Abstract
Objective: To evaluate whether garlicin can attenuate reperfusion no-reflow in a catheter-based porcine model of acute myocardial infarction (AMI). Methods: Twenty-two swine were used: six in a sham-operation group, and eight each in the control and garlicin groups. The distal part of the left anterior descending coronary artery (LAD) in the latter two groups was occluded by a dilated balloon for 2 hr, then reperfused for 3 hr. Garlicin (1.88mg/kg) was injected just before reperfusion until reperfusion for 1 hr in the garlicin group. Hemodynamic data were examined before AMI, 2 hr after occlusion, and 3 hr after reperfusion. Myocardial contrast echocardiography (MCE) and pathological staining were performed to evaluate the myocardial no-reflow area (NRA). Serum proinflammatory cytokines and endothelin (ET)-1 were examined by radioimmunoassay. Results: Left ventricular systolic pressure (LVSP) and left ventricular end-diastolic pressure (LVEDP) significantly improved in the garlicin group after reperfusion compared with the control group and also 2hr after AMI (p<0.05 for both). MCE and pathological staining both showed garlicin attenuated reperfusion NRA after AMI (p<0.05, p<0.01). Garlicin not only decreased serum interleukin (IL)-6 and tumor necrosis factor (TNF)-α after reperfusion (p<0.05 for both), but also ET-1 level (p<0.01). Conclusion: Garlicin attenuated reperfusion no-reflow in our catheter-based porcrine model of AMI, possibly through decreasing serum proinflammatory cytokines and ET-1.
Coronary reperfusion therapy, such as catheter-based percutaneous coronary intervention (PCI), is widely performed in patients with acute myocardial infarction (AMI). However, patency of the infarct-related artery does not always guarantee salvage of myocardium at risk of ischemia. The concept of “no-reflow” refers to a state of myocardial tissue hypoperfusion in the presence of a patent epicardial coronary artery. “Reperfusion no-reflow” occurs after primary PCI for reperfusion of an infarct-related artery in AMI, which may be asymptomatic or present with continued chest pain and ST-segment elevation. Reperfusion no-reflow is preceded by ischemic cell injury, which is confined to the irreversibly damaged necrotic zone, and may be exacerbated at the time of reperfusion (Jaffe et al. Citation2008), which is an independent predictor of adverse clinical outcome after AMI, regardless of infarct size, and is associated with heart failure and increased mortality (Morishiima et al. 2000).
Garlicin is a kind of chemical compound extracted from garlic corn. Many recently published reports show that garlic possesses plasma lipid-lowering, anticoagulant, and antioxidant properties and improves impaired endothelial function (Yin et al. Citation2002, Banerjee et al. Citation2002, Saravanan and Prakash Citation2004, Gorinstein et al. Citation2007). To our knowledge, little is known about the preventive potential of garlicin on reperfusion no-reflow after AMI. The aim of the present study is to evaluate whether garlicin can attenuate reperfusion no-reflow in a catheter-based porcine model of AMI. Diallyl trisulfide, one main garlic constituent, was used in our study.
Materials and methods
AMI models and animal grouping
All animal experiments were approved by the Animal Care and Use Committee of China-Japan Friendship Hospital, Beijing, China, and were in compliance with the “Guide for the Care and Use of Laboratory Animals” published by the US National Institute of Health (Publication No. 85-23, revised in 1996). Twenty-two male Chinese mini swines, 22±3kg, from China Agricultural University were randomized into three groups: six in the sham-operation group, and eight each in the control group and garlicin group. Our porcine model of AMI was modified on the basis of the previous study by Suzuki et al. (Suzuki et al. Citation2008). The mini-swines were intubated under general anesthesia using 30mg diazepam, 0.03g/ml pentobarbital sodium, 30ml and 4mg pipecuronium and ventilated. Animals were placed in the right anterior oblique position and had continuous electrocardiograph (ECG) (limb and precordial leads) and hemodynamic monitoring throughout all procedures. Vascular access was obtained using a 6 Fr vascular sheath, placed in the right femoral artery. All animals received preprocedural heparin 6000u. After advancing a 6 Fr guiding catheter (Judkins left type) through the ascending aorta into the coronary ostia, baseline coronary angiography (CAG) was performed to identify the subsequent location of the occlusion and coronary artery size. The distal part (about 1/2-1/3 site) of left anterior descending coronary artery (LAD) was completely occluded by dilated balloon (2.0mm×10mm)for 2 hr and successful AMI model was confirmed by CAG and ECG. Then LAD was reperfused for 3 hr and CAG was performed again to ensure LAD with TIMI 3 blood flow. In sham-operation group, balloon was just placed in LAD without dilatation. There was no AMI, reperfusion or no-reflow. Garlicin (Shanghai Harvest Pharmaceutical) at a dosage of 1.88mg/kg body weight was injected just before reperfusion until reperfusion for 1 hr in the garlicin group. Saline was used in the control group.
