Abstract
Abstract: In this paper, a novel amperometric phenol biosensor with immobilization of polyphenol oxidase (tyrosinase) on electrochemically polymerized polypyrrole-polyvinylsulphonate (PPy-PVS) film has been accomplished via the entrapment technique on the surface of a platinum electrode. The amperometric determination is based on the electrochemical reduction of quinon generated in the enzymatic reaction of phenol. The effects of pH and temperature were investigated and optimum parameters were found to be 8.0 and 30 °C, respectively. The linear working range of the electrode was 1.0 × 10−7 – 5.0 × 10−6 M. The storage stability and operation stability of the enzyme electrode were also studied.
INTRODUCTION
Phenolic compounds are released into the environment by industrial wastes [Citation1]. Phenols exist as pollutants of air, water, and soil. Phenol is easily adsorbed by humans, regardless of the type of exposure. High levels of phenol cause damage to animal health. Prolonged oral or subcutaneous exposure causes damage to the lungs, liver, kidney, and genito-urinary tract. In view of major concern regarding toxicity of these compounds, there is a growing need for innovative devices to monitor phenols in complex environments, food, pharmaceutical and industrial matrices. Determinations of phenol compounds have an important role in the field of biomedicine and environmental science. There are many methods for phenol determination, such as gas chromatography and spectrophotometric analysis [Citation2, Citation3]. However, these methods suffer from complicated pretreatments. An amperometric biosensor based on an enzyme, tyrosinase, has been considered to be a promising method because of its effectiveness and simplicity [Citation4–7].
For phenolic compounds’ determinations, polyphenol oxidase (also known as tyrosinase, EC 1.14.18.1), which is a copper-containing enzyme, is used [Citation8]. This enzyme catalyzes phenol's oxidation and o-quinone takes form as a product of the enzymatic reaction. This is accomplished in two reaction steps. In the first step, tyrosinase oxidizes phenol into the corresponding cathecol. In the second step, this cathecol is reoxidized into o-quinone. Amperometric reduction of the generated quinone is then used as the quantification method [Citation9,Citation10].
A variety of methods for the immobilization of tyrosinase with an electrochemical transducer have been reported, such as cross-linking on the surface of electrodes [Citation11–13], incorporation within a carbon paste matrix [Citation14, Citation15], and entrapment in polymer films [Citation8, Citation16].
In this paper we report the immobilization of polyphenol oxidase onto PPy–PVS film via an entrapment procedure for determination of phenol. The parameters affecting the performance of the biosensor (substrate concentrations, the pH, and temperature) were investigated.
MATERIAL AND METHODS
Instrumentation and Reagents
All electrochemical experiments were carried out using an CHI 660B Electrochemical Workstation conventional three-electrode system equipped with a Pt plate (0.5 cm2) as the working electrode, an Ag/AgCl electrode (3 M KCl) as the reference electrode, and a platinum wire (diameter and length, 1 mm, 4 cm, respectively) for the counter electrode and with an activity of 0.12 mg mL−1, and polyphenol oxidase was purchased from Sigma. Pyrrole and sodium polyvinylsulphonate were supplied by Fluka. All other chemicals were obtained from Sigma. All the solutions were prepared using distilled water.
Preparation of Pt/PPy–PVS Film Electrode
The surface of the Pt plate electrode was cleaned [Citation17] and covered with a conducting polypyrrole in polyvinylsulphonate media [Citation18]. The electrode was immersed in an 0.1 M pyrrole monomer in aqueous solution of the sodium salt of polyvinylsulphonate (25%). The solution was purged with argon in order to remove the oxygen. The electropolymerization of pyrrole upon the electrode surface was performed by the cyclic voltammetry between −1.0 and +2.0 V at a scan rate of 100 mV/s and 4 cycles (). The electrode was washed with buffer solution after the coating process.
Immobilizaton of Polyphenol Oxidase on Pt/PPy-PVS Film Electrode
A Pt/PPy-PVS-Polyphenol oxidase film electrode was used against the Pt electrode, which was used as the counter electrode. The reference electrode was composed of Ag/AgCl. Immobilization of polyphenol oxidase (PPO) was carried out by the physical entrapment approach. The electrode was immersed in 0.1 M pyrrole monomer in aqueous solution of the sodium salt of polyvinylsulphonate (25%) and 2 mL of PPO enzyme (0.12 mg/mL) was added into the solution. The solution was then purged by argon for the removal of oxygen before electropolymerization. Electropolymerization was performed in the mode of cyclic voltammetry to immobilize polyphenol oxidase onto the electrode. The scanning voltage range was from -1.0 to +2.0 V with a scanning rate of 100 mV/s. After the fabrication of polyphenol oxidase entrapped Polypyrrole film onto the PPy–PVS working electrode was finished, the electrode was rinsed with deionized water to remove the unreacted pyrrole monomer and free polyphenol oxidase. Immobilized enzyme electrode was kept in a refrigerator at 4°C in phosphate buffer when it was not in use.
