Abstract
Abstract: Herein, we describe a non-conventional method for immobilization of enzymes onto different solid surfaces using ultrasound as a source of energy. When horseradish peroxidase (HRP) was taken on the surface of an activated support and allowed to float on a sonicator bath operating at a frequency of 40 KHz, it readily started binding itself to the surface. Maximum binding was observed in 10 min whereas a control experiment carried out similarly without ultrasound waves showed insignificant immobilization. Ultrasound wave-mediated immobilization is rapid and reproducible and is better suitable for versatile applications in different fields, including fabrication of enzyme-based biosensors or bioreactors.
INTRODUCTION
Newly developed techniques like microarray, DNA-based biosensor, or high throughput drug screening in the emerging fields of genomics, proteomics, or glycomics require rapid, stable, and efficient immobilization of biomolecules. Several methods have been developed for immobilization of proteins, which are mainly based on the mechanisms of physical, covalent, and affinity interaction [Citation1].
Non-conventional energy, for instance microwave, laser ray, high pressure, and ultrasound, has become the subject of intense research in different fields of basic and applied science. Earlier we showed that different forms of energy can be utilized for rapid immobilization of enzymes onto solid supports such as heat [Citation2], microwave [Citation3], light [Citation4], and pressure [Citation5]. Herein, we tried to explore the applicability of ultrasound waves for rapid immobilization of enzymes onto different polymer supports. Ultrasonic waves have been used in several biotechnology applications [Citation6] such as enhancement of the reaction rate of agglutination test [Citation7,Citation8], plasma preparation from whole blood [Citation9] or proliferation of adherentcells in a microfluidic chip [Citation10]. In most of these applications, sound waves are involved to accelerate the physical processes whereas in the present method ultrasonic waves are used to accelerate the chemical reaction between the activated surface and the enzyme. Recently, we have demonstrated rapid ELISA where all the steps, including antigen immobilization, were carried out by ultrasound waves [Citation11]. Being a novel method, it becomes necessary to find out whether other biomolecules, such as enzymes, can also be immobilized by ultrasound waves. This study becomes more important because of the contradictory literature report about the stability of enzymes under the influence of ultrasound energy [Citation6,Citation12].
In this communication, we accelerate enzyme immobilization without altering activation procedure, support, or reagent. The method involves optimum use of ultrasonic waves, generated in a laboratory sonication bath, to effect rapid immobilization rather than using a thermal incubator. Rapid covalent immobilization has advantages as it reduces nonspecific adsorption and is better suited for versatile applications in different fields, including pharmaceuticals, food processing, diagnostics, fabrication of enzyme-based biosensors or bioreactors, and DNA-, protein-, or carbohydrate-based microarrays.
EXPERIMENTAL
Materials
Horseradish peroxidase (HRP), o-phenylenediamine dihydrochloride (OPD), and cellulose dialysis membranes were purchased from Sigma, USA. Polystyrene microtiter plates were purchased from Greiner, Germany. Polypropylene microcentrifuge tube was purchased from Axygen, India. All other chemicals were of analytical grade and were purchased either from Qualigens, India, or SRL, India. 1-Fluoro-2-nitro-4-azidobenzene (FNAB) was prepared as described previously [Citation13]. Phosphate buffer saline (PBS) was prepared by dissolving 0.85% (w/v) NaCl to phosphate buffer (0.01 M, pH 7.2) and washing buffer was prepared by adding 0.1% (v/v) Tween-20 to PBS. Sonication was carried out in a laboratory sonicator bath (Elma, Germany).
