Abstract
Abstract: PEG-Alb represents a new class of low viscogenic plasma expanders that achieve super perfusion in vivo by mimicking the vasodilatory influence of high viscogenic plasma expanders. PEGylation-engineered structure of PEG albumin can be envisaged as a deformable molecular domain around the rigid central protein core. The correlation between the structure of PEG-shell in terms of packing of the PEG inside the PEG shell and PEGylation induced plasma expander (PE)-like properties of albumin has been investigated as a function of the number and length of the PEG-chain. The increase in molecular radius of albumin on PEGylation is non-linear as a function of the number of PEG chains conjugated. The packing density of PEG within the PEG-shell is an inverse correlate of PEG-chain size; i.e. the shorter chains pack more compactly than the longer ones. The PEGylation induced increase in the viscosity and COP of albumin is an exponential correlation of the number of ethylene oxide units (-CH2-CH2-O-) conjugated and is also a function of the PEG-chain length. At equivalence of PEG mass conjugated, the viscosity and COP of PEG-albumin adducts correlate inversely with packing density of PEG. All PEGylated albumins are not equivalent on the basis of total PEG mass conjugated. Accordingly, the structure of PEG albumin and its solution properties can be engineered to optimize a given total PEG mass for the application of PEG albumin as a resuscitation fluid. The extension arms minimize the influence of PEG shell on the structure of the protein core. We speculate that EAF-PEGylation is a preferable platform for PEGylation of protein therapeutics and is expected to generate products with better therapeutic efficacy.
ABBREVIATIONS | ||
COP | = | colloidal osmotic pressure |
HSA | = | human serum albumin |
2-IT | = | 2-iminothiolane-HCl (Traut's reagent) |
4-PDS | = | 4,4-dithiodipyridine |
PEG | = | poly ethylene glycol |
RI | = | refractive index |
SEC | = | size exclusion chromatography |
SP | = | succinimidophenyl |
EAF | = | extension arm facilitated |
PE | = | plasma expander |
DSC | = | differential scanning calorimetry |
TCP- | = | thiocarbamoyl phenyl |
INTRODUCTION
PEGylation of therapeutic proteins has been pursued for quite some time with a remarkable success as a chemical approach to increase the in vivo stability, and to lower the antigenicity of these molecules. Our recent studies have identified a new aspect of this technology, namely PEGylation induced increase in the viscosity and colloidal osmotic pressure [plasma expander (PE)-like properties] of albumin and of Hb makes these excellent plasma expanders. Hexa-PEGylated Hb and Albumin (using PEG 5K) appear to represent a new class of plasma expanders, supra resuscitation fluids. These lower viscosity PEGylated Hb and PEGylated albumin exhibit vasodilatation that is generally observed with the use of high viscosity plasma expanders. The correlation of the structure of the PEG-shell of either albumin or of Hb with the PEGylation-induced super perfusion properties has not been addressed so far. It is generally assumed that the beneficial effects of PEGylation is a correlate of the total PEG mass conjugated and is independent of chemistry and/or pattern of PEGylation. In the present study, we attempt to investigate this and this information is needed to attempt any optimization of PEGylation on Hb and albumin in their application as supra perfusion agents [Citation1–10].
The structure of PEG albumin and of PEGylated proteins in general, particularly of the ones with multiple copies of PEG chains, can be viewed to represent a novel class of semi-synthetic hybrid biopolymers with two distinct regions of packing density of the molecule. These have a central protein core of high packing density that is surrounded by a PEG-shell of low packing density and this structural design is very distinct as compared to natural biopolymers like starch and dextran that are used as colloidal plasma expanders. Understanding the structure of the PEG-shell at the molecular level, especially packing of the molecule in the PEG shell, is influenced by the chemistry, pattern of PEGylation, the PEG chain length, and the cross-correlation with the PEGylation induced plasma expander-like properties is essential information to improve the design of PEG-Hbs and of PEG albumins as new supra plasma expanders.
The PEGylation induced plasma expander-like properties of hexaPEGylated PEG-Hb (using PEG 5K) was originally hypothesized as facilitating the molecule to overcome in vivo the intrinsic vasoconstrictive activity of acellular Hb [Citation8] through improvements in functional capillary density, with little or no effect on the in vitro nitric oxide scavenging activity of Hb. Accordingly, the PEGylated Hb by themselves are a new class of blood substitutes distinct from the previously studied derivatives of Hb generated using small molecular weight protein modification reagents; i.e, these derivatives of Hb are oxygen-carrying plasma expanders. The PEG-albumin conjugates are the corresponding non-oxygen carrying plasma expanders, with a comparable size of the central protein core, and are without the complications of heme-mediated toxicity. Accordingly, we used a simple molecule to investigate supra perfusion independent of oxygen delivery. The supra perfusion afforded by PEG albumin is apparently a synergistic that lowers the transfusion trigger. Maximizing “active plasma expansion” properties of the PEG-shell by a given mass of PEG-conjugated to albumin and minimizing the PEGylation induced perturbation on the structure of central protein core will further increase the efficacy of PEGylated albumin and Hb as novel resuscitation fluids.
The well-recognized PEGylation mediated improvements in the properties of therapeutic proteins are increased in vivo half-life leading to improved efficacy, which is apparently a result of lowered susceptibility of the molecule to proteolysis and their propensity to camouflage the immune system. These general properties of PEGylated proteins will certainly play their role in the respective therapeutic applications of PEG-Hb and PEG-albumin as well, but the supra properties of these are expected to be the primary determinants for their clinical applications. We envisage that PEG-albumin is likely to serve as the volume expander (resuscitation fluid) when only volume replacement component of blood loss is required and the oxygen delivery can be taken care of by the remaining red blood cells in the circulatory system.
Correlation between chemistry of PEGylation, molecular size as well as the number of PEG-chains with the PEGylation induced PE-like properties of either PEG-Hb or of PEG-albumin has not been attempted so far. In the present study we have chosen human serum albumin, a single chain protein with a molecular weight comparable to that of Hb, to correlate PEGylation induced PE-like properties as a function of pattern of PEGylation and to map the influence of chemistry of PEGylation on the structure of the protein core.
Extension Arm Facilitated (EAF) PEGylation [Citation3] introduced to simplify the PEGylation of Hb and albumin has been chosen here as the PEGylation platform to delineate the correlation of PEGylation induced PE-like properties of albumin and pattern and chemistry of PEGylation. The compactness in the packing of the PEG-chains within the PEG shell of PEG-albumin adducts has been investigated as a function of number of PEG-chains using PEG chains of two different molecular sizes. PEGylation induced increase in viscosity and COP of the PEG-albumin adducts are correlated with the packing density of PEG in the PEG shell. The studies have established that shorter chains pack more compactly on the molecular surface of albumin than the longer chains, and also lower the increase in the viscosity and COP induced by PEGylation when compared with equal mass of PEG conjugated to albumin. The extension arms sandwiched between the PEG-shell and protein core attenuate the PEG-shell induced perturbation of the structure/conformation of the protein molecule. It is speculated that engineering extension arms in designing new PEG-protein hybrid biopolymers as PEGylated protein therapeutics could enhance the therapeutic efficacy of protein therapeutics in general as compared to the products generated using direct PEGylation platforms.
