Abstract
Optical vorticesare generated and controlled to form trapping tools in the same way as optical tweezers. By using the intense optical vortices generated within the PANDA ring resonator, the required atoms/molecules can be trapped and moved (transported) dynamically within the wavelength router or network. The advantage of the proposed system is that a transmitter and receiver can be formed within the same system, which is available for atoms/molecules storage and transportation based on methods that have been proposed to deliver drugs into cells for specific diagnosis.
Introduction
Optical tweezers have become one of the primary weapons in the arsenal of biophysicists and high-resolution biomolecules (Fazal and Block Citation2011, Hwang and Park Citation2011). The ability of optical tweezers to trap and manipulate biomolecules (Fazal and Block Citation2011, Ashok and Dholakia Citation2011),for chemical analysis (Fan and White Citation2011), and to study physics and chemistry began to develop in science, engineering, and medicine for study at the molecular level (Fazal and Block Citation2011, Fan and White Citation2011). Cordovez et al. (Citation2011) have shown the selection of fluidic delivery fromisolated microchannels through an electrochemically drivenpumping reaction and movement of the fluid toward an implantable microfluidic drug delivery device. Polymer micro-needles are of interest for implantable drug delivery due to their enhanced biocompatibility. The tools used by the optofluidics community to enable this control included micro- and nanofluidic channels and photonic elements such as waveguides, optical resonators, optical fibers, and lasers (Erickson et al. Citation2011). The combination of microfluidic trapping of technologies devices with microparticle arrays is a promising lab-on-a-chip tool reviewed by Derveaux et al. (Citation2008). To the combination of optical, fluidics, and electrical manipulation technologies, an alternative new techniquehas emergedcalled optoelectrofluidics, whichrefers to the motions of particles or fluids under an electric field, which is induced or perturbed by an optical source (Jalil et al. Citation2011). In medicine, the application of nanotechnology,a single red blood cell (Mohanty, #11) deformability testhas been performed by optical trapping plastic in a microfluidics chip (Lee et al. Citation2005) and lab-on-a-chip for RBC transportation in a capillary network to circulate oxygen and carbon dioxide throughout the human body (Chen et al. Citation2010). Optical trapping for manipulation of molecules in liquid core capillaries and its application to drug delivery has been reported by Suwanpayak et al. (Citation2011a), who used a PANDA ring resonator to form, transmit, and receive the microscopic volume (of drug) by controlling the ring parameters. A microscopic volume can be trapped and moved dynamically within the wavelength router or network.
Recently, promising techniques of biomoleculetrapping (Yang et al. Citation2009) and transporting within the add/drop multiplexer have been reported theoretically (Jalil et al. Citation2011, Piyatamrong et al. Citation2010) and experimentally (Cai and Poon Citation2010, Bellini et al. Citation2010), in which the transporter is an optical tweezer. Static tweezers are now well recognized and used. Dynamic tweezers are now also used in practical work (Egashira et al. Citation1998, Kachynski et al. Citation2003, Oguchi and Terada Citation2010). Schulzyzet al. (Citation2007) have shown that the transfer of trapped atoms between two optical potentials could be performed. Optical tweezers use the forces exerted by intensity gradients in the strongly focused beams of light to trap and move microscopic volumes of matter; the trap volume is thus much larger than the particle sizes. Other combinations of forces are induced by the interaction between photons, caused by photon scattering effects. In practice, the field intensity can be adjusted and tuned to produce the desired gradient field and scattering force and thus produce a suitable trapping force. Hence, the appropriate force can be configured for the transmitter/receiver parts.
Dynamic optical tweezers/vortices are generated using theGaussian pulse propagating within anadd/drop optical multiplexer incorporated with two nanoring resonators (PANDA ring resonator). The dynamic behavior of soliton and Gaussian pulse is well described by Kachynski et al. (Citation2003). The PANDA ring resonator is a specific device whose name was given by the authors. The design of the original structure of a PANDA ring and its application for drug delivery was reported by Oguchi and Terada (Citation2010). Tumors are similar to organs, with unique physiology giving rise to an unusual set of transport barriers to drug delivery. Cancer and tumor therapy are limited by non-uniform drug delivery via blood vessels, thetransportation of drug via blood into regions of the tumor, and distribution to the region of the tumor cell by a vascular network (Bauer and Nadler Citation2006, Lee et al. Citation2010, Chakraborty and Tsuchiya Citation2008); convective drug transport from blood vessels into and throughout most of the tumor tissueisa major issue that needs to be resolved (Lee et al. Citation2010).