Amperometric Biosensor Measurements
Amperometric response studies were carried out in phosphate buffer. Operational stability, storage stability, pH, and temperature were determined via application of −0.09 V (versus Ag/AgCl) by detecting the reduction current of quinon. After the background current reached a stable value, phenol solution was added to the cell using a micropipette and stirred for 10 min. Then the resulting current difference was recorded. Research on operational and storage stability as well as effects of pH and temperature was carried out using 1.0 × 10−6 M concentration of phenol.
RESULT AND DISCUSSION
In this study, we prepared a new phenol biosensor with the entrapment of polyphenol oxidase (PPO) on poly(pyrrole)-polyvinylsulphonate (PPy-PVS) composite film. The parameters affecting the performance of the biosensor were examined.
The Working Potential
In 0.1 M pH = 8.0 phosphate buffer solution, electrochemical behavior of quinone was investigated with square wave voltammetry. The reduction peak potential of quinone was found to be −0.09 V ().
Effects of pH and Temperature
Immobilized polyphenol oxidase enzyme showed maximum activity at pH 8.0 and 30 °C. The buffers at various pH values were tested to investigate the effect of pH. The pH values of the buffers were varied between 6.0 and 10.0. The measurements were performed at a constant phenol concentration of 1.0 × 10−6 M. shows that the maximum response was obtained at pH 8.0. For phenol biosensor, pH values different from 8.0 were employed in literature (pH 7.5, 7.0) [Citation16, Citation19]. This was attributed to the fact that the used polymer and the type of immobilization were different.
Figure 3. The effect of pH on the response of the biosensor (in the phosphate buffer containing 1.0 × 10−6 M phenol, operating potential is −0.09 V, 30°C).
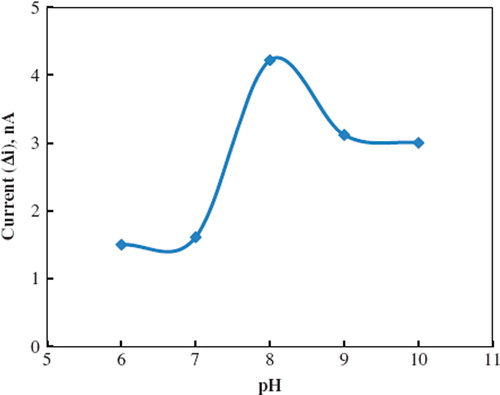
Temperature has a great effect on enzyme activity and it is important to investigate the dependence of the response of the enzyme electrode to the temperature. The temperature influence on the response of phenol enzyme electrode was tested between 25°C and 45°C at pH 8.0 using constant phenol concentration of 1.0 × 10−6 M (). As seen from the figure, the electrode response increases with temperature up to 30 oC and decreases afterwards. The highest electrode response was obtained at 30°C. For phenol biosensor, temperature values different from 30 oC were employed in literature (40°C, 21 oC) [Citation11, Citation19]. This was attributed to the fact that the used polymer and the type of immobilization were different.
Effect of Substrate Concentration on Biosensor
The value of the Michaelis–Menten kinetic parameter (Km), which shows the enzyme–substrate kinetics, was determined by the analysis of the graph of enzymatic reaction. Vmax is the maximum rate for enzymatic reaction. The effect of the substrate concentration on the reaction rate, catalyzed by immobilized PPO, was studied by using different concentrations (1.0 × 10−7 – 1.0 × 10−4 M) of phenol substrate (). With the increase in substrate concentration, there was an increase in amperometric current signal. There were linear parts ranging between 1.0 × 10−7−5.0 × 10−6 M (R2: 0.99). The graph for the calibration curve is given in . It has been shown that the linearity of the graph was highly satisfactory and it can be used for the quantitative determination of phenol. The detection limit of the biosensor was 1.0 × 10−8 M and the response time of the biosensor was 300 s. Kinetic parameters Km(app) and Imax for the enzyme biosensor were detected at constant temperature (30 °C) and pH (pH 8.0) in varying substrate concentrations. Km(app) and Imax were calculated as 2.5 μM, 0.125 μA/min, respectively, using 1/[phenol]-1/Δi graph (Lineweaver-Burk). Km values for immobilized PPO presented in the literature are 100.0 mM, 70 μM [Citation16,Citation19].
Operational Stability and Storage Stability
The biosensor was used at optimum conditions for 10 activity assays in one day to determine the operational stability. Storage stability of the biosensor was determined by performing activity assays within 45 days. The operational stability was studied by applying activity assay (under optimum conditions) 10 times in the same day at constant temperature, pH, and substrate concentration (1.0 × 10−6). At the end of the 10 measurements, the biosensor showed 77 % of its initial activity (). The activity assay was applied within 45 days to display the storage stability of immobilized enzyme. It was observed that 57% of initial activity was lost on the forty-fifth day.
Interference Effect
A few cations found in wastewater, such as Cd2+, Pb2+, Sn2+, As3+, As5+, Cr3+ Cr6+, Sb3+, Mn2+, Co2+, Cu2+, Ni2+, were studied for any interfering effect on the analysis of phenol. 1.0 × 10-2 M − 1.0 × 10-5 M concentrations of cations were added. It was observed that Cd2+, Pb2+, Sn2+, As3+, As5+, Cr3+, Cr6+, Sb3+, Mn2+, Co2+, and Ni2+ had no interfering effects on the analysis of phenol. However, the interfering effect of copper (1.0 × 10−2 − 1.0 × 10−5 M) on the analysis of phenol was observed.
