Abstract
Carbazole substituted imines (2a-l) were prepared from N-methyl-3-amino carbazole with different aldehydes. The imines compounds undergo (2+2) cycloaddition reactions with in situ ketenes to produce β-lactam compounds (3a-l). The β-lactam compounds were tested as inhibitors of the xanthine oxidase (XO) purified from bovine milk. The results show that these compounds exhibit inhibitory effects on XO at low concentrations with IC50 values ranging from 21.65 to 58.04 µM. The most effective compound for XO was 4-(4-chlorophenyl)-1-(9-ethyl-9H-carbazol-3-yl)-3-phenylazetidin-2-one with IC50 of 21.65 μM. The lactams investigated here showed effective XO inhibitory effects, in the same range as the clinically used allopurinol.
Introduction
Enzyme inhibitors can interact with enzymes and block their activity towards natural substrates. The importance of enzyme inhibitors as drugs is enormous since these molecules have been used for treating a number of pathophysiological conditions Banner and Hadvary Citation1991, Sandstrom Citation1989). Xanthine oxidase (XO; EC 1.2.3.22) is situated at the end of a catabolic sequence of the purine nucleotide metabolism in humans and a few other uricotelic species. Its major function is to catalyze the oxidation of hypoxanthine to xanthine and that of xanthine to uric acid (Harris et al. Citation1999). XO is widely distributed throughout various organs including the liver, gut, lung, kