Abstract
Oral tissue engineering aims to treat and fill tissue deficits caused by congenital defects, facial trauma, or malignant lesion surgery, as well as to study the biology of oral mucosa. The Food and Drug Administration (FDA) and the European Medicines Agency (EMA) require a large animal model to evaluate cell-based devices, including tissue-engineered oral mucosa, prior to initiating human clinical studies. Porcine oral mucosa is non-keratinized and resembles that of humans more closely than any other animal in terms of structure and composition; however, there have not been any reports on the reconstruction of a porcine oral mucosa equivalent, probably due to the difficulty to culture porcine fibroblasts. In this study, we demonstrate the feasibility of a 3D porcine oral mucosa equivalent based on a collagen-GAG-chitosan scaffold, as well as reconstructed porcine epithelium by using an amniotic membrane as support, or without any support in form of epithelial cell sheets by using thermoresponsive culture plates. Explants technique was used for the isolation of the porcine fibroblasts and a modified fibroblast medium containing 20% fetal calf serum was used for their culture. The histological and transmission electron microscopic analyses of the resulting porcine oral mucosa models showed the presence of non-keratinized epithelia expressing keratin 13, the major differentiation marker of non-keratinized oral mucosa, in all models, and the presence of newly synthesized collagen fibers in the lamina propria equivalent of the full-thickness model, indicating the functionality of porcine fibroblasts.
Introduction
Tissue-engineered oral mucosa provides hope for many patients with congenital defects such as cleft palate, and to regenerate tissues lost due to disease, facial trauma, and craniofacial cancer surgery (Garcia-Godoy and Murray Citation2006). For the treatment of these wounds, donor oral tissue is limited and grafting of skin substitutes has several disadvantages due to physiological differences between skin and oral mucosa, such as hair growth and keratinization (Ueda et al Citation2001, Izumi et al. 2003). Additionally, autografts are associated with donor site morbidity (Ophof et al. Citation2008). For these reasons, tissue-engineered oral mucosa, which can be reconstructed according to the needs of the surgeon, emerges as an ideal solution (Feinberg et al. Citation2005). We had previously demonstrated the possibility to reconstruct full-thickness human oral mucosa equivalents mimicking very closely the native non-keratinized human oral mucosa by using collagen-based foams and fibers as scaffolds (Kinikoglu et al. Citation2009 and Kinikoglu et al. Citation2011). These oral mucosa equivalents comprised both a pluristratified epithelium expressing the major differentiation marker of non-keratinized oral mucosa: keratin 13, and a lamina propria equivalent containing newly synthesized collagen fibers. An ultrastructurally well-organized basement membrane expressing laminin 332 and anchoring firmly the epithelium to the underlying lamina propria equivalent was also observed in our models.
So far, only one study investigated the intra-oral implantation of a full-thickness oral mucosa equivalent in an open wound, and it failed (Ophof et al. Citation2008). This study by Ophof and colleagues was conducted in dogs and the oral mucosa equivalents were composed of acellular dermis and autologous keratinocytes, and they did not contain fibroblasts. The aim of the present study was to choose a pig as the large animal model prior to clinical trials, and to test the feasibility of reconstructing various porcine oral mucosa models, with and without fibroblasts, including the ones developed in our laboratory. This knowledge will then be used for the reconstruction and transplantation of human autologous oral mucosa equivalents during clinical trials. The Food and Drug Administration (FDA) and also the European Medicines Agency (EMA) require a large animal model to evaluate cell-based devices prior to initiating human clinical studies (Izumi et al. Citation2004). We chose the pig as our large animal model because of its similarity to the human in size and physiology, and in organ development and disease progression. The pig model allows for deliberately timed studies and, at kill, collection of detailed mucosal tissues (Lunney Citation2007). In fact, the porcine model has been identified as the most similar non-primate to the human, and therefore the most appropriate large animal for tissue engineering studies (Athanasiou et al. Citation2009). Especially for oral mucosa engineering, it is the most attractive choice because porcine oral mucosa is non-keratinized and resembles that of human more closely than any other animal in terms of structure and composition (Squier et al. Citation1991, Collins et al. Citation1981). To our knowledge, a porcine oral mucosa equivalent has not been produced before, probably due to the fact that porcine fibroblasts do not proliferate as well as human fibroblasts do and they do not synthesize enough extracellular matrix under standard conditions (Ouellet et al. Citation2011). Here, we demonstrate for the first time the feasibility of porcine oral mucosa equivalents as full-thickness equivalents based on collagen-glycosaminoglycan (GAG)-chitosan porous scaffolds and co-culture of porcine fibroblasts and epithelial cells, or as epithelial cell sheets cultured on amniotic membranes and on thermoresponsive culture plates.
