Abstract
In this paper, a novel amperometric choline biosensor with immobilization of choline oxidase on electrochemically polymerized polypyrrole–polyvinylsulphonate (PPy–PVS) film has been accomplished via the entrapment technique. The effects of pH and temperature were investigated and optimum parameters were found to be 9.0 and 60 °C, respectively. There are two linear parts in the region between 1.0 × 10 −7 − 1.0 × 10 −6M (R2 = 0.997) and 1.0 × 10 −5 − 1.0 × 10 −3 M (R2 = 0.986). The storage stability and operation stability of the enzyme electrode were also studied.
Introduction
Choline is an amino alcohol with a very important function in the human organism. Choline can be found in several different fields as a main component in many plants and animal organs, e.g. bile, brain, egg yolks, hops, belladonna, strophantus, as an essential nutrient in human breast milk, or as a constituent of different biological fluids, e.g. amniotic liquid and blood. Choline is a hydrolytic product of acetylcholine (acetylcholine esterase) and phosphatidylcholine (phospholipase D). Phosphatidylcholine (PC) is a major component of cellular plasma membrane (Serradilla et al. Citation2003).
Choline deficiency can affect memory and easily cause liver dysfunction or cancer. Abnormal choline metabolism has been implicated in a number of neurodegenerative disorders such as Alzheimer's and Parkinson's diseases (Zhanxia et al. Citation2010, Michel et al. Citation2006, Zeisel Citation2004).
Thus, choline detection and determination is a basic problem for food and clinical analyses. Generally, analytical methods used up to date are based on determination of hydrogen peroxide liberated as a result of the enzymatic oxidation of choline by choline oxidase (ChO) (Langer et al. Citation2004).
Instrumental techniques and analytical procedures applied (e.g., HPLC, GC, spectrophotometry, chemiluminescence) are well known (Marazuela and Moreno-Bondi Citation1998, Tsafack et al. Citation2000); however, most of them are expensive and time-consuming. Conducting polymer sensors and particularly polyaniline-based biosensors of a more general use have also been reported (Trojanowicz et al. Citation1997, Hoa et al. Citation1992); e.g., for potentiometric analysis (Karyakin et al. Citation1996), electrochemical studies (Erdogdu and Karagozler Citation1997, Iwuoha et al. Citation1997), and some applications such as pesticide determination with immobilized cholinesterase (Ivanov et al. Citation2002).
In this paper, we report the immobilization of choline oxidase onto Ppy–Pvs film via an entrapment procedure for determination of choline. Effects of the immobilization process on kinetic parameters, storage, and operation stability of the enzyme were investigated. The optimum working conditions with respect to the substrate concentrations, the pH, and temperature were investigated.
Material and methods
Equipment and reagents
The electrochemical studies were carried out using Epsilon EC electrochemical analyzer using a three-electrode cell. The working electrode was a Pt plate (0.5 cm2). The auxiliary and the reference electrodes were Pt wire and Ag/AgCl electrode (3 M KCl), respectively. The pH values of the buffer solutions are measured with an ORION Model 720A pH/ion meter. Temperature control was achieved with Grant LT DGG thermostat.
Choline oxidase (EC 1.1.3.17, purified from the Alcaligenes species, activity of 12.03 unit/ml) and choline were purchased from Sigma. Pyrrole and sodium polyvinylsulphonate (Pvs) were supplied by Fluka and from Aldrich, respectively. All other chemicals were obtained from Sigma. All the solutions were prepared with the use of distilled water.