Declaration of interest: The authors report no conflicts of interest. The authors alone are responsible for the content and writing of the paper.
REFERENCES
- Atlow, S.C., Bonadonna-Apro, L., Klibanov, A.M. (1984). Dephenolization of industrial wastewaters catalyzed by polyphenol oxidase. Biotech Bioeng, 26: 599–603.
- Chriswell, C.D., Chang, R.C., Fritz, J.S. (1975). Chromatographic determination of phenols in water. Anal Chem, 47: 1325–1329.
- Poerschmann, J., Zhang, Z.F., Kopinke, D., Pawliszyn, T. (1997). Solid phase microextraction for determining the distribution of chemicals in aqueous matrices. Anal. Chem, 69: 597–600.
- Hall, G.F., Best, D.J., Turner, A.P.F. (1988). The determination of p-cresol in chloroform with an enzyme electrode used in the organic phase. Anal Chim Acta, 213: 113–119.
- Tillyer, C.R., Gobin, P.T. (1991). The development of a catechol enzyme electrode and its possible use for the diagnosis and monitoring of neural crest tumours. Biosens Bioelectron, 6(7): 569–573.
- Imabayashi, S., Kong, Y.T., Watanabe, M. (2001). Amperometric biosensor for polyphenol based on horseradish peroxidase immobilized on gold electrodes. Electroanalysis, 13(5): 408–412.
- Rajesh, W. Takashima, Kaneto, K. (2004). Amperometric tyrosinase based biosensor using an electropolymerized PTS-doped polypyrrole film as an entrapment support. Reactive & Functional Polymers 59(2): 163–169.
- Tembe, S., Inamdar, S., Haram, S., Karve, M. and Souza, S.F.D. (2007). Electrochemical biosensor for catechol using agarose-guar gum entrapped tyrosinase. Journal of Biotechnology, 128(1): 80–85.
- Gutés, A., Céspedes, F., Alegret, S., del Valle., M. (2005). Determination of phenolic compounds by a polyphenol oxidase amperometric biosensor and artificial neural network analysis. Biosensors and Bioelectronics, 20(8): 1668–1673.
- Wang, Y. and Hasebe, Y. (2010) Acridine orange-induced signal enhancement effect of tyrosinase-immobilized carbon-felt-based flow biosensor for highly sensitive detection of monophenolic compounds. Analytical and Bioanalytical Chemistry, 399(3):1151–1162.
- Stanca, E.S., Popescu, I.C. (2004). Phenols monitoring and Hill coefficient evaluation using tyrosinase-based amperometric biosensors. Bioelectrochemistry, 64: 47–52.
- Carralero Sanz, V., Luz Mena, M., González-Cortés, A., Yáñez-Sedeño, P., Pingarrón, J.M. (2005). Development of a tyrosinase biosensor based on gold nanoparticles-modified glassy carbon electrodes: Application to the measurement of a bioelectrochemical polyphenols index in wines. Analytica Chimica Acta, 528: 1–8.
- Wanga, P., Liua, M., and Kan, J. (2009). Amperometric phenol biosensor based on polyaniline. Sensors and Actuators B: Chemical, 140(2): 577–584.
- Rodrıguez, M.C., Rivas, G.A. (2002). Glassy carbon paste electrodes modified with polyphenol oxidase: Analytical applications. Analytica Chimica Acta. 459: 43–52.
- Mailleya, P., Cummings, E.A., Mailley, S., Cosnier, S., Eggins, B.R., McAdams, E. (2004). Amperometric detection of phenolic compounds by polypyrrole-based composite carbon paste electrodes. Bioelectrochemistry, 63: 291–296.
- Kıralp, S., Toppare, L., Yagcı, Y. (2003). Immobilization of polyphenol oxidase in conducting copolymers and determination of phenolic compounds in wines with enzyme electrodes. International Journal of Biological Macromolecules, 33(1-3): 37–41.
- Cochet, M., Maser, W.K., Benito, A.M., Callejas, M.A., Martínez, M.T., Benoit, J.M., Schreiber, J., Chauvet, O. (2001). Synthesis of a new polyaniline/nanotube composite: in-situ polymerisation and charge transfer through site-selective interaction. Chem. Commun, 16: 1450–1451.
- Chaubey, A., Gerard, M., Singhal, M., Singh, V.S., Malhotra, B.D. (2000). Immobilization of lactate dehydrogenase on electrochemically prepared polypyrrole–polyvinylsulphonate composite films for application to lactate biosensors. Electrochim Acta, 46(5): 723–729.
- Xue, H., Shen, Z. (2002). A highly stable biosensor for phenols prepared by immobilizing polyphenol oxidase into polyaniline–polyacrylonitrile composite matrix. Talanta, 57: 289–295.