Today, chemotherapy drugs are used to process delivery to alter the behavior of tumor cells. However, the side-effects of chemotherapy kill growing cells and normal cells, too. In the proposed system, optical waveguides are designed sothe drug atoms and molecules can be trapped and transported (filter) through a microneedle (Chauhan et al. Citation2011), in which molecules can be moved and transported to a specific target such as a tumor, tumor cancer, or abnormal cells. The atoms/molecules of the drug can be stored and transported in the hollow liquid core waveguide using add/drop optical vortices the same as liquid core microneedles (Chakraborty and Tsuchiya Citation2008). The drug types have been controlled/released into the target by a single ring resonator. In this study, the use of two different-wavelength tweezers, molecular buffers, and bus networks to transport drug volume, especially for direct delivering and transporting large volumes of drug, has been investigated. In addition, two different-wavelength tweezersare fed into the tumor cellvia molecular network stability. In practice, the multi-drug delivery networkscan be used for large-scale drug delivery, i.e. large-volume diagnosis.
Principles and Method
In principle, trapping forces are exerted by the intensity gradients of highly focused light beams to trap and transport the microscopic volumes of matter. The optical forces are customarily defined by the relationship between optical scattering force and gradient force (Fgrad) (Svoboda and Block Citation1994). Furthermore, in the Rayleigh regime, the trapping forces decompose naturally into two components, since the electromagnetic field is uniform across the dielectric. Thus, the particles can be treated as induced point dipoles. Increasing the numerical aperture (NA) increases the gradient strength due to a decrease in focal spot size, which can be formed within the tiny system, for instance a nanoscale device (nanoring resonator). In this proposed system as shown in , the trapping force is produced by a dark soliton, in which the valley of the dark soliton is generated and controlled within the PANDA ring resonator by the control port signals. shows the optical potentialwell at the through port and normalized of drop port. Conventional optical tweezerswere formed at the diffraction-limited focus of a laser beam; they have become a powerful and flexible tool for manipulating micrometer-sized objects, as reviewed by Juan et al. (Citation2011). In the add/drop device, the nonlinear refractive index is ignored because it does not affect the system. In this work, the proposed ring resonator, called a PANDA ring, is a modified add-drop filter. By using the broad-spectrum output, the multitweezers can be generated and molecules can be trapped and transported.
Figure 1. Schematic diagram of molecular buffer array: (a) PANDA ring resonator; (b) atomic array and network; (c) diagram of optical trapping forces are formed by scattering force and gradient force; (d) molecular buffer array in hollow liquid corewaveguides.
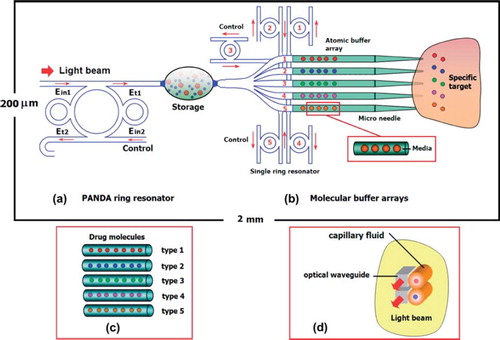
The power output (Pt1) at the through port is written as
The power output (Pt2) at the drop port is
The optical trapping devices in show that the drug molecules can be transported and the moving process using optical vortices generated via PANDA ring resonator (Suwanpayak et al. Citation2011a), in which the trapping forces are exerted by the intensity gradients of highly focused light beams to trap and transport the microscopic volumes of matter. The devices are designed for direct delivery of drug molecules to anti-specific target disease such as tumor cancers.