Hemodynamic assessment
To assess serial cardiac function, the left ventricular systolic pressure (LVSP), left ventricular end-diastolic pressure (LVEDP), and heart rate (HR) were obtained by the catheter method at three time points: (1) before AMI; (2) 2 hr after occlusion; and (3) 3 hr after reperfusion.
Myocardial contrast echocardiography
Real-time myocardial contrast echocardiography (MCE) and trigger MCE were performed by an investigator, blind to treatment allocation at three time points: (1) before AMI; (2) 2 hr after occlusion; (3) 3 hr after reperfusion. Short-axis images of the left ventricle at the papillary muscle level were obtained with an echocardiographic system (Philips iE33) equipped with a transducer (S5-1). Real-time MCE was performed by an intravenous injection of SONOVUE (25mg in 5ml saline, Bracco), and the mechanical index was 0.1. We drew 0.05 ml/kg of SONOVUE into a 30-ml syringe and gave a bolus injection from the femoral vein in 1 min followed by a 10-ml saline flush. After uniform development of the left ventricle, flash triggering (flash mechanical index 1.19, flash frame 8) was performed and dynamic images of at least 20 cardiac cycles were obtained continuously for analysis. In trigger MCE, images were obtained with the fundamental mode and triggering on the R wave of the ECG. We used a mechanical index of 1.02 and a trigger interval of 8-10 cardiac cycles. Dynamic images were recorded when the right ventricle developed. The tracing method was used with Q-lab software to detect the myocardial filling defect area and left ventricular area. These measurements were performed three times continuously at R wave in the clearest section. The filling defect areas 2 hr after occlusion and 3 hr after reperfusion were defined as risk area (RA) and no-reflow area (NRA), respectively. NRA/RA was calculated.
Pathological staining
Double-staining with 0.01g/ml Evans blue dye and 0.04g/ml thioflavin-S was performed to delineate RA and NRA. Three hours after reperfusion, 1 ml/kg thioflavin-S was injected into the left ventricle. The reperfused region was stained, while the no-reflow region was not stained. After another complete occlusion of LAD by a dilated balloon, Evans blue was injected into the left ventricle and normal myocardium was stained. Animals were then sacrificed with an intravenous bolus injection of potassium chloride after staining. The hearts were then excised and the left ventricles were sectioned into a 10-mm-thick cross-sectional myocardial slice. The cross-sectional myocardial slice at the papillary muscle level was chosen. In room light, normal myocardium, reperfused myocardium, and NRA were stained blue, yellow, and unstained, respectively. Sections similar to MCE were photographed. RA, NRA, and left ventricular wall area (LVWA) were measured using an image processing software IPP 6.0. RA/LVWA and NRA/LVWA were calculated.
Serum IL-6, TNF-α, and ET-1 level by radioimmunoassay
To assess serum interleukin (IL)-6, tumor necrosis factor (TNF)-α, and endothelin (ET)-1 by radioimmunoassay, blood was obtained from the femoral vein at five time points: (1) before AMI; (2) 2 hr after occlusion; (3) 1 hr after reperfusion; (4) 2 hr after reperfusion; (5) 3 hr after reperfusion. For radioimmunoassay, serum samples were stored at -80 °C until final processing. Concentrations of IL-6, TNF-α, and ET-1 were determined by 125I radioimmunoassay kits (East Asia Radioimmunoassay Center of Chinese PLA General Hospital) according to the manuals. The detection limits were all 1500pg/ml.