Activation of Solid Surfaces
Solid surfaces were activated either by the protocols as described earlier or by modifying them. For activation of polystyrene plates, methanolic solution of FNAB (10 μmol/50 μl/well) was poured to the wells and evaporated in dark. The photolinker coated dry wells were exposed to UV light of 365 nm in a UV Stratalinker (Model-2400; Stratagene, USA) for 12 min followed by washing twice with ethanol and once with methanol and then being dried [Citation14]. A polypropylene microcentrifuge tube of 0.5 ml capacity was activated by pouring 8 μmole of FNAB in 50 μl of carbon tetrachloride into it and exposing the tube to UV light for 20 minutes. After exposure, the tubes were washed with carbon tetrachloride followed by methanol and dried [Citation15]. Cellulose dialysis membrane and high density polyethylene sheet were cut into 1-cm2 discs and washed with methanol. Stock solution of FNAB (200 μmol/ml of methanol) was prepared and was slowly smeared (100 μl/disc) on both the sides of the cleaned disc and allowed to dry. Each side of the coated dry discs was irradiated at 365 nm for 8 minutes. After UV irradiation, the cellulose discs were washed with methanol and dried in air [Citation16].
Immobilization of Horseradish Peroxidase by Ultrasound
The activated and untreated wells of polystyrene microtiter plates were loaded with 100 μl of HRP solution (0.25 μg/well dissolved in 100 μl PBS). The plates were then floated on the sonicator bath and subjected to ultrasound waves for 5 to 25 min at an output ultrasound power of 110, 120, and 130 W, respectively. Temperature of the sonicator bath was maintained at 37°C by occasionally pouring ice in the bath. After sonication, the plates were washed four times with washing buffer to remove unbound enzyme and assayed colorimetrically.
Enzyme Assay
The immobilized HRP was assayed colorimetrically by loading 100 μl of substrate–dye buffer (5 mg OPD and 5 μl H2O2 dissolved in 12 ml of 0.2 M citrate buffer, pH 5.0). Color development was stopped after 5 min by adding 20 μl of 5 % H2SO4 and absorbance was recorded at 490 nm in an ELISA reader (Spectra Max, Molecular Devices, USA).
Comparison Between Sonication and Conventional Immobilization
HRP (0.25 μg/well/100 μl of phosphate buffer) was loaded into the activated and untreated wells of a polystyrene microtiter plate. The plate was then sonicated on the sonicator bath at an optimum power output of 120 W for 10 min at 37°C. In control experiments, HRP was immobilized in 10 min without sonication at 37°C. For comparison, HRP was immobilized conventionally for 4 h at 37°C in an incubator. The microtiter plates were washed and the immobilized HRP was assayed.
Immobilization of HRP by Sonication onto Different Matrices
Immobilization of HRP (0.25 μg/well) onto the wells of the polystyrene microtiter plate was carried out as described above. HRP (0.25 μg/tube) was immobilized into the polypropylene microcentrifuge tube by loading 100 μl HRP solution in it and sonicated for 10 min at an ultrasound output power of 120 W. Onto cellulose discs and polyethylene discs, HRP was immobilized by immersing the discs into microcentrifuge tube containing HRP solution (0.25 μg/100 μl PBS/disc) and sonicated for 10 min at an ultrasound output power of 120 W. After sonication, the surfaces were washed with washing buffer and the immobilized HRP was assayed as described above.
RESULTS AND DISCUSSION
Conventionally, immobilization of an enzyme onto a solid matrix is carried out in an incubator at a certain temperature. In this study, we have shown rapid immobilization of enzymes by ultrasound waves generated in a common laboratory sonicator bath. Thus, HRP was allowed to immobilize under the influence of ultrasound waves at different output power (110 W, 120 W and 130 W) at a fixed temperature of 37°C in sonicator bath. Simultaneously, for each output power, time-dependent immobilization was also studied and results showed that HRP immobilization was occurred at all of the three output powers by sonication. At 110 W, maximum absorbance was observed in 15 min whereas at output power of 120 W and 130 W, 10 min was found to give maximum absorbance. Hence, ultrasound waves of 120 W were taken as optimum output power for immobilization of enzymes onto solid supports. Significant immobilization was observed even in 5 min at a power output of 120 W and or 130 W. However, in both cases the absorbance started decreasing after 10 min that is beyond optimum conditions, which may be due to the deactivation of enzyme at higher ultrasound energy. In all these cases untreated surfaces showed insignificant immobilization, which indicates that the activated surface is a prerequisite for rapid immobilization of enzymes by sonication. In other words, sonication facilitates immobilization through covalent bond formation rather than adsorption (). The labile fluoro group of the activated surface is easily displaced by the incoming amino group of an enzyme forming a stable covalent linkage. The acceleration of immobilization by ultrasound waves may be due to the increase in collision frequency between the reactants, that is the amino group of the enzyme and fluoro group of the activated surface.