Materials and methods
Culture of porcine epithelial cells and fibroblasts
Epithelial cells and fibroblasts were isolated from porcine oral mucosa biopsies removed from the non-keratinized cheek region of the oral cavity by using a scalpel. The biopsies were disinfected in Betadine, rinsed in basic growth medium supplemented with antibiotics, and then cut into small pieces in order to increase the efficacy of the enzymes used. The separation of the epithelium from the lamina propria was performed with dispase (GIBCO), 10 mg/mL for 3 h at 4 °C. After separation, epithelium was treated with trypsin 0.5 g/L–EDTA 0.2 g/L for 20 min to extract the cells, which were collected every 10 min. Epithelial cells were grown at 8000–10 000 cells/cm2 on a feeder layer of irradiated human fibroblasts in the epithelial cell medium as follows: DMEM-Ham-F12 2.78/1 (Sigma), 10% fetal calf serum (Hyclone), 0.4 μg/mL hydrocortisone (Upjohn), 0.12 UI/mL insulin (Umuline, Lilly), 0.033 μg/mL selenium (Laboratoire Aguettant), 0.4 μg/mL isoprenaline hydrochloride (Isuprel, Sterling Winthrop), 2 × 10 −9 m tri iodo thyronine (Sigma), 10 ng/mL epidermal growth factor (Austral Biologicals), and antibiotics. The isolation of fibroblasts was performed using the explants method. Small pieces (1 mm2) of lamina propria were plated on Petri dishes and the cells were allowed to migrate out of biopsies during 10 days. Then, the fibroblasts were trypsinized, seeded in tissue culture flasks at a density of 10,000 cells/cm2, and cultured in a modified fibroblast medium composed of DMEM, 20% fetal calf serum (FCS), and antibiotics. The medium was changed every two days until cell confluence was reached. For the reconstruction of oral mucosa models the cells were used at passage 2 or 3.
Reconstruction of full-thickness porcine oral mucosa equivalents
Full-thickness porcine oral mucosa equivalents were reconstructed using collagen-GAG-chitosan (CGC) porous scaffolds, which were prepared as previously described (Collombel et al. Citation1987). Briefly, types I and III bovine collagens, chitosan (95% deacylated), and chondroitin 4–6 sulfates (LPI, Lyon, France) were dissolved in water and mixed. After mixing, the gel, which contained 72% collagen, 20% chitosan, and 8% GAG, was poured into Snapwell inserts (Costar) and frozen overnight at − 70 °C. The frozen plates were then lyophilized, submerged in 70% ethanol for 24 h, rinsed and equilibrated in 5 mL of DMEM, and incubated at 37 °C with 5% CO2 for a minimum of 24 h. Lamina propria equivalents were prepared by adding a suspension of 2.5 × 105 fibroblasts/cm2 on top of the 4 cm2 CGC foam. Equivalents were then cultured for 21 days in a medium composed of DMEM, 20% fetal calf serum, 10 ng/mL epidermal growth factor, 50 μg/mL ascorbic acid (Bayer). Then, porcine epithelial cells were plated on lamina propria equivalents at a concentration of 2.5 × 105/cm2. Epithelialized oral mucosal substitutes were cultured in epithelial cell medium supplemented with 50 μg/mL ascorbic acid (Bayer) for 3 weeks.