In the design system, optical vortices (optical potential wells) and optical forces are generated by the PANDA ring resonator, as shown in and as the optical dynamic tweezers with different center wavelengths are generated. The biomolecules and drug particle can be transported and moved on the waveguide surface (liquid capillaries) into the tumor target, in which the distributed drug types are different around the tumor cells that can be controlled/released via the control filter port (Ein2) and add/drop ring resonator number 1–5, where drugs are released at different times.
Figure 2. Results of the trapping tools: (a) wavelengths center 400 nm; (b) 450 nm; (c) wavelengths center 500 nm and 600 nm; (d) the normalized of wavelengths center 500 nm and 600 nm, Rad = 2 μm, RR = RL = 1 μm. The coupling coefficients are fixed κ0 = 0.95, κ1 = 0.5, κ3 = 0. 5; κ2 are varied at 0.2, 0.3, 0.4; the input power is 1 W.
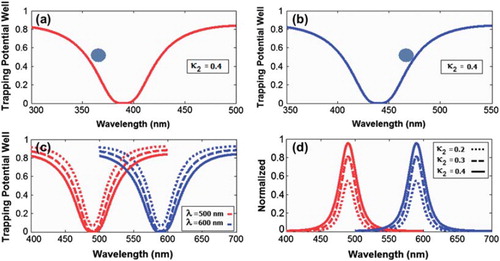
Figure 3. Results of the trapping tools at wavelengths center 500 nm and 650 nm; the coupling coefficients are given as κ0 = 0.5, κ1 = 0.35, κ2 = 0.1, and κ3 = 0.35, respectively. The ring radii are Radd = 2 μm, RR = RL = 1 μm, respectively.
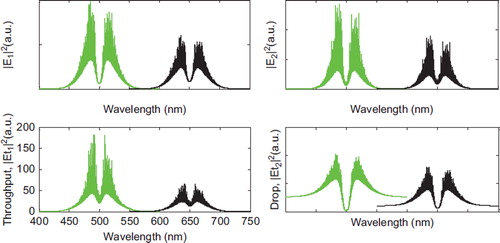
A molecular buffer needs to be included in the system. The molecular buffer plays an important role for storing or delaying atoms/molecules over a period of time, which gives enough time for operation (Macleod Citation2010, Karp Citation2010). A molecular buffer is a new device (Suwanpayak et al. Citation2011b). In simulation, the light beam with center wavelength 400 nm and 450 nm, peak power 1 W, and pulse width of 35 fs is fed into the system via the input port as shown in , where the coupling coefficients are κ0 = 0.95, κ1 = 0.5, κ3 = 0.5, and κ2 are varied as 0.2, 0.3, 0.4. The ring radii are Radd = 2 μm, RR = RL = 1 μm. The evidence for the practical device with a radius of 2–3 μm has been reported by Zhu et al. (Citation2010). In this case, the dynamic tweezers (gradient fields) are in the form of visible light and Gaussian pulses, which can be used to trap the required microscopic volume. In this investigation four tweezers with different center wavelengths are generated.In , there are two different center wavelengths of tweezers, where the dynamical movements are (a) |E1|2, |E2|2, through port and drop port signals. In this case, the trapped drug molecules are transported and moved by the drop port, in this case the tunable trapping can be obtained. The important aspect of this system is that the tunable tweezers can be obtained by tuning (controlling)the add (control) port input signal.
The advantage of the proposed system is that the transmitter and receiver can fabricate on-chip and alternatively can be operated by a single device. The magnitude of optical trapping force is in thepico Newton (pN) range, depending on the relative refractive index of the particle (Takada et al. Citation2002). The particle radius located in the cavity decreases with the decrease in refractive indices compared with the host medium (Sarapat et al. Citation2009, Smit Citation2005). The waveguide of the drug delivery system can be an optical waveguide with a liquid core, which can trap the drug molecules smoothly within the micro-needle network. The advantage of the proposed system is that it provides multiple access to the drug volumes and targets. Moreover, the use of mesh networks (combined networks) can also be realized, which can offer a large diagnosis area.