MATERIALS AND METHODS
Chemicals
Human Serum Albumin (HSA) essentially fatty acids free, ∼99 % (lot # A3782) lyophilized powder and 4′4′-dithiodipyridine (4-PDS; Aldrithiol-4) are from Sigma-Aldrich, Inc., MO, USA. 2-IT (Lot # 10222) and maleimidophenyl PEG5K (lot # 01186) were custom synthesized for us by BioAffinity, Inc., Rockford, IL. Ingredients for PBS and other analytical chemicals are of HPLC grade from Sigma-Aldrich or Fisher Scientific, USA.
Thiolation of HSA and Estimation of Thiolation
HSA was reacted with 5 mM 2-imunothiolane (2-IT) at different protein concentrations at 4°C for different time intervals (0.25, 0.5, 0.75, 1, 1.5, 2, 3, 4, 6, 8 hrs and overnight). In control experiments without PEG maleimide, the thiols introduced by the reaction of 2-IT on HSA are estimated by a method explained by Grassetti and Murray [Citation11].
Kinetics of thiolation of HSA at a concentration of 0.5 mM with 10-fold molar excess of 2-IT have shown that a product with an average of 6 thiol groups on its surface is generated and the same reaction condition has been chosen for the preparation of hexaPEGylated albumin using maleimide PEG 5K and PEG 3K.
PEGylation of HSA
PEGylation of HSA is carried out by a thiolation-mediated maleimide chemistry-based protocol () as detailed in Manjula et al. [Citation3] and carried out at different protein concentration as described earlier [Citation14]. Reaction mixture is dialyzed through 50K molecular weight cut-off membranes against PBS (pH 7.4) to remove excess IT and MPPEG5K using a Minim™ Tangential Flow Filtration instrument (Pall Corporation, Ann Arbor, MI, USA). Finally, the PEGylated sample is concentrated and collected in the tubes. Concentration of PEGylated HSA was determined using a Bradford protein assay kit (Pierce Biotechnology, Inc., Rockford, IL) and stored at −80 °C.
Figure 1. A: Schematic representation of EAF-PEGylation. B: Stability of thiolated albumin against scrambling of the internal disulfide bonds of albumin by thiol disulfide exchange reaction and against intermolecular cross-linking reaction to form oligomers through oxidation of thiols to generate inter-molecular disulfide bonds. A, HSA without IT; b, HSA (0.5 mm) with 5 mM IT incubated overnight (about 6 thiols in the final product); c, HSA (0.5 mM) with 10 mM IT incubated overnight at 4°C (about 12 thiol groups per mole) were subjected to SEC analysis using FPLC equipped with two analytical Superose 12 columns connected in series. C: PEGylation of HSA with 10 mM maleimidophenyl PEG3K in the presence of 5 mM 2-IT as a function of protein concentration ranging from 0.0625 to 1 mM as reflected by SDS-PAGE. D: Extent of PEGylation of HSA with 10 mM maleimidophenyl PEG5K in the presence of 5 mM 2-IT carried out in PBS pH 7.4 overnight at 4°C as a function of protein concentration ranging from 0.0625 (∼ 4 mg/ml) to 1 mM (∼ 66 mg/ml) as reflected by SDS-PAGE (Lane 1; marker, lane 2; control HSA, lane 3; 1 mM, lane 4; 0.5 mM, lane 5; 0.25 mM, lane 6; 0.125 mM and lane 7; 0.0625 mM HSA).
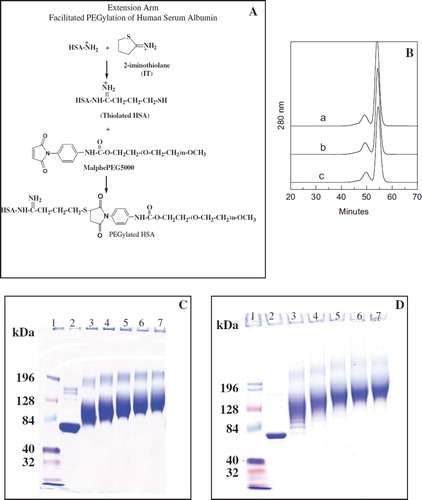
SEC of PEGylated samples
Size exclusion chromatographic analysis of PEGylated HSA is carried out on two Superose-12 columns (300 × 10 mm) connected in series using PBS pH 7.4 at a flow rate of 0.5 ml/min and effluents monitored at 280 nm [Citation14].
SDS-PAGE
SDS-PAGE analysis was carried out using a precast 4-12% tris-glycine gel from Invitrogen Corporation as described previously [Citation12].
Fluorescence Measurements and Circular Dichroism Spectroscopic Analysis of PEGylated HSA
Steady-state fluorescence measurements and circular dichroism spectral analysis of the samples were carried out as discussed previously [Citation14].
Molecular Radius, Colloidal Osmotic Pressure, and Viscosity
The molecular radius COP and viscosity of HSA and PEGylated samples were determined as described previously [Citation14].
Differential Scanning Microcalorimetry
Thermal transitions of the HSA and PEGylated HSA are measured using microcalorimeter (VP-DSC, Microcall, LLC, Northampton, MA). Both control HSA and PEGylated HSA samples of 2 mg/ml are dialyzed overnight with PBS before loading to VP-DSC. Dialysis buffer is used as reference data to subtract from the sample data before normalizing the result by concentration. All samples are scanned at a temperature range of 20-100°C at a scan rate of 90°C per hour and Origin® scientific plotting software is used for the analysis of all DSC data.
RESULTS
Correlation of PEGylation Induced PE-like Properties with the Structure (PEG Packing) of PEG-Shell
Thiolation of HSA. The extent of PEGylation during Extension Arm Facilitated PEGylation (EAFP) is essentially dictated by the thiolation reaction, i.e. extent of thiolation, as long as the concentration of maleimide PEG is not limiting. Accordingly, by manipulating the reaction conditions for introducing increasing number of the extension arms with the thiol group [δ-mercapto butirimidyl chains on the amino groups of protein using 2-IT], we can essentially control the extent of the PEGylation reaction. The kinetics of thiolation of albumin in the presence of 5 mM 2-IT has been studied as a function of protein concentration. By lowering the protein concentration from 1 mM to 0.0625 mM and keeping the 2-IT concentration at 5 mM, the molar excess of the thiolating reagent, 2-IT, over the protein is increased from 5-fold to 80-fold. The concentration of albumin studied here for thiolation (and for PEGylation) varied by 16-fold (from 1 mM down to 0.0625 mM) in the presence of 5 mM 2-IT. At all protein concentrations, the kinetics was essentially biphasic, a fast initial phase and a slow second phase. At the highest protein concentration studied, namely at 1 mM (66.4 mg/ml), there is not much difference in the extent of thiolation of albumin in the overnight vs. eight hrs of reaction. On the other hand, as the protein concentration studied is lowered in the reaction mixture, the difference in the number of thiol groups introduced onto albumin in the overnight reaction vs the 8 hrs of reaction increased significantly. At the lowest protein concentration studied, nearly eight thiol groups are introduced in 8 hrs of reaction and they increased to about 20 groups in an overnight reaction. The level of thiolation of albumin increased by only about six-fold in these experiments, even though the increase in the molar ratio of thiolating reagent (2-IT) over albumin in the thiolation reaction mixture is increased by 16-fold. This is a clear reflection of the differential reactivity of the amino groups of albumin for thiolation, and only a subset of amino groups albumin are accessible for thiolation under a given set of thiolating conditions.