Reconstruction of porcine epithelium on amniotic membranes
The amniotic membrane (AM) was conserved at −80 °C until use. Immediately before use, it was thawed at room temperature and washed several times with sterile phosphate buffered saline (PBS) containing antibiotics. For porcine oral epithelial culture, the epithelial cells of the amniotic membrane were removed by incubation in a solution of trypsine-EDTA (trypsine 0.025%/EDTA 0.01%) at 37 °C for 15 minutes. The AM was then rinsed in an epithelial cell culture medium described in section 2.1 and porcine oral epithelial cells were seeded on top at a concentration of 2.5 × 105/cm2 and were cultured in the same medium for three weeks.
Reconstruction of porcine epithelial cell sheets on thermoresponsive culture plates
Porcine oral epithelial cells were seeded on thermoresponsive UpCell 6 well plates (CellSeed, France) at a concentration of 2.5 × 105/cm2 and were cultured in the epithelial cell culture medium described in section 2.1 for three weeks at 37 °C. At the end of this culture period, the cell sheet that they formed was incubated at 20 °C for 40 minutes and then gently removed from the thermoresponsive surface of the culture plate using forceps.
Histology and immunohistochemistry
For the histological analysis, porcine oral mucosa models were fixed in 4% formaldehyde solution and embedded in paraffin. Sections, 5 μm thick, were cut and stained using hematoxylin–phloxin–saffron (HPS). For the detection of keratin 13 (dilution 1:100) (Chemicon, USA) tissue equivalents were embedded in an optimum cutting temperature compound (OCT) (Tissue-Tek, Sagura, Japan) and frozen at − 20 °C. Then, sections of 5 μm thickness were fixed in acetone for 10 min at − 20 °C and blocked in phosphate buffered saline containing 4% bovine serum albumin (Sigma, USA) and 5% normal goat serum (Chemicon, USA). Primary antibody was incubated for 90 min at room temperature followed by the secondary antibody AlexaFluor 488 IgG (Invitrogen, USA) for 45 min. Propidium iodide stain (Vector Laboratories, USA) was used to counterstain the cell nuclei. Specimens were analyzed with a Nikon Eclipse Fluorescence Microscope.
Transmission electron microscopy
Tissue equivalents were fixed with 2% glutaraldehyde–0.1 m NaCacodylate/HCl, pH 7.4 for 2 h, and postfixed with 1% osmium tetroxide–0.15 m NaCacodylate/HCl, pH 7.4 for 1 h. After dehydration in a growing gradient of ethanol, the samples were embedded in Epon A + B and finally polymerized at 60 °C for 48 h. The blocks were cut using an ultramicrotome and sections of 60–80 nm thickness were contrasted with uranyl acetate and lead citrate. Observations were performed with a JEM JEOL 1400 transmission electron microscope. Images were recorded using an Orius Gatan camera and 20 fibril diameters were measured using a digital micrograph by two observers on three different regions of lamina propria, and average values were calculated.
Statistical analysis
Numbers of cell layers in the epithelia of the three models were determined using HPS micrographs of three samples for each model and three different areas of each image. The statistical analysis of the data was done by using the non-parametric two-tailed Mann-Whitney U test (Statext v1.41) with 5% significance level, α = 0.05. The results were expressed as mean ± standard error of the mean.