Preparation of choline biosensor
The surface of the Pt plate electrode was cleaned (Gros et al. Citation2000). It was covered with polypyrrole by the electropolymerization of pyrrole (Chaubey et al. Citation2000). Immobilization of choline oxidase was carried out by the physical entrapment technique. The Pt plate electrode was immersed in a 10mL solution of 0.1M pyrrole and 2.5 mL (25%) of sodium polyvinylsulphonate and 1 mL of choline oxidase (12.03 mg mL−1). The solution was purged with argon in order to remove the oxygen. The electropolymerization of pyrrole was performed upon the electrode surface by the cyclic voltammetric scans between 1.0 and + 2.0 V at a scan rate of 100 mV/s (vs Ag/AgCl electrode (3 M KCl)) and 4 cycles (). After entrapping the choline oxidase onto the Ppy-Pvs film, the electrode was rinsed with deionized water to remove the unreacted pyrrole monomer and free choline oxidase. Immobilized enzyme electrode was kept in a refrigerator at the 4 °C in phosphate buffer when not in use.
Amperometric measurements
The Pt/Ppy-Pvs electrode was immersed into the phosphate buffer (0.1 M) of pH 9.0. The solution contained 0.1 M sodium perchlorate as a supporting electrolyte. The electrode was brought to equilibrium by keeping it at 0.3 V (vs. Ag/AgCl electrode (3 M KCl)). Steady-state current (ia) was recorded. Choline was added to the cell from stock solution and the system was stirred. The currents (ib) obtained at 0.3 V were recorded. The current values (Δi = ib−ia) were plotted against the choline concentration in order to determine the working range of the electrode.
Results and discussion
In this study, we prepared a new choline biosensor with the entrapment of choline oxidase on polypyrrole-polyvinylsulphonate (PPy-Pvs) composite film. The parameters affecting the performance of the biosensor were investigated.
The determination of working potential
After preparing Pt/Ppy-Pvs electrodes, the hydrogen peroxide oxidation was carried out at different potentials (0.1, 0.2, 0.3, 0.4, 0.5, 0.6, 0.7 V). Current differences of H2O2 (0.1 mM) in different potentials (0.1, 0.2, 0.3, 0.4, 0.5, 0.6, 0.7 V) were measured by using Pt/PPy–Pvs electrode and plotted against potential in . When is examined, it is seen that oxidation currents of H2O2 are too low and close to each other at 0.1 V and 0.2 V. Potential increases from 0.3 V to the increasing currents. At higher potentials, interferences were caused by exogenous substances present in body fluids (e.g., ascorbic acid) (Zhang et al. Citation2007). Therefore 0.3 V was used as working potential.
The effect of pH on amperometric response of biosensors
Buffers solutions (0.1 M phosphate buffer) at various pH values were tested to investigate the effect of pH. The pH values of the buffer solutions varied between 6.0 and 10. The measurements were performed at a constant choline concentration of 5.0 × 10 −5 M. shows that the maximum response was obtained at pH 9.0. For the choline biosensor, pH values different than 9.0 were employed in the literature (pH 7.4; 8.0) (Serradilla et al. Citation2003, Langer et al. Citation2004). This was attributed to the fact that the used polymer and the type of immobilization were different.
The effect of temperature
The amperometric response of the prepared biosensor was investigated at different temperatures using constant choline concentration of 5.0 × 10 −5 M (). As seen from the figure, the current difference increases with temperature up to 60 °C and decreases afterwards. The highest current difference was obtained at 60 °C. The enzyme was thought to be denaturized after this temperature. The study was carried out at 25 °C due to the difficulties involved in working at 60 °C. For the choline biosensor, temperature values different than 60 °C were employed in the literature (37, 30 °C) (Serradilla et al. Citation2003, Langer et al. Citation2004). This was attributed to the fact that the used polymer and the type of immobilization were different.