Figure 1. Immobilization of horseradish peroxidase by sonication at output power of 110 W, 120 W, and 130 W in 5, 10, 15, 20, and 25 min, respectively, onto activated (closed symbols) and untreated (open symbols) wells of polystyrene microtiter plates. Absorbance was recorded after assaying immobilized enzyme colorimetrically.
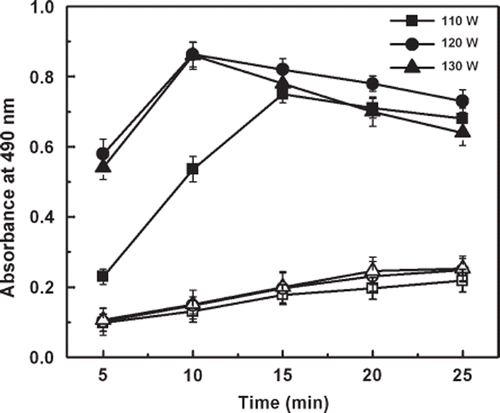
Earlier we showed that the photoactivated surface gave maximum immobilization of protein molecules in 3 to 4 h by conventional thermal incubation at 37°C [Citation2]. Therefore, we have compared sonication-mediated immobilization with the conventional method of immobilization, which was carried out at 37°C in 4 h. The absorbance value obtained by the conventional method was akin to that of the sonication method. To authenticate that the present method indeed describes a rapid method, we have carried out an experiment where immobilization was performed in absence of ultrasound waves in 10 min. As expected, very low absorbance (around 5-fold less than the ultrasound method) was obtained in the experiment carried out without sonication, which confirmed that the ultrasound wave indeed was responsible for rapid immobilization of enzyme to the solid matrix ().
Figure 2. Immobilization of HRP onto the activated (black bar) and untreated (white bar) wells of polystyrene microtiter plates by: (i) sonication at 120 W in 10 min at 37°C; (ii) control experiment carried out at 37°C in 10 min without sonication; and (iii) conventional experiment at 37°C for 4 h without sonication.
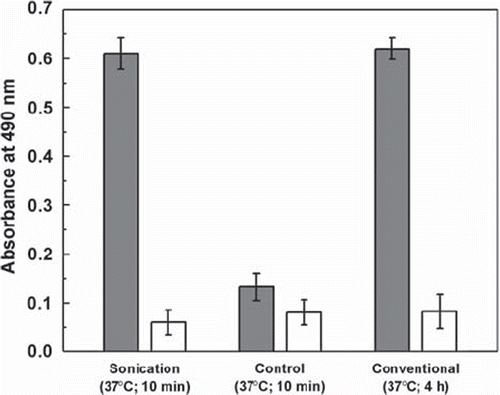
In order to explore the immobilization of enzymes onto different solid matrices by ultrasound waves, we have carried out immobilization of HRP (0.25 μg/matrix) onto the activated surface of polystyrene microtiter plates, polypropylene centrifuge tubes, polyethylene discs, and cellulose discs by sonicating at an ultrasound output power of 120 W for 10 minutes. The results showed significant immobilization of enzymes by ultrasound waves irrespective of solid supports. In all these cases activated surfaces give more than 5- to 7-fold higher absorbance values by 10 min of sonication than without sonication (), reaffirming the fact that ultrasound is indeed responsible for rapid covalent immobilization of enzymes onto the activated matrices.