The Stability of the Thiolated Protein. EAF-PEGylation was first used to PEGylate Hb [Citation3, Citation6]. Hb does not have any disulfide bonds and hence there was no concern about the possibility that the new thiol groups introduced on Hb could participate in a thiol-disulfide exchange reaction with the native disulfide bond of the protein. The tertiary structure of albumin is conserved by a set of 17 native disulfide bonds. The native sets of disulfide bonds of albumin may be susceptible to thiol-disulfide exchange reaction when the albumin is thiolated. The loss of the native pattern of disulfide bonds of proteins, either through disulfide reshuffling or reduction, is expected to result in a significant increase in the hydrodynamic volume of the protein as a result of the loose packing of the polypeptide chain [relative to native protein, Acharya and Taniuchi, [Citation13]. This is seen by the SEC patterns of the proteins. In , the SEC pattern of two samples of thiolated albumin (0.5 mM albumin in PBS at 4°C, in the presence of 5 and 10 mM 2-IT) is shown. These samples carry approximately six and 12 thiol groups after the overnight reaction. The pattern of control HSA is also shown for comparison. The two patterns of thiolated HSA are indistinguishable from that of the native protein. It is clear that the hydrodynamic volume of HSA has not changed during the thiolation. Accordingly, it is inferred that scrambling of the internal disulfide bonds of albumin did not occur as a result of either intramolecular thiol-disulfide exchange reactions or by intermolecular disulfide bond formation. It may be noted that thiols of partially reduced albumin generated by incubation of albumin with DTT are susceptible to intermolecular disulfide bond formation to generate molecular species of higher hydrodynamic volume (data not shown), thus absence of higher hydrodynamic volume species in the overnight thiolated albumin is not a consequence of the low resolution of the column. This reflects the stability of the thiolated HSA, and accordingly the thiolation mediated PEGylation (extension arm facilitated) of HSA can be undertaken without any major concern. It is also clear that oligomerization of thiolated albumin also did not occur as a result of the formation of intermolecular disulfide bonds through air oxidation, even though the thiolation was done under aerobic conditions.
EAF-PEGylation of Albumin as a Function of Protein Concentration. Since the above conditions, wherein the molar excess of the thiolating agent (2-IT) over the albumin is varied by 16-fold, presents an excellent range of thiolation of albumin; albumin has been subjected to PEGylation under these conditions in the presence of either 10 mM MPPEG3K or MPPEG5K (two-fold molar excess over the thiolating agent) for overnight reaction in 4°C. The numbers of PEG-chain introduced onto albumin in the absence and in the presence of maleimide PEG. The calculated difference in the number of thiols per mole of thiols between the two samples represents the number of PEG chains conjugated to albumin. We have previously shown that the number of PEG chains in PEG-BSA calculated by this approach correlated well with the level of PEGylation calculated by NMR approach [Citation14]. Accordingly, the number of PEG-chains conjugated to each sample is considered to be the same as the difference in the number of thiols per mole of albumin between that of thiolation control and the PEGylation reaction, and this number is presented in .
Table 1. PEGylation induced increase in the molecular volume of HSA as a function of number of chains conjugated using EAF-PEGylation with maleimido phenyl PEG (5K and 3K) as the PEGylating reagenta
SEC analysis of all PEGylated samples has been carried out for the distribution of PEGylated species as the hydrodynamic volume of albumin increases as a function of protein concentrations. The SEC patterns indicate a continuous increase in the hydrodynamic volume as a function of proteins; these patterns suggest a broad distribution of products with a very wide distribution of the PEGylated products with different number of PEG-chains that slowly become a narrow distribution of PEGgylated products with a more uniform distribution of the number of PEG-chains per molecule. This apparent molecular size homogeneity is seen at almost every protein concentration studied, and is apparently a consequence of the significant differences in the reactivity of the amino groups of albumin for thiolation and the very high reactivity, and the selectivity of the thiols on the extension arm with maleimide PEG. This suggests that, at given concentrations of protein and 2-IT, PEGylation is limited only to a set of amino groups that react with 2-IT and these thiols are PEGylated almost quantitatively.
SDS-PAGE Pattern of PEGylated Albumin as a Function of Protein Concentration. The SDS-PAGE of the PEGylated albumins generated using both maleimide PEG3K and maleimide PEG5K is shown in and 1D, respectively. As the protein concentration is lowered in the reaction mixture, the apparent molecular size of the PEGylated samples increases in both series of PEGylated samples. The SDS-PAGE bands of all PEGylated proteins generated at different concentrations remained reasonably compact, and this reflects the limited distribution of the molecular size of the PEGylated albumin at a given protein concentration. The apparent molecular weight of albumin with an average 3.5 copies of PEG5K chains is around 128 K while the molecule with nearly 19 copies of PEG5K chains is close to 196 K. Similarly, the molecular mass of albumin with about 4 copies of PEG3K chains are around 84 K while the one with about 19 copies of PEG3K chains is around 128 K. The difference between the apparent molecular weight on the SDS-PAGE and the actual molecular size of PEG albumin is reduced as the extent of PEGylation on the protein increases. The apparent molecular mass of the PEGylated albumin in SDS-PAGE, for the sample generated at a protein concentration of 0.0625 mM, is comparable to the calculated molecular mass for the adduct with nearly 20 copies of PEG chains.
Molecular Radius of PEG-albumin Conjugates as a Function of PEGylation. The molecular radius of PEG albumin conjugates has been determined by dynamic light scattering as function of number of PEG chains conjugated (). The molecular radius of the PEGylated albumin generated using PEG3K is smaller as compared to the product generated using PEG5K with the same number of PEG-chains. Besides, the increase in the molecular radius of albumin as a function of the PEG chains is a biphasic phenomenon, an initial phase wherein the rate of increase in the molecular radius as the function of PEG-chain increases, is relatively large (until about six to eight PEG-chains are introduced), and a second phase wherein the rate of increase in the molecular radius of PEGylated albumin is small. We have seen this biphasic correlation pattern earlier with bovine serum albumin and Hb [Citation14]. The present study shows that the molecular size of the PEG-chain seems to have little influence on this biphasic nature of the increase in molecular radius of PEG-albumin adducts, and the transition appears to be independent of the PEG-chain size, i.e. occurs at the same level (number of PEG-chains) of PEGylation.