Substrate concentration and calibration curves
In order to determine the linear working range of the choline biosensor, the current values obtained for solutions containing 1.0 × 10 −7 − 1.0 × 10 −3 M choline were recorded (). There are two linear parts in the region up to 1.0 × 10 −3 M ranging between 1.0 × 10 −7 − 1.0 × 10 −6 M (R2 = 0.997) () and 1.0 × 10 −5 − 1.0 × 10 −3 M (R2 = 0.986) (). The graph for the lower concentration range is given in . It has been shown that the linearity of this graph is highly satisfactory and it can be used for the quantitative determination of choline. The low detection limit of the biosensor was found to be 1.0 × 10 −7 M and the response time of the biosensor was 200 s. The KM(app) constant, a specific parameter of enzymes, was found out through the use of 1/[choline])-1/Δi graph (Lineweaver–Burke plot, ). KM(app) and Imax values were found to be 5.58 × 10 −4 mM and 0.4648 μA/min, respectively. The KM(app) value that shows the affinity of the biosensor was 5.58 × 10 −4 mM and the values cited in the literature were 0.4 mM, 2.32 mM, and 0.083 mM (Langer et al. Citation2004, Gülce et al. Citation2003, Shi et al. Citation2006). Since the enzymatic reactions take place through a carrier medium, the affinity of the enzyme towards the substrate is not the same in every carrier media. Therefore it is normal that KM(app) values differ.
The operational stability of the choline biosensor
In order to test the operational stability of the enzyme electrode prepared, the current changes obtained after subsequent usage were plotted against the number of measurements. The relative standard deviation obtained after19 measurements at a constant choline concentration of 5.0 × 10 −5 M was found to be 6% ().
The storage stabilization of the enzyme electrode
The response of the enzyme electrode prepared under optimum conditions was measured for a period of 42 days at constant choline concentration (5.0 × 10 −5 M). Results of nine measurements during this period are plotted in . There was no noticeable decrease in the response during the first seven days. There was a rapid decrease in current values between the seventh and tenth days and there was not a significant change after the thirty-fifth day. The electrode showed 35% of the initial amperometric response at the end of the forty-second day. This indicates that the biosensor can be used for quite a long time. The properties and the optimum working conditions of the biosensor prepared are summarized in .
Figure 10. Storage stability of the biosensor (in the phosphate buffer (pH 7.5), in operating potential 0.3 V, 25 °C).
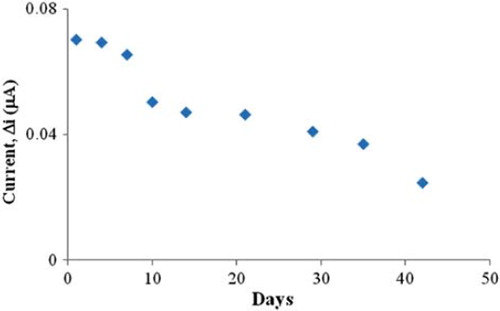
Table I. The properties and the optimum working conditions of the biosensor.
Interference effect
A few common substances found in serum were studied for any interfering effect on the analysis of choline. Known concentration of uric acid, ascorbic acid, glucose, paracetamol (acetaminophen), alanine, sistein, sistin, and dopamine were added to 1.0 × 10 −4 M choline solutions and results are presented in . When is examined, it can be seen that interference effects of paracatemol and uric acid were the most. These interferences can almost be removed by dilution of solution in cell.
Table II. Interfering effects of substances and the removal of interfering effects were investigated
In conclusion, the choline biosensor prepared in this study:
Is useable in a large concentration range (1.0 × 10 −7 − 4.0 × 10 −6 M (R2 = 0.997) and 1.0 × 10 −5 − 1.0 × 10 −3 M (R2 = 0.986).
Has a very low detection limit (1.0 × 10 −7 M).
Has an acceptable response time for a biosensor (200 s).
Gives good reproducible results (the relative standard deviation is 6% after 19 measurements, the standard deviation obtained).
Has satisfactory storage stabilization (gives 35% of the initial amperometric response at the end of the forty-second day).
The Km(aap) and Imax(aap) values of choline oxidase enzyme immobilized in polypyrrole-polyvinylsulphonate (Ppy–Pvs) composite film were 5.58×10−4 mM and 0.4648 μ/min, respectively.
Is easy to prepare and highly cost-effective.
Declaration of interest
The authors report no conflicts of interest. The authors alone are responsible for the content and writing of the paper.
References
- Chaubey A, Gerard M, Singhal M, Singh VS, Malhotra BD. 2000. Immobilization of lactate dehydrogenase on electrochemically prepared polypyrrole–polyvinylsulphonate composite films for application to lactate biosensors. Electrochim Acta. 46(5):723–729.