Statistical analysis
Data are expressed as means±SD. Statistical analysis was carried out by analysis of variance (ANOVA) followed by appropriate post-hoc tests, including multiple comparison tests (LSD). All analyses were made using an SPSS 11.5 statistical software package and probability values of less than 0.05 were considered statistically significant.
Results
Procedure success and mortality
Twenty-six male Chinese mini swines were used and four died during the procedure. Causes of death included: 1) laryngeal edema by intubation failure; 2) too deep anesthesia; 3) thrombosis in left main coronary artery due to coronary injury by excessive balloon inflation; and 4) recurrent ventricular tachycardia and fibrillation after reperfusion. Six swines were in a sham-operation group and eight were in two other groups each. Except in the six swine of the sham-operation group, a complete occlusion of the left anterior descending coronary artery(LAD) by dilating ballon was confirmed by CAG, and CAG was performed again to confirm LAD with TIMI 3 blood flow after the balloon deflated in the 16 swine of the other two groups, which successfully simulated reperfusion after AMI.
Effect of garlicin on hemodynamics
Hemodynamic parameters were similar in each group before AMI, as shown in . LVSP decreased significantly in the control group 2hr after AMI and 3 hr after reperfusion, compared with that before AMI (p<0.05 for both), while LVEDP was elevated significantly (p<0.05 for both). HR, LVSP, and LVEDP were similar in the control and garlicin group 2 hr after AMI, while LVSP and LVEDP significantly improved in the garlicin group after reperfusion compared with the group (p<0.05) and 2 hr after AMI (p<0.05).
Table I. Hemodynamics at different time points of each group.
MCE
Myocardial perfusion at the left ventricular short-axis papillary muscle level in different groups was evaluated by real-time MCE and trigger MCE. There were no differences between the left ventricular area (LVA) measured by real-time MCE and that by the trigger MCE 2 hr after LAD occlusion and 3 hr after reperfusion (p>0.05 for both), indicating that the sections selected at the same level were of good comparability. The correlation coefficient of trigger MCE and real-time MCE was 0.864, which consistently reflected the effect of garlicin on myocardial repferfusion and no-reflow. As shown in , RA/LVA was similar in the control and garlicin groups when calculated by the two methods (p>0.05). NRA/RA by trigger MCE and real-time MCE was 35.3% and 45.1%, respectively, in the garlicin group, which both reached statistical significance (p<0.05, respectively) compared with the model group (58.2% and 63.5%).
Table II. MCE results assessing RA and NRA.
Pathological staining
Three hours after reperfusion, normal myocardium, reperfusion area, and NRA could be recognized by double-staining with Evans blue dye and thioflavin-S. Normal myocardium, reperfusion area, and no-reflow areas were dark blue, yellow, and dark red, respectively, in room light and black, bright yellow, and deep red, respectively, in the UV light (as shown in ). As shown in , RA/LVWA was 49.93% and 50.62% in the control and garlicin groups, respectively, and no significant differences were found between the two groups. NRA/RA in the garlicin group was 24.32%, significantly lower than 46.85% in the control group (P<0.01).
Figure 1. Excised heart stained with Evans blue dye and thioflavin-S. Normal myocardium, reperfusion area, and NRA were dark blue, yellow, and dark red, respectively, in room light (A,C,E), while black, bright yellow, and deep red, respectively, in UV light (B,D,F). A,B: sham-operation group; C,D: control group; E,F: garlicin group.
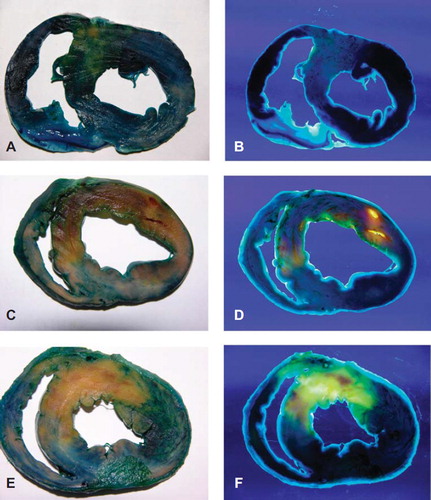
Table III. Pathological staining assessing RA and NRA.