Figure 2. Packing density of PEG within the PEG-shell as a function of number of PEG-chains conjugated to albumin. A: Increase in the molecular radius of albumin as a function of PEGylation, i.e., number of PEG chains conjugated to albumin. B: Packing density of PEG in the PEG-shell of EAF PEG albumin as a function of number of PEG chains conjugated; note that PEG-3K chains pack much more compactly than PEG5K chains. C: Packing density of PEG in the PEG shell of EAF PEG albumin as a function of total PEG mass conjugated to the protein.
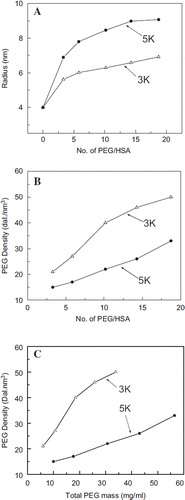
Influence of Molecular Size of PEG-chain on the Packing Density of PEG within the PEG-shell as a Function of Number of PEG-chains. The nonlinear increase in the molecular radius of the PEG-albumin suggests the tighter packing of the PEG-chains within the PEG-shell as a function of PEGylation. The packing density of PEG within the new PEG shell generated around albumin is calculated as a function of number of the PEG-chains that constitute the PEG-shell (). The correlation of packing density of PEG chains in the PEG-shell as a function of PEGylation is presented in . The correlation pattern of PEG-density as a function of PEGylation is very distinct between PEG3K and PEG5K, i.e. the PEG-chain length plays a major role in dictating the packing density within the PEG-shell. At all levels of PEGylation, the PEG packing-density is higher in the samples PEGylated with PEG3K as compared with that of PEG5K. PEG chains of smaller PEG-shell pack more tightly between themselves and also with the protein surface. Thus PEG3K appears to establish more PEG-protein interactions and PEG-PEG interactions as compared to PEG-5K. The packing density increases almost linearly with PEG5K in the whole range studied, but in the case of PEG3K it appears to be linear until about 10 copies of chains; after that the increase becomes smaller.
Increase in Viscosity and COP of Albumin as a Function of PEGylation. The changes in the viscosity and COP of albumin as a function of number of PEG-chains (both PEG 5K and PEG 3K), respectively, are presented in and . Viscosity and COP of PEGylated albumin at 4 gm % increase exponentially as a function of increase in the number of PEG-chains conjugated with PEG5K. The increase in viscosity as well as COP seen with PEG3K as a function of PEGylation is more linear than exponential, besides being smaller than with PEG-5K. Accordingly, the difference in the COP between the samples PEGylated with PEG5K and PEG3K is significantly increased as the number of PEG-chains conjugated to albumin increases.
Figure 3. Correlation of the PEGylation induced solution properties (viscosity and Colloidal oncotic pressure) of PEG-albumin adducts as a function of PEGylation. PEG-chains of two different molecular sizes have been used for PEGylation. Viscosity (A) and COP (B) of PEG-albumin were measured at 4 gm % (protein). Inset shows correlation of viscosity (inset in A) and COP (inset in B) as a function of oxy ethylene units to define whether solution properties are just a function of PEG mass.
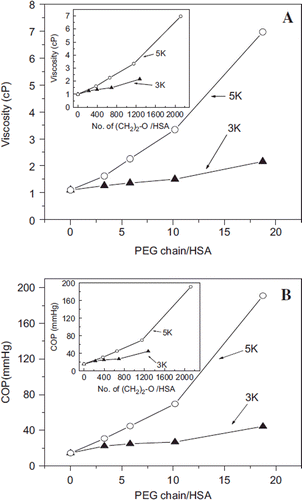
The viscosity and COP the PEGylated albumin are presented in the insets of and , respectively, as a function of number of oxy-ethylene units conjugated per albumin molecule. The molecular size of the PEG-chain when normalized as number of oxy-ethylene units, the overall efficacy of PEG3K and PEG5K chains to increase viscosity and COP of albumin follows very different and distinct patterns (inset, ). The oxy-ethylene units, the building blocks of this synthetic polymer PEG, are more efficient to increase the COP and viscosity (inset ) as a part of longer PEG-chains.
Influence of the PEG-Shell on the Overall Conformation of Protein Core as Function of Number of PEG-chains. EAF-PEGylation of albumin with PEG5K results in a quenching of the fluorescence, and this quenching is not accompanied by any significant change in the emission maximum of the fluorescence emission spectra (). As the level of PEGylation is increased, the fluorescence quenching is also increased. The inset in shows that the EAF-PEGylation induced quenching of Trp fluorescence of albumin is a direct correlate of the extent of PEGylation.
Figure 4. Influence of conjugated and free PEG5K chains on the Trp fluorescence of albumin. A: Fluorescence spectra of EAF PEGylated albumin. Curves a to d represent the fluorescence of HSA conjugated with 0, 3, 6, and 10 copies of PEG5K chains, respectively, in PBS at pH 7.4. The inset in A shows the % quenching (decrease) in the fluorescence of albumin as a function of number of PEG5K chains conjugated. B: Fluorescence spectra of albumin in the presence of free monomethoxy PEG 5K. Curves a, e to h in B represent the fluorescence of HSA in the presence of 0, 5, 10, 15, and 20 equivalents of free PEG5K chains per HSA in PBS at pH 7.4. Note that free PEG in solution increases the fluorescence of HSA, in a PEG concentration-dependent fashion, whereas the conjugated PEG-chains quench the fluorescence of albumin. The inset shows the % increase in the fluorescence of albumin.
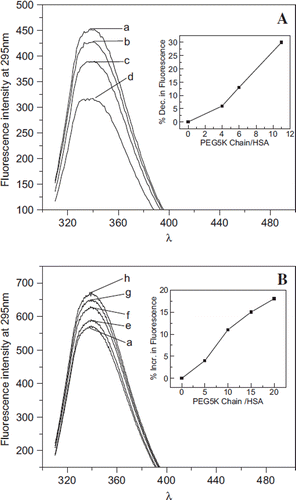
The influence of the presence of monomethoxy PEG (not conjugated to protein) in the solution on Trp fluorescence of albumin is presented in . Interestingly, the influence of free PEG5K in solution is to enhance the fluorescence of albumin in contrast to the PEG chains that are conjugated to albumin. The inset shows the increase in florescence of albumin is a direct correlate of the concentration of monomethoxy PEG5K in solution. Thus the influence of generating the PEG-shell on HSA by conjugating the PEG-chains to albumin is very distinct as compared to the influence of free (unbound PEG) on the overall conformation of albumin as reflected by Trp fluorescence of albumin.
Influence of Extension Arms Sandwiched Between Protein Core and PEG Shell on Structure/Conformation of Albumin. Albumin is the first protein to be PEGylated [Citation15, Citation16], and since then it has been PEGylated using different conjugation chemistries [Citation3, Citation5, Citation6, Citation12, Citation17–24]. EAF-PEGylation of albumin, a new simplified strategy for PEGylation of protein [Citation3], has also been applied to PEGylate albumin. HexaPEGylated albumin (P5K6-Alb) has been investigated extensively as a potential plasma expander.