- Erdogdu G, Karagozler AE. 1997. Investigation and comparison of the electrochemical behavior of some organic and biological molecules at various conducting polymer electrodes. Talanta 44: 2011–2018.
- Gros P, Durliat H, and Comtat M. 2000. Use of polypyrrole film containing Fe(CN)63 − as pseudo-reference electrode: Application for amperometric biosensors. Electrochim. Acta. 46:643–650.
- Gülce H, Aktas YS, Gülce A, Yıldız A. 2003. Polyvinylferrocenium immobilized enzyme electrode for choline analysis. Enzyme and Microbial Technology 32:895–899.
- Hoa DT, Suresh Kumar TN, Punekar NS, Srinivasa RS, Lal R, Contractor AQ. 1992. A biosensor based on conducting polymers. Anal. Chem. 64:2645–2646.
- Ivanov AN, Lukachova LV, Evtugyn GA, Karayakina EE, Kiseleva SG, Budnikov HC, Orlov AV, Karpacheva GP, Karyakin AA. 2002. Polyaniline-modified cholinesterase sensor for pesticide determination. Bioelectrochemistry 55:75–77.
- Iwuoha EI, Saenz de Villaverde D, Garcia NP, Smyth MR, Pingarron JM. 1997. Reactivities of organic phase biosensors. 2. The amperometric behaviour of horseradish peroxidase immobilised on a platinum electrode modified with an electrosynthetic polyaniline film. Biosens. Bioelectron. 12:749–761.
- Karyakin AA, Bobrova OA, Lukachova LV, Karyakina EE. 1996. Potentiometric biosensors based on polyaniline semiconductor films. Sens. Actuators B 33:34–38.
- Langer JJ, Filipiak M, Kecin'ska J, Jasnowska J, Włodarczak J, Buładowski B. 2004. Polyaniline biosensor for choline determination. Surface Science 573:140–145.
- Marazuela MD, Moreno-Bondi MC. 1998. Determination of choline-containing phospholipids in serum with a fiber-optic biosensor. Anal. Chim. Acta. 374:19–29.
- Michel V, Yuan Z, Ramsubir S, Bakovic M. 2006. Choline transport for phospholipid synthesis. Exp. Biol. Med. 231:490–504.
- Serradilla Razola S, Pochet S, Grosfils K, Kauffmann JM. 2003. Amperometric determination of choline released from rat submandibular gland acinar cells using a choline oxidase biosensor Biosensors and Bioelectronics 18:185–191.
- Shi H, Yang Y, Huang J, Zhao Z, Xu X, Anzai J-I, Osa T, Chen Q. 2006. Amperometric choline biosensors prepared by layer-by-layer deposition of choline oxidase on the Prussian blue-modified platinum electrode. Talanta 70:852–858.
- Trojanowicz M, Krawczynski T, Alexander PW. 1997. Organic conducting polymers as active materials in electrochemical chemo-sensors and biosensors. Chemia Analityczna 42(2):199–213.
- Tsafack VC, Marquette CA, Pizzolato F, Blum L. 2000. Chemiluminescent choline biosensor using histidine-modified peroxidase immobilised on metal-chelate substituted beads and choline oxidase immobilised on anion-exchanger beads co-entrapped in a photocrosslinkable polymer. Biosens. Bioelectron. 15:125–133.
- Zeisel SH. 2004. Nutritional importance of choline for brain development. J. Am. Coll. Nutr. 23(6):621S–626S.
- Zhang Y, Wen G, Zhou Y, Shuang S, Dong C, Choi MMF. 2007. Development and analytical application of an uric acid biosensor using an uricase-immobilized eggshell membrane. Biosens. Bioelectron. 22:1791–1797.
- Zhanxia Zhang, Juan Wang, Xiaolei Wang, Yong Wang, Xiurong Yang. 2010. A sensitive choline biosensor with supramolecular architecture. Talanta 82:483–487.