Garlicin decreased serum IL-6, TNF-α, and ET-1 after myocardial reperfusion
By radioimmunoassay, serum IL-6 and TNF-α didn't change significantly at different time points in the sham-operation group. They were also similar in each group 5 min before occlusion. As shown in and , serum IL-6 and TNF-α increased significantly 2 hr after occlusion, reperfusion 1 hr, reperfusion 2 hr, and reperfusion 3 hr compared with that before occlusion in the control group (P < 0.05 for all). In the garlicin group, IL-6 and TNF-α) increased significantly 2 hr after occlusion compared with that before occlusion (P < 0.05), while IL-6 decreased 1 hr, 2 hr, and 3 hr after reperfusion compared with 2 hr after occlusion (P < 0.05 for all). TNF-α increased further after reperfusion in the garlicin group, while they were still lower than those of the control group (P < 0.05 for all). Garlicin decreased serum IL-6 and TNF-α level after myocardial reperfusion.
Table IV. Serum IL-6 at different time of each group(pg/ml)
Table V. Serum TNF-α at different time of each group(ng/ml).
As shown in , serum ET-1 didn't change significantly at different time points in the sham-operation group. They were also similar in each group 5 min before occlusion. Serum ET-1 increased significantly 2 hr after occlusion and after reperfusion compared with that before occlusion in both the model and garlicin group (P < 0.05 respectively). However, serum ET-1 level in the garlicin group at reperfusion 1 hr, reperfusion 2 hr, and reperfusion 3 hr was still lower than the model group (P < 0.01 respectively).
Table VI. Serum ET-1 at different time of each group (pg/ml)
Discussion
AMI animal models using dogs or pigs are often developed by coronary ligation after surgical thoracotomy. However, left ventricular remodeling of this open-chest model is influenced by opening both the chest and pericardium. Resultant trauma may also cause infection, unsuccessful recovery from anesthesia, and increased mortality. An intracoronary balloon inflation technique can allow the control of both the location and duration of coronary artery occlusion, which has been reported in previous studies (Krombach et al. 2005). Our porcine model of AMI was modified on the basis of a previous study (Suzuki et al. Citation2008). In this model, selective catheterization and placement of the balloon catheter at the desired site of LAD can be achieved within 10 min. In addition, the duration of occlusion can be controlled exactly because the LAD can be reperfused immediately after balloon deflation.
In our study, LVSP decreased significantly in the control group 2hr after AMI and 3hr after reperfusion, compared with that before AMI, while LVEDP elevated significantly. When LAD occluded, systolic function decreased in ischemic myocardium commonly with a lower LVSP; at the same time, cardiac diastolic function also decreased, showing a higher LVEDP. After reperfusion, systolic and diastolic function of ischemic myocardium were restored to some extent, while reperfusion no reflow might happen. Myocardial ischemia-reperfusion injury and endothelial damage underlie the development of reperfusion no reflow. Infarct size and microvascular hypoperfusion may increase at the time of coronary reperfusion beyond that observed during the ischemic period. Endothelial injury is induced by an acute inflammatory response, generation of reactive oxygen species, intracellular calcium overload, and opening of the mitochondrial permeability transition pore (Jaffe et al. Citation2008).
LVSP and LVEDP were significantly improved in the garlicin group after reperfusion compared with the control group and 2hr after AMI. Real-time and trigger MCE here can both delineate myocardial NRA and RA, which demonstrated garlicin decreased NRA after reperfusion in AMI, though the specific values of NRA by the two methods were not exactly the same. Our study provided evidence of the potential benefit of garlicin at the time of reperfusion in reducing no-reflow produced by 2 hr ischemia followed by 3 hr reperfusion.
Although experimental models and clinical trials have established that prompt, effective, and sustained restoration of blood flow after coronary occlusion can limit infarct size, improve ventricular function, and enhance survival, reperfusion triggers an active inflammatory response associated with an intense neutrophilic infiltration of ischemic myocardium that may produce further tissue injury. Garlicin has antioxidant and anti-inflammatory properties, which can reduce oxidative stress, increase eNOS activity, and also attenuate VCAM-1 expression (Vazquez-Prieto et al. Citation2011). It's also reported that garlicin controls TNF-α-mediated inflammation and vascular disease (Hui et al. Citation2010). In our study, garlicin suppressed the production of serum IL-6 and TNF-α after myocardial reperfusion. Inhibiting TNF-α and IL-6 mediated inflammation may be an important cardioprotective mechanism of garlicin.