Results and discussion
Histology and immunohistochemistry
Histological analysis of the porcine oral mucosa equivalent based on the CGC scaffold showed that porcine fibroblasts were able to migrate into the porous scaffold, proliferate, and synthesize new extracellular matrix, giving rise to a lamina propria equivalent (). The porcine epithelial cells, which had been seeded on top, were able to proliferate and form a pluristratified epithelium consisting of an average number of 5.6 ± 0.3 cell layers (). On the other hand, both the porcine epithelium reconstructed on the AM () and the porcine epithelial cell sheet obtained on thermoresponsive culture plates () were found to be much less thick than that obtained with the CGC scaffold containing fibroblasts, with 1.5 ± 0.4 and 1.4 ± 0.3 cell layers, respectively. The differences in the number of epithelial cell layers between the CGC-based 3D model and the other two models were statistically significant (Mann-Whitney U test, p < 0.001). These thinner epithelia might be due to the absence of fibroblasts in these two models. The interactions of fibroblasts with epithelial cells are known to influence the thickness of the epithelium developed by the latter (Kinikoglu et al. Citation2011). Nevertheless, the porcine oral epithelial cells were able to form intact epithelial cell sheets on both AMs and thermoresponsive culture plates and were easy to handle, proving promising for the treatment of superficial wounds. The porcine epithelial cell sheets could be easily harvested from the thermoresponsive surface of the culture plates by simply reducing the room temperature to 20 °C. In the epithelia of all three models, corneum stratum and corneum granulosum were absent as in native non-keratinized oral mucosa and the superficial cells were seen to retain their nuclei (). Furthermore, all three epithelia strongly expressed keratin 13, which is the major differentiation-associated marker of non-keratinized oral mucosa ().
Transmission electron microscopy
Transmission electron microcopy (TEM) was used to investigate the ultrastructural organization of the three models (). In the epithelia of all models, intercellular junctions such as desmosomes were detected between adjacent epithelial cells (). These intercellular junctions have a crucial role in the epithelium: they hold the cells together (Alberts et al. Citation2002) and thus provide resistance to the epithelium. They regulate epithelial morphogenesis and cell positioning (Runswick et al. Citation2001). In the epithelial cell sheet they were retained intact after removal from the thermoresponsive surface, resulting in a resistant cell sheet. In the lamina propria equivalent of the 3D model, large amounts of newly synthesized collagen fibers were detected by TEM, indicating the functionality of the porcine fibroblasts (). The average collagen fiber diameter measured in the porcine model was 16 nm, whereas that in the human model was measured as 29 nm.
Figure 2. Transmission electron microscopic analysis of the porcine oral mucosa models. Numerous intercellular junctions such as desmosomes (D) were detected in the epithelium of the model based on the CGC scaffold (a), the epithelium cultured on the AM (b), and the reconstructed epithelial cell sheet (c). In the lamina propria equivalent of the CGC-based 3D model, transversal and longitudinal sections of the newly synthesized collagen fibers (C) by the active porcine fibroblasts were visible (d). Bars = 200 nm.
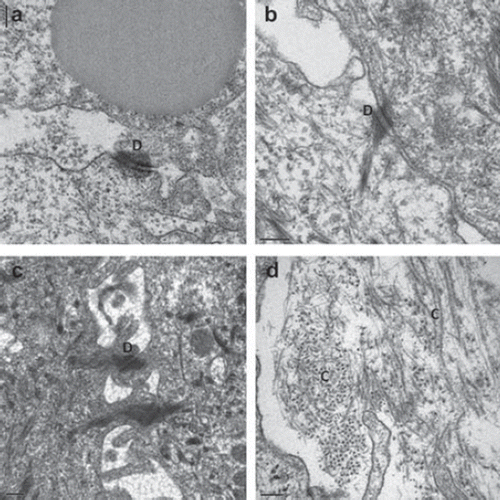
Conclusions
In this study, we demonstrated the feasibility of reconstructing several porcine oral mucosa models, including 3D full-thickness oral mucosa equivalents and epithelial models with or without support, to be transplanted in pigs prior to clinical trials. The epithelia in the three models were pluristratified, expressing the major differentiation marker keratin 13 of non-keratinized oral mucosa, and the fibroblasts of the 3D model were shown to be functional, synthesizing new extracellular matrix components such as collagen type I. The presence of fibroblasts was shown to significantly increase the thickness of the reconstructed porcine epithelium.