CONCLUSIONS
This method could be used for rapid immobilization of enzymes without loosing its activity to any surface of any shapes. Sonication-mediated immobilization is best achieved when the solid surface has an active functional group for covalent bonding. The method is handy because, instead of an incubator shaker, any common laboratory sonicator bath can be used for this purpose. The advantages of ultrasound wave-mediated immobilization include: (i) it is fast; (ii) less non-specific immobilization; (iii) easily accessible sonicator bath; (iv) enhanced thermal heating not required; and (v) potentially useful for preparation of protein, DNA, or carbohydrate microchips as minimum evaporation of solvent or buffer during immobilization in contrast to a common incubator shaker.
Declaration of interest: The authors report no conflicts of interest. The authors alone are responsible for the content and writing of the paper.
REFERENCES
- Rusmini, F., Zhong, Z., Feijen, J. (2007). Protein immobilization strategies for protein biochips. Biomacromolecules, 8: 1775–1789.
- Bora, U., Chugh, L., Nahar, P. (2002). Covalent immobilization of proteins onto photoactivated polystyrene microtiter plates for enzyme-linked immunosorbent assay procedures. J Immunol Methods, 268: 171–177.
- Nahar, P., Bora, U. (2004). Microwave-mediated rapid immobilization of enzymes onto an activated surface through covalent binding. Anal Biochem, 328: 81–83.
- Kumar, S., Nahar, P. (2007). Sunlight-induced covalent immobilization of proteins. Talanta, 71: 1438–1440.
- Kannoujia, D.K., Ali, S., Nahar, P. (2009). Pressure-induced covalent immobilization of enzymes onto solid surface. Biochem Eng J, 48: 136–140.
- Rokhina, E.V., Lens, P., Virkutyte, J. (2009). Low-frequency ultrasound in biotechnology: State of the art. Trends Biotechnol, 27: 298–306.
- Thomas, N.E., Sobanski, M.A., Coakley, W.T. (1999). Ultrasonic enhancement of coated particle agglutination immunoassay: Influence of particle density and compressibility. Ultrasound Med Biol, 25: 443–450.
- Doubrovski, A.D., Dvoretski, K.N. (2000). Ultrasonic wave action upon the red blood cell agglutination in vitro. Ultrasound Med Biol, 26: 655–659.
- Cousins, C.M., Holownia, P., Hawkes, J.J., Limaye, M.S., Price, C.P., Keay, P.J., Coakley, W.T. (2000). Plasma preparation from whole blood using ultrasound. Ultrasound Med Biol, 26: 881–888.
- Hultström, J., Manneberg, O., Dopf, K., Hertz, H.M., Brismar, H., Wiklund, M. (2007). Proliferation and viability of adherent cells manipulated by standing-wave ultrasound in a microfluidic chip. Ultrasound Med Biol, 33: 145–151.
- Sharma, P., Nahar, P. (2009). Ultrasound wave-mediated enzyme-linked immunosorbent assay technique. Anal Chim Acta, 650: 241–246.
- Vercet, A., Burgos, J. Crelier, S., Lopez-Buesa, P. (2001). Inactivation of proteases and lipases by ultrasound. Innov. Food Sci Emerg Techno, 2: 139–150.
- Naqvi, A., Nahar, P. (2004). Photochemical immobilization of proteins on microwave-synthesized photoreactive polymers. Anal Biochem, 327: 68–73.
- Nahar, P., Wali, N.M., Gandhi, R.P. (2001). Light-induced activation of an inert surface for covalent immobilization of a protein ligand. Anal Biochem, 294: 148–153.
- Naqvi, A., Gandhi, R.P., Nahar, P. (2002). Introduction of functional groups onto polypropylene and polyethylene surfaces for immobilization of enzymes. Anal Biochem, 306: 74–78.
- Bora, U., Kannan, K., Nahar, P. (2005). A simple method for activation of cellulose membrane for covalent immobilization of biomolecules. J Memb Sci, 250: 215–222.