The role of extension arms in EAF-P5K6-Hb as compared to P5K6-Hb in terms of reducing the impact of the PEG-shell on the structure of protein core, particularly on the interdimeric interactions of Hb has been established [Citation22, Citation25], making EAF PEGylation of Hb the preferred approach over the direct PEGylation platforms [Citation26]. Because HSA is a single chain protein, the dissociation is not a concern, but the perturbation of the protein structure by PEGylation is of interest in establishing whether EAF-P5K6-HSA is a better material to develop it as therapeutic material. The solution and the molecular properties of EAF-hexaPEGylated albumin has been compared below () with that of the hexaPEGylated albumin generated using isothicyanato chemistry based on direct PEGylation [Citation12].
Table 2. Comparison of molecular and solution properties of hexaPEGylated HSA
Influence of Extension Arm Chemistry on the PEGylation Induced Plasma Expander Like Properties. The molecular and solution properties of EAF-hexaPEGylated albumin are compared with that of (TCP-PEG5K)6 HSA in .
The PEGylation induced increase in the viscosity of the EAF and direct PEGylated albumin are nearly comparable. The COP increase on EAF hexaPEGylated HSA is slightly higher as compared to the direct PEGylation protocol. On the other hand, EAF hexaPEGylated HSA product exhibits a molecular radius higher by about 1 nm as compared to the hexaPEGylated product generated by direct PEGylation. Accordingly, packing density of PEG within the PEG-shell of albumin hexaPEGylated by direct PEGylation is significantly higher. The PEGylation induced PE-like properties of PEG-Alb appear to be slightly better when the extension arm chemistry is used.
A comparison of the SEC pattern of EAF-(SP-PEG5K)6 HSA with that of (TCP-PEG5K)6-HSA is presented in . It may be seen that the hydrodynamic volume of the EAF-hexaPEGylated albumin is only marginally larger than that of the (TCP-PEG5K)6-HSA, even though the molecular volume increase with (EAF-P5K6)-HSA is nearly 33 % larger than that seen with (TCP-PEG5K)6-HSA. The elution position of the EAF (SP-PEG5K)6 HSA corresponds to the position of a globular protein of a molecular weight of 300 KDa, and that of (TCP-PEG5K)6-HSA is slightly lower, even though the actual molecular mass of these products is around 96 KDa. The hydrodynamic volume of the two hexaPEGylated HSA under the flow conditions is comparable, even though molecular radius of (TCP-PEG5K)6-HAS is significantly lower as compared to the EAF PEGylated product. The results suggest that the PEG shells of the two-hexaPEGylated proteins are deformed (perturbed) to nearly the same degree under the SEC flow conditions.
Figure 5. Influence of extension arms on molecular, solution, and conformational properties of HexaPEGylated albumin. A: Hydrodynamic volume of hexaPEGylated albumin as reflected by SEC: a: HSA; b: EAF-P5K6- HSA; and c: (TCP-PEG5K)6-HSA. B: Apparent molecular mass of hexaPEGylated HSA as reflected by SDS-PAGE: a: HSA; b: EAF-P5K6-HSA; c: (TCP-PEG5K) 6-HSA. C: Influence of extension arm on the secondary structure (overall α-helical conformation) of hexaPEGylated HSA as reflected by Far UV circular dichroism. D: Influence of extension arm on the overall tertiary structure of hexaPEGylated albumin as reflected by tryptophyl fluorescence (a: HSA; b: EAF-P5K6-HSA; c: (TCP-PEG5K)6-HSA).
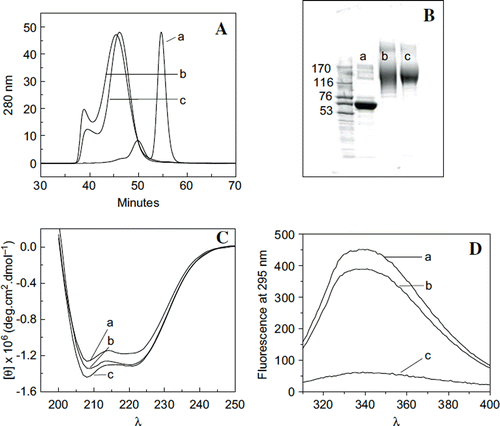
The SDS-PAGE pattern of the two PEGylated proteins is shown in along that of molecular markers. Both proteins exhibit an apparent molecular mass around 160 KDa. This molecular mass is still larger than the actual molecular mass of the two proteins, i.e. 96 KDa. It may also be noted that the pattern of (EAF-PEG5K)6-HSA is slightly more diffused than that of (TCP-PEG5K)6-HSA. However, it is not clear whether it is a reflection of the presence of extension arm, or a reflection of some other differences in the structure of these two proteins.
Influence of HexaPEGylation of Albumin on its Overall Conformation. Influence of extension arms on the HexaPEGylation induced perturbation of the secondary structure of albumin. The secondary structure (overall α-helical conformation) of the two-hexaPEGylated albumins are compared with that of albumin using far UV circular dichroic spectroscopy. As shown in , hexaPEGylation of HSA by EAF PEGylation using PEG5K increases the ellipticity around 210 nm only marginally. This probably reflects the increased hydrophobicity of the microenvironment of the protein due to the presence of the PEG-shell. The far UV circular dichroic spectra of (TCP-PEG5K)6-HSA is not significantly different from that of (EAF-PEG5K)6-HSA. The increase in the overall α-helical content of the protein on hexaPEGylation is small. The extension arms have very little influence on the PEGylation induced increase in the α-helical conformation (secondary structure) of the protein core, HSA.
Influence of extension arms on the hexaPEGylation induced changes in the tertiary interactions of albumin. The Trp florescence of HSA is an indicator of the perturbations of the overall tertiary interactions of the protein on PEGylation. Trp fluorescence of HSA is extensively quenched when it is hexaPEGylated using thiocarbamoylation chemistry-based PEGylation. This apparently suggests the increased hydration of the microenvironment of Trp by the PEG-shell. On the other hand, fluorescence quenching is very small when it is hexaPEGylated using EAF-PEGylation. The extension arms attenuate the influence of the PEGylation-induced perturbations of the tertiary interactions of albumin.
Thermal Stability of HexaPEGylated Albumin: Influence of Extension Arm on the Thermal Transition of HSA. The thermal transition of the two hexaPEGylated albumin, with and without extension arms, have been studied by differential scanning calorimetry and compared with that of HSA (). Thiocarbmoylation-based hexaPEGylation increases the thermal transition by nearly 10°C. Extension arms engineered between the PEG chains and protein amino groups inhibit the PEGylation-induced increase in the transition temperature of albumin. The buffering zone generated by the extension arm between the protein core and PEG-shell buffers the influence of PEG-shell on the protein structure. However, this does not mean that PEG has no influence on the thermal transition of the protein. The EAF-PEGylation appears to lower the thermal transition temperature of albumin slightly. The enthalpy changes associated with thiocarbamoylation-based PEGylation is also significantly higher than that of the control, but slightly smaller than that of EAF hexaPEGylated albumin, but the transition is at a higher temperature.