Conclusions
Trapped drug molecules can move into the liquid core waveguide and networks using optical tweezers, in which the drug can be trapped, stored, and delivered via the atomic micro-needle on a chip. Such a system can also be used for a large-scale molecular drug delivery network and diagnosis. The drug particles can be trapped and transported within the micro-needle and network, such as a nanocarrier using practical device parametersand the proposed system can be fabricated and integrated into a practical thin film device. The trapping and movement of molecules in the system can be used for certain diagnostic purposes and to deliver small molecules to target organs in the human body. The proposed system can be used for long-distance transport of drug molecules in multi-drug delivery networks, in which drug delivery or molecular communication can be performed via the wavelength router and bus network, which will be available for large volume diagnosis in the near future. The power sourcesare used for thesystem andimplantable devicescurrently being developedoffer the possibility of enzyme-free glucose fuel cells without a filter membrane between the electrodes, as demonstrated by Oncescu and Erickson (Citation2011).
Acknowledgments
We would like to thank the Institute of Advanced Photonics Science, Nanotechnology Research Alliance, UniversitiTeknologi Malaysia (UTM), and King Mongkut's Institute of Technology (KMITL), Thailand, for providing the research facilities. This research work has been supported by UTM's Tier 1/Flagship Research Grant, MyBrain15 Fellowship/MOHE SLAB Fellowship, and the Ministry of Higher Education (MOHE) research grant. N. Suwanpayak would like to acknowledge King Mongkut's Institute of Technology Ladkrabang, Bangkok (KMITL), Thailand, for partial support in higher education at KMITL, Thailand.
Declaration of interest
The authors report no conflicts of interest. The authors alone are responsible for the content and writing of the paper.
References
- Ashok PC, Dholakia K. 2011. Optical trapping for analytical biotechnology.Current OpinBiotechnol.23:1–6.
- Bauer WR, Nadler W. 2006. Molecular transport through channels and pores: Effects of in-channel interactions and blocking. PNAS 103(31):11446–51.
- Bellini N, Vishnubhatla KC, Bragheri F, Ferrara L, Minzioni P, Ramponi R, . 2010.Femtosecond laser fabricated monolithic chip for optical trapping and stretching of single cells. Opt Express. 18(5):4679–88.
- Cai H, Poon A. 2010. Optical manipulation and transport of microparticle on silicon nitride microring resonator-based add-drop devices.Opt Lett.35:2855–>57.
- Chakraborty S, Tsuchiya K. 2008. Development and fluidic simulation of microneedles for painless pathological interfacing with living systems. J Appl Phys. 103:11470.
- Chauhan VP, Stylianopoulos T, Boucher Y, Jain RK. 2011. Delivery of molecular and nanoscale medicine to tumors: Transport barriers and strategies. Annu Rev ChemBiomol Eng. 2:281–98.
- Chen YC, Chen GY, Lin YC, Wang GJ. 2010. A lab-on-a-chip capillary network for red blood cell hydrodynamics. Microfluid Nanofluid. 9:585–91.
- Cordovez B, Chung AJ, Mak M, Erickson D. 2011. A novel polymer microneedle fabrication process for active fluidic delivery. Microfluid Nanofluid. 10:785–91.
- Derveaux S, Stubbe B, Braeckmans K, Roelant C, Sato K, Demeester J, .2008. Synergism between particle-based multiplexing and microfluidics technologies may bring diagnostics closer to the patient. Anal. Bioanal. Chem. 391(7):2453–67.
- Egashira K, Terasaki A, Kondow T. 1998. Photon-trap spectroscopy applied to molecules adsorbed on a solid surface: Probing with a standing wave versus a propagating wave. App Opt. 80:5113–15.
- Erickson D, Sinton D, Psaltis D.2011. Optofluidics for energy applications. Nat Photon. 5(10):583–90.
- Fan X, White IM. 2011. Optofluidic microsystems for chemical and biological analysis. Nat Photon. 5(10):591–7.