ET-1 is one of the most potent endogenous vasoconstrictors and mediates a host of responses, including endothelial dysfunction, vasomotor contraction, leukocyte activation, and cellular proliferation and high levels of ET-1 impair endothelial nitric oxide production (Ramzy et al. Citation2006). ET-1 release and subsequent calcium overload are important mediators of reperfusion injury during myocardial infarction (Tamareille et al. Citation2008). Interestingly, garlicin also inhibited the release of ET-1 and ET-1 antagonism may provide a novel therapeutic strategy to improve vascular homeostasis in ischemia/reperfusion injury.
Garlicin attenuated reperfusion no-reflow in our catheter-based porcine model of AMI, possibly through decreasing serum proinflammatory cytokines and ET-1.
Acknowledgments
This work was partly supported by the Capital Medical Development Research Fund of China (03III02).
Declaration of interest
The authors report no conflicts of interest. The authors alone are responsible for the content and writing of the paper.
References
- Banerjee SK, Dinda AK, Manchanda SC, Maulik SK. 2002. Chronic garlic administration protects rat heart against oxidative stress induced by ischemic reperfusion injury. BMC Pharmacology 2:16.
- Gorinstein S, Jastrzebski Z, Namiesnik J, Leontowicz H, Leontowicz M, Trakhtenberg S. 2007. The atherosclerotic heart disease and protecting properties of garlic: contemporary data. Molecular Nutrition & Food Research 51:1365–1381.
- Hui C, Like W, Yan F, Tian X, Qiuyan W, Lifeng H. 2010. S-allyl-L-cysteine sulfoxide inhibits tumor necrosis factor-alpha induced monocyte adhesion and intercellular cell adhesion molecule-1 expression in human umbilical vein endothelial cells. Anat Rec (Hoboken) 293:421–430.
- Jaffe R, Charron T, Puley G, Dick A, Strauss BH. 2008. Microvascular obstruction and the no-reflow phenomenon after percutaneous coronary intervention. Circulation 117:3152–3156.
- Morishima I, Sone T, Okumura K, Tsuboi H, Kondo J, Mukawa H, . 2000. Angiographic no-reflow phenomenon as a predictor of adverse long-term outcome in patients treated with percutaneous transluminal coronary angioplasty for first acute myocardial infarction. Journal of the American College of Cardiology 36: 1202–1209.
- Ramzy D, Rao V, Tumiati LC, Xu N, Sheshgiri R, Miriuka S, . 2006. Elevated endothelin-1 levels impair nitric oxide homeostasis through a PKC-dependent pathway. Circulation 114:I319–326.
- Saravanan G, Prakash J. 2004. Effect of garlic (Allium sativum) on lipid peroxidation in experimental myocardial infarction in rats. Journal of Ethnopharmacology 94:155–158.
- Suzuki Y, Lyons JK, Yeung AC, Ikeno F. 2008. In vivo porcine model of reperfused myocardial infarction: In situ double staining to measure precise infarct area/area at risk. Catheter Cardiovasc Interv. 71:100–107.
- Tamareille S, Achour H, Amirian J, Felli P, Bick RJ, Poindexter B, . 2008. Left ventricular unloading before reperfusion reduces endothelin-1 release and calcium overload in porcine myocardial infarction. The Journal of Thoracic and Cardiovascular Surgery 136:343–351.
- Vazquez-Prieto MA, Rodriguez Lanzi C, Lembo C, Galmarini CR, Miatello RM. 2011. Garlic and onion attenuates vascular inflammation and oxidative stress in fructose-fed rats. Journal of Nutrition and Metabolism 2011:475216.
- Yin MC, Hwang SW, Chan KC. 2002. Nonenzymatic antioxidant activity of four organosulfur compounds derived from garlic. Journal of Agricultural and Food Chemistry 50:6143–6147.