Figure 6. Influence of extension arm on the thermal transition of HexaPEGylated albumin. Scanning microcolorimetry has been used to generate the thermal transition curves. Deconvoluted curves for the experimentally generated transition curves are also shown. A: Control HSA; B: EAF-P5K6-HSA; and C: TCP P5K6-HSA.
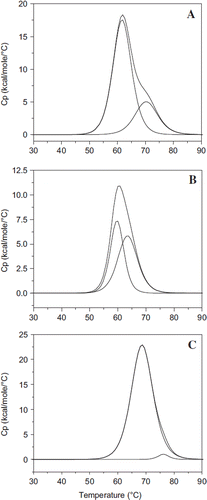
The experimentally determined transition curves of hexaPEGylated albumin have also been deconvoluted into a two-component system. The transition with Albumin is convoluted into two components with the transition temperatures of 61.7 and 70.4, respectively (). The two components are present in the ratio of approximately 4:1; the enthalpy changes associated with these two are also provided in the table. The thermal transition curve of EAF hexaPEGylated albumin could also be resolved into two components of the transition temperatures of 59.8°C and 63.5°C of nearly equal amounts, respectively (). It may be noted that both components exhibit a lower transition temperature, as compared to the respective components of the parent molecule. The first component is associated with a lower enthalpy change compared that of the parent, but the second component has the enthalpy changes comparable to that of the second component of the parent molecule. In the case of the direct PEGylated albumin, the decovolution of the thermal transition curve gave essentially a single component, with trace amounts of the second component, with the transition temperatures of 68.7 and 76.2°C, respectively (). The enthalpy change associated with the first transition is significantly higher than that of the parent molecule, in addition to the transition temperatures being higher than that of the control sample. The enthalpy change of the second component is very small. Thus extension arms between the PEG-chains and the protein amino groups exert an attenuating influence on PEG-shell-induced stabilization of the thermal transition pattern of albumin. Further detailed studies of thermal transition of EAF PEGylated albumin as a function of PEGylation will be necessary to understand the role of extension arm in attenuating the impact of the PEG-shell on the protein structure.
Table 3. Influence of extension arms on the thermal transitions of HexaPEGylated albumin
Viscosity and Colloidal Osmotic Pressure of hexaPEGylated Albumin as a Function of Concentration of the Adducts: Influence of the Molecular Size of PEG-chain on the Solution Properties. viscosity. The viscosity of HSA and of hexaPEGylated HSA is presented in as a function of adducts’ concentration expressed as protein concentration. The viscosity of albumin shows a small linear increase as the concentration of PEG-protein adduct is increased from 10 mg/ml to 70 mg/ml. HexaPEGylation of albumin with PEG5K as well as PEG3K increases the viscosity of albumin; the viscosity increase is higher with PEG5K at all protein concentrations (the concentration of the PEG-albumin adducts is nearly 1.5 times than that of protein). The viscosity of hexaPEGylated albumins (both with PEG5K and 3K) increases exponentially as a function of the protein concentration; at every protein concentration studied the viscosity of hexaPEGylated material generated using PEG5K was higher than that with PEG3K ().
Figure 7. Viscosity (A) and colloidal osmotic pressure (B) of HSA hexaPEGylated with SP-PEG3K and SP-PEG5K as a function of protein concentration; viscosity (C) and colloidal osmotic pressure (D) of HSA hexaPEGylated with ITPPEG3K and ITPPEG5K as a function of protein concentration. Insets show increased viscosity and COP of the four hexaPEGylated albumins as function of PEG mass.
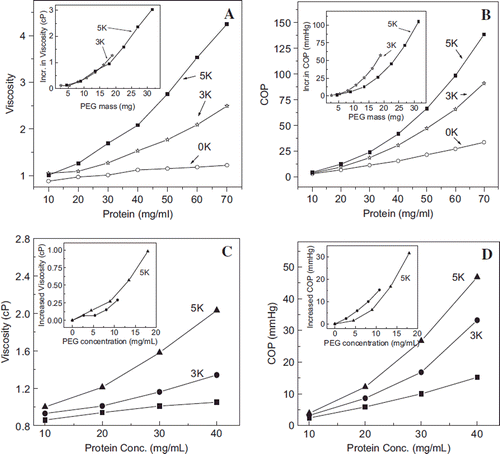
The inset shows the correlation in increase in the viscosity of the two hexaPEGylated albumins when the concentrations of the PEGylated albumins are normalized to the mass of PEG in each sample. It may be seen that increase in viscosity as a function of PEG mass is still exponential, but the values for the two PEGylated proteins essentially merge into a single exponential pattern. This suggests that the viscosity of the PEGylated albumin in the hexaPEGylation pattern is essentially a correlate of PEG mass conjugated to the protein.
The viscosity data is shown for albumin hexaPEGylated with PEG5K and PEG3K using thiocarbamoylation chemistry in . The inset shows that on a PEG weight basis, albumin PEGylated with PEG3K exhibits a lower viscosity than PEG5K, a pattern that is distinct from that of EAF PEGylated albumin. This may be related to the extension arm; the extension arms increase the flexibility and deformability of the PEGylated albumin.
Colloidal Osmotic Pressure. COP of the albumin EAF hexaPEGylated generated using maleimide PEG5K as well as PEG3K is shown in as a function of protein concentration. The increase in the COP of EAF PEGylated HSA is exponential whereas the increase in the COP of albumin is linear with little difference as the concentration is increased. At a given concentration, the PEG3K adducts exhibit a lower COP as compared to PEG5K, a reflection of the PEG mass dependence of PEGylation-induced COP. In the inset, the COP of hexaPEGylated albumin is presented as amount of PEG mass in solution. Surprisingly, in the EAF hexaPEGylation pattern, surface decoration with PEG3K is more efficient in increasing the COP of the PEG-protein adducts when compared to the basis of PEG mass in the solution.
The correlation of the actual mass of PEG in thiocarbamoylated hexaPEGylated albumin is presented in the inset in , and again on an actual mass base the PEG3K in the hexaPEGylation pattern is more efficient in inducing COP to albumin than PEG5K. Thus chemistry of conjugation appears to have this better efficiency of lower mass PEG-chain to induce COP to the protein.
DISCUSSION
The lack of a linear non-correlation of the increase in molecular radius of albumin on increasing the number of PEG-chains conjugated implies that as the number of PEG chains increases, the inter PEG-chain interactions increase, i.e. PEG chains pack tighter between themselves as the number of chains in the PEG-shell increase. In a previous study [Citation14], the biphasic nature of the increase in the molecular radius of protein on PEGylation was suggested to indicate the beginning of the transition of PEG protein from a mushroom (cloud-like) to a brush conformation.