- Fazal FM, Block SM. 2011. Optical tweezers study life under tension. Nat Photon. 5(6):318–21.
- Hwang H, Park JK. 2011. Optoelectrofluidic platforms for chemistry and biology. Lab Chip. 11(1):33–47.
- Jalil MA, Suwanpayak N, Kulsirirat K, Suttirak S, Ali J, Yupapin PP. 2011. Embedded nanomicro syringe on chip for molecular therapy. Int J Nanomed. 6:2925–32.
- Juan ML, Righini M, Quidant R. 2011. Plasmon nano-optical tweezers. Nat Photon. 5(6):349–56.
- Kachynski AV, Kuzmin AN, Pudavar HE, Kaputa DS, Cartwright AN, Prasad PN.2003. Measurement of optical trapping forces by use of the two-photon-excited fluorescence of microspheres. Opt Lett. 28:2288–90.
- Karp G. 2010. Cell and molecular biology, 6th ed. Hoboken, NJ: John Wiley & Sons, Inc.
- Lee WG, Park K, Bang H, . 2005. Single red blood cell defromnility test using optical trapping in plastic microfluid chip. Proceedings of the 31 Annual International IEEE EMBS Special Topic on Conference Microtechnologies in Medicine and Biology, Kahuku, Oahu, Hawaii; 12–15 May 2005:389–90.
- Lee JH, Yigit MV, Mazumdar D, Lua Y. 2010. Molecular diagnostic and drug delivery agents based on aptamer-nanomaterial conjugates. Advanced Drug Delivery Reviews. 62: 592–605.
- Macleod HA.2010. Thin-film optical filter, 4th ed. New York: Taylor & Francis.
- Oguchi K, Terada S. 2010. Optical network system, optical router, fault recovery method of optical network, and program. JP 2010063009 (patent).
- Oncescu V, Erickson D. 2011.A microfabricated low cost enzyme-free glucose fuel cell for powering low-power implantable devices. J Power Source. 196 (22):9169–75.
- Piyatamrong B, Kulsirirat K, Mitatha S, Yupapin PP. 2010. Dynamic potential well generation and control using double resonators incorporating in an add/drop filter. Mod PhysLett B. 24:3071–82.
- Sarapat K, Ali J, Yupapin PP. 2009. A novel storage and tunable light source generated by a soliton pulse in a micro ring resonator system for super dense wavelength division multiplexing use. Microw Opt TechnolLett. 51:2948–52.
- Schulzyz M, Crepaz H, Schmidt-Kaler F, Eschner J, Blatt R. 2007. Transfer of trapped atoms between two optical tweezer potentials. J Mod Opt. 54:1619–26.
- Smit MK. 2005. Progress in AWG design and technology. Proceedings of WFOP 2005. 4th IEEE/LEOS workshop on fibres and optical passive component, p. 26. Mondello, Italy.
- Suwanpayak N, Jalil MA, Teeka C, Ali J, Yupapin PP. 2011a. Optical vortices generated by a PANDA ring resonator for drug trapping and delivery applications. Bio Med Opt Express. 2:159–68.
- Suwanpayak N, Jalil MA, Aziz MS, Ali J, Yupapin PP. 2011b. Molecular buffer using a PANDA ring resonator for drug delivery use. Int J Nanomed. 2011;6:575–80.
- Svoboda K, Block SM. 1994. Biological applications of optical forces.Ann Rev Biophy Biomol Struct. 23:247–82.
- Takada K, Abe M, Shibata T, Okamoto K. 2002. Field demonstration of over 1000-channel DWDM transmission with supercontinuum multi-carrier source. Electron Lett. 38:572–73.
- Yang AHJ, Moore SD, Schmidt BS, Klug M, Lipson M, Erickson D. 2009.Optical manipulation of nanoparticles and biomolecules in sub-wavelength slot waveguides. Nature 457:71–75.
- Zhu J, Ozdemir SK, Xiao YF, Li L, He L, Chen DR. 2010. On-chip single nanoparticle detection and sizing by mode splitting in an ultrahigh-Qmicroresonator. Nat Photonics. 4:46–49.