Acknowledgements
This study was supported by Hospices Civils de Lyon and Institut de Biologie et Chimie des Protéines (IBCP).
Declaration of interest
The authors declare no conflict of interest. The authors alone are responsible for the content and writing of the paper.
References
- Alberts B, Johnson A, Lewis J, Raff M, Roberts K, Walter P. 2002. Molecular biology of the cell. New York: Garland Science.
- Athanasiou KA, Almarzar A, Detamore M, Kalpakci K. 2009. Tissue engineering of temporomandibular joint cartilage. Morgan & Claypool Publishers.
- Collins P, Laffoon J, Squier CA. 1981. Comparative study of porcine oral epithelium. J Dent Res 60:543.
- Collombel C, Damour O, Gagnieu C, Marichy J, Poinsignon F. 1987. Biomaterials with a base of collagen, chitosan and glycosaminoglycans; Process for preparing them and their application in human medicine. French patent 8708252, 1987; European patent 884101948, 1988; US patent PCT/FR/8800303, 1989.
- Feinberg SE, Aghaloo TL, Cunningham Jr. LL. 2005. Role of tissue engineering in oral and maxillofacial reconstruction: Findings of the 2005 AAOMS Research Summit. J Oral Maxillofac Surg 63:1418–25.
- Garcia-Godoy F, Murray PE. 2006. Status and potential commercial impact of stem cell-based treatments on dental and craniofacial regeneration. Stem Cells Dev 15:881–7.Izumi K, Feinberg SE, Iida A, Yoshizawa M. 2003. Intraoral grafting of an ex vivo produced oral mucosa equivalent: a preliminary report. Int J Oral Maxillofac Surg 32:188–97.
- Izumi K, Song J, Feinberg SE. 2004. Development of a tissue-engineered human oral mucosa: From the bench to the bed side. Cells Tissues Organs 176:134–52.
- Kinikoglu B, Auxenfans C, Pierrillas P, Justin V, Breton P, Burillon C, Hasirci V, Damour O. 2009. Reconstruction of a full-thickness collagen-based human oral mucosal equivalent. Biomaterials 30:6418–25.
- Kinikoglu B, Rodríguez-Cabello JC, Damour O, Hasirci V. 2011. The influence of elastin-like recombinant polymer on the self-renewing potential of a 3D tissue equivalent derived from human lamina propria fibroblasts and oral epithelial cells. Biomaterials 32:5756–64.
- Kinikoglu B, Rovere MR, Haftek M, Hasirci V, Damour O. 2011. Influence of the mesenchymal cell source on oral epithelial development. J Tissue Eng Regen Med 2011 May 5.
- Lunney JK. 2007. Advances in swine biomedical model genomics. Int J Biol Sci 3:179–84.
- Ophof R, Maltha JC, Kuijpers-Jagtman AM, Von den Hoff JW. 2008. Implantation of tissue-engineered mucosal substitutes in the dog palate. Eur J Orthod 30:1–9.
- Ouellet G, Dubé J, Gauvin R, Laterreur V, Bouhout S, Bolduc S. 2011. Production of an optimized tissue-engineered pig connective tissue for the reconstruction of the urinary tract. Tissue Eng Part A 17:1625–33.
- Runswick SK, O'Hare MJ, Jones L, Streuli CH, Garrod DR. 2001. Desmosomal adhesion regulates epithelial morphogenesis and cell positioning. Nature Cell Biology 3:823–30.
- Squier CA, Cox P, Wertz PW. 1991. Lipid content and water permeability of skin and oral mucosa. J Invest Dermatol 96:123–6.
- Ueda M, Tohnai I, Nakai H. 2001. Tissue engineering research in oral implant surgery. Artif Organs 25:164–71.