The PEG packing density increases almost linearly as a function of PEG mass for PEGylation with PEG 5K, whereas in the case of PEG-3K the correlation appears to be linear initially, and with a tendency to plateau at the last stages of the increase in the PEG mass. Since the brush confirmation of PEG shell implies a rigid configuration for the PEG-chains the plateau stage seen with PEG 3K should represent a stage when new PEG chains are added, the resulting increase in the PEG mass in the PEG shell correlates directly with the increase in the molecular volume. The role of molecular surface area protein, i.e. changing surface density PEG chains, in dictating the structure of the PEG shell (molecular volume) is not addressed in calculating the packing density of the PEGylated albumin, as the molecular surface remains the same during the course of PEGylation.
The present studies highlight the fact that PEGylated proteins represent a new class of hybrid biopolymers with a unique molecular structure with two distinct regions of differing packing densities of the atoms. The packing density in the PEG-shell is not a function of the number of PEG chains joined or the total mass of the PEG chains conjugated to the protein core. The packing density of the PEG albumin with PEG-3K is higher than that with PEG5K in any given pattern of PEGylation. Even when the data is corrected for molecular mass, it is clear that PEG 3K is packed more compactly in the PEG shell than PEG 5K. Thus packing density of PEG shell of albumin suggests its structure can not be explained in terms of two very distinct conformational states, mushroom vs brush conformation. Individual PEG albumins are distinct molecular species, their structure being dictated by the pattern, PEG-chain length, number, and chemistry of PEGylation.
The increase in the viscosity and COP of Alb (at 4 gm %) as a function of number of PEG-5K chains exhibits an exponential behavior. On the other hand, the increase in viscosity and COP for PEG-albumin as of number of PEG3K chains conjugated is more of a linear correlate of the number of the PEG-chains. These PEGylation-induced solution properties of albumin have been correlated as a function of the number of oxyethylene units per HSA molecule. Surprisingly, the oxyethylene units provided as a part of longer PEG5K chains induce better (higher) COP and viscosity than the PEG3K-chains. The PEGylation-induced increase of the solution properties by given mass of PEG is a reverse correlate of the packing density of PEG in the PEG-shell. The study suggests that the use of PEG 10 K with three copies (instead of six copies of PEG5K) may generate better products, i.e. with lower packing density and higher COP and viscosity (as compared to the hexaPEGylated molecule generated using PEG 5K) and may be a better material as active supra plasma expanders.
The EAF PEGylation of albumin quenches the intrinsic florescence of Trp residue of albumin. The quenching also appears to be a direct correlate of the extent of PEGylation with 5K; i.e., structure of the PEG-shell. On the other hand, the quenching of the fluorescence induced by hexaPEGylation using thiocarbamoylation chemistry is significantly higher. The influence of PEGylation on fluorescence of albumin appears to be related to the chemistry of the PEGylation. This influence apparently originates from the impact of the PEG chains on the protein hydration layer of albumin and the concomitant influence on the intramolecular interactions of albumin. Since the higher PEG density can be considered as the increased order in the structure of PEG-chains within the PEG-shell, increase in the extent of PEGylation may be considered as the increased strength of the barrier between the protein hydration layer and the bulk water. The larger quenching effect seen with direct PEGylation is apparently a result of the absence of extension arms, and the high PEG-density of the PEG cell of (TCP-PEG-5K)6-HSA.
The extension arms appear to minimize the influence of the PEG-shell on protein hydration layer. The extension arm of the EAF-PEGylated protein places the PEG shell further away from the protein surface as compared to the PEG-chains conjugated directly to the side chains of protein (). This concept is supported by the differential scanning microcalorimetric studies as well. EAF-PEGylation has very little influence on the experimentally determined transition temperature of albumin. On the other hand, the thermal transition temperature of albumin is increased by about 10°C when it is hexaPEGylated using thiocarbamoylation chemistry. Thus the role of the extension arm appears to be to minimize the influence of the perturbation of the structure of the protein core by PEG-shell. This is consistent with the fact that EAF hexaPEGylation of Hb is the only protocol that does not perturb the interdimeric interactions of the protein [Citation3].
Figure 8. Comparison of the chemistry of conjugation of PEG chains between the EAF mode and the direct mode. The presence of extension arm pushes the PEG-chain away from the protein surface into the bulk water. Thus the interactions of the PEG-chains with the protein molecular surface should be lowered relative to direct PEGylation, and the interactions with the bulk solvent should be increased. Thus the extension arms may be expected to influence and/or modulate the influence of PEGylation on the structure and the functional properties of the PEGylated proteins.
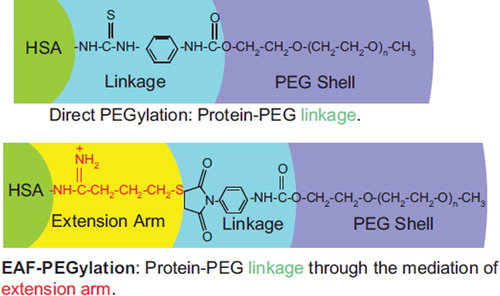
Accordingly, we conclude that stabilizing influence of the PEG-shell on the structure of the protein core is reduced by the presence of extension arms engineered between the protein core and PEG-shell. The present studies using two PEG-chains of different molecular sizes have suggests that the PEGylation-induced molecular radius, viscosity, and COP can be manipulated independently by understanding the structure of this new class of semisynthetic hybrid biopolymers and designing the product to have the viscosity and/or COP desired for a particular application as we move onto the next phase of designing “super perfusion fluids.” In addition, since the extension arms attenuate the impact of the PEG shell on the protein core as compared to direct PEGyation samples, we speculate that EAF PEGylation of therapeutic proteins is likely to generate product with better efficacy. It is known that direct PEGylation of therapeutic proteins generally lowers the biological activity in vitro, but the therapeutic efficacy increases as a result of increased half-life of the proteins. It is anticipated that EAF PEGylation will lower the PEGylation-induced decrease in the in vitro biological activity, and thus could lead to an increased efficacy.
Declaration of interest: The authors report no conflicts of interest. The authors alone are responsible for the content and writing of the paper.
REFERENCES
- Manjula, B. N., Malavalli, A., Smith, P. K., Chan, N. L., Arnone, A., Friedman, J. M., and Acharya, A. S. (2000). Cys-93-betabeta-succinimidophenyl polyethylene glycol 2000 hemoglobin A. Intramolecular cross-bridging of hemoglobin outside the central cavity. J Biol Chem, 275: 5527–5534.
- Winslow, R. M. (2000). Safety and utility of blood substitutes. Dev Biol (Basel), 102: 167–182.
- Manjula, B. N., Tsai, A., Upadhya, R., Perumalsamy, K., Smith, P. K., Malavalli, A., Vandegriff, K., Winslow, R. M., Intaglietta, M., Prabhakaran, M., Friedman, J. M., and Acharya, A. S. (2003). Site-specific PEGylation of hemoglobin at Cys-93(beta): Correlation between the colligative properties of the PEGylated protein and the length of the conjugated PEG chain. Bioconjug Chem, 14: 464–472.
