substitutes. Transfusion 41: 287–99.
- Winslow, R.M. (2000).Alpha alpha-crosslinked hemoglobin: Was failure predicted by preclinical testing? Vox Sang. 79: 1–20.
- Zhu, X.L., Chu, W., Wang, T., Wang, F., Fan, D., Dan, N., Chen, C. (2007). Variations in dominant antigen determinants of glutaraldehyde polymerized human, bovine and porcine hemoglobin. Artificial Cells, Blood Substitutes and Biotechnology 35:518–532.
- Chang, T.M.S. (1971). Stabilization of enzyme by microencapsulation with a concentrated protein solution or by crosslinking with glutaraldehyde. Biochem. Biophys. Res. Com. 44:1531–1533.
- Gould, S.A., Moore, E.E., Hoyt, D.B., Ness, P.M., Norris, E.J., Carson, J.L., Hides, G.A., Freeman, I.H., DeWoskin, R. and Moss, G.S. (2002). The life-sustaining capacity of human polymerized Hb when red cells might be unavailable. J. Am. Coll. Surg. 195: 445–452.
- Jahr, J.S., Mackenzie, C., Pearce, L.B., Pitman, A., Greenburg, A.G. (2008). HBOC-201 as an alternative to blood transfusion: Efficacy and safety evaluation in a multicenter phase III trial in elective orthopaedic surgery. J Trauma 64: 1484–97.
- Pearce, L.B., Gawryl, M.S., Rentko, V.T., Moon-Massat, P.F., Rausch, C.W. (2006). HBOC-201 (Hb Glutamer-250 (Bovine), Hemopure): Clinical studies. Blood Substitutes, Winslow, R. San Diego: Academic Press, 437–4509.
- Chang, T.M.S. (2007). Artificial Cells: Biotechnology, Nanomedicine, Regenerative Medicine, Blood Substitutes, Bioencapsulation, and Cell/Stem Cell Therapy, Vol. 1 of Regenerative Medicine, Artificial Cells and Nanomedicine. Singapore: World Scientific.
- Chang, T.M.S. (1997). Blood Substitutes: Principles, Methods, Products and Clinical Trials, Vol. 1. Basel: Karger.
- Gould, S.A., . (1998). The clinical development of human polymerized hemoglobin, in blood substitutes. Principles, Methods, Products and Clinical Trials, Chang, T.M.S. Vol. 2. Basel: Karger 12–28.
- Hart, J., Ledvina, M., Muldoon, S. (1997). Actions of diaspirin crosslinked hemoglobin on isolated rat and dog vessels. J Lab. Clin. Med. 129: 356–363.