- Wettstein, R., Tsai, A. G., Erni, D., Winslow, R. M., and Intaglietta, M. (2003). Resuscitation with polyethylene glycol-modified human hemoglobin improves microcirculatory blood flow and tissue oxygenation after hemorrhagic shock in awake hamsters. Crit Care Med, 31: 1824–1830.
- Wettstein, R., Cabrales, P., Erni, D., Tsai, A. G., Winslow, R. M., and Intaglietta, M. (2004). Resuscitation from hemorrhagic shock with MalPEG-albumin: comparison with MalPEG-hemoglobin. Shock, 22: 351–357.
- Acharya, S. A., Friedman, J. M., Manjula, B. N., and Intaglietta, M., Tsai, A. G., Winslow, R. M., Malavalli, A., Vandegriff, K., Smith, P. K. (2005). Enhanced molecular volume of conservatively pegylated Hb: (SP-PEG5K)6-HbA is non-hypertensive. Artif Cells Blood Substit Immobil Biotechnol, 33: 239–255.
- Cabrales, P., Tsai, A. G., Winslow, R. M., Intaglietta, M. (2005). Extreme hemodilution with PEG-hemoglobin vs. PEG-albumin. Am. J. Physiol. Heart Circ. Physiol, 289: H2392–2400.
- Manjula, B. N., Tsai, A. G., Intaglietta, M., Tsai, C. H., Ho, C., Smith, P. K., Perumalsamy, K., Kanika, N. D., Friedman, J. M., and Acharya, S. A. (2005). Conjugation of multiple copies of polyethylene glycol to hemoglobin facilitated through thiolation: Influence on hemoglobin structure and function. Protein J, 24: 133–146.
- Cabrales, P., Tsai, A. G., Ananda, K., Acharya, S. A., and Intaglietta, M. (2008). Volume resuscitation from hemorrhagic shock with albumin and hexaPEGylated human serum albumin. Resuscitation, 79: 139–146.
- Martini, J., Cabrales, P., K., A., Acharya, S. A., Intaglietta, M., and Tsai, A. G. (2008). Survival time in severe hemorrhagic shock after perioperative hemodilution is longer with PEG-conjugated human serum albumin than with HES 130/0.4: A microvascular perspective. Crit Care, 12: R54.
- Grassetti, D. R., and Murray, J. F., Jr. (1967). Determination of sulfhydryl groups with 2,2′- or 4,4′-dithiodipyridine. Arch Biochem Biophys, 119: 41–49.
- Meng, F., Manjula, B. N., Smith, P. K., and Acharya, S. A. (2008). PEGylation of human serum albumin: Reaction of PEG-phenyl-isothiocyanate with protein. Bioconjug Chem, 19: 1352–1360.
- Acharya, A. S., and Taniuchi, H. (1980). Influence of solution conditions on the formation of an intermediate from hen egg lysozyme containing non-native disulfide bonds during thiol-dependent renaturation. J Biol Chem, 255: 1905–1911.
- Sahu, R. K., Nacharaju, P., Manjula, B. N., and Acharya, S. A. (2009). Induced plasma expander-like properties as a function of PEG-chains on extension arm facilitated PEGylation of albumin: “Mushroom to brush-like” conformational transition of the PEG-albumin conjugate. Artif Cells Blood Substit Immobil Biotechnol, 37: 245–256.
- Abuchowski, A., McCoy, J. R., Palczuk, N. C., van Es, T., Davis, F. F. (1977). Effect of covalent attachment of polyethylene glycol on immunogenicity and circulating life of bovine liver catalase. J Biol Chem, 252: 3582–3586.
- Abuchowski, A., van Es, T., Palczuk, N. C., Davis, F. F. (1977). Alteration of immunological properties of bovine serum albumin by covalent attachment of polyethylene glycol. J Biol Chem 252: 3578–3581.
- Winslow, R. M., Lohman, J., Malavalli, A., Vandegriff, K. D. (2004). Comparison of PEG-modified albumin and hemoglobin in extreme hemodilution in the rat. J Appl Physiol, 97: 1527–1534.
- Huang, Y., Komatsu, T., Yamamoto, H., Horinouchi, H., Kobayashi, K., Tsuchida, E. (2006). PEGylated albumin-heme as an oxygen-carrying plasma expander: Exchange transfusion into acute anemia rat model. Biomaterials, 27: 4477–4483.
- Li, D., and Manjula, B. N., and Acharya, A. S. (2006). Extension arm facilitated PEGylation of hemoglobin: Correlation of the properties with the extent of PEGylation. Protein J, 25: 263–274.
- Acharya, S. A., Acharya, V. N., Kanika, N. D., Tsai, A. G., Intaglietta, M., Manjula, B. N. (2007). Non-hypertensive tetraPEGylated canine haemoglobin: Correlation between PEGylation, O2 affinity and tissue oxygenation. Biochem J, 405: 503–511.
- Li, D., Manjula, B. N., Ho, N. T., Simplaceanu, V., Ho, C., Acharya, A. S. (2007). Molecular aspects of the high oxygen affinity of non-hypertensive hexa pegylated hemoglobin, [(SP-PEG5K)(6)-Hb]. Artif Cells Blood Substit Immobil Biotechnol, 35: 19–29.
- Li, D., Hu, T., Manjula, B. N., and Acharya, S. A. (2008). Non-conservative surface decoration of hemoglobin: Influence of neutralization of positive charges at PEGylation sites on molecular and functional properties of PEGylated hemoglobin. Biochem Biophys Acta, 1784: 1395–1401.
- Nacharaju, P., Manjula, B. N., Acharya, and S. A. (2007). Thiolation mediated pegylation platform to generate functional universal red blood cells. Artif Cells Blood Substit Immobil Biotechnol, 35: 107–118.
- Meng, F., Manjula, B. N., Tsai, A. G., Cabrales, P., Intaglietta, M., Smith, P. K., Prabhakaran, M., and Acharya, S. A. (2009). Hexa-thiocarbamoyl phenyl PEG5K Hb: vasoactivity and structure: Influence of rigidity of the conjugation linkage on the PEGylation induced plasma expander-like solution properties of PEG-Hb adducts. Protein J, 28: 199–212.
- Ananda, K., and Acharya, S. A. (2008). Role of extension arm in PEG-Hb conjugates on the stability of the tetramer: Non-conservative EAF maleimide thio-PEG mediated PEGylation. Artif Cells Blood Substit Immobil Biotechnol, 36: 499–512.
- Li, D., Hu, T., Manjula, B. N., and Acharya, S. A. (2009). Extension arm facilitated pegylation of alphaalpha-hemoglobin with modifications targeted exclusively to amino groups: functional and structural advantages of free Cys-93(beta) in the PEG-Hb adduct. Bioconjug Chem, 20: 2062–2070.
- Winslow, R. M. (2004) MP4, a new nonvasoactive polyethylene glycol-hemoglobin conjugate. Artif Organs, 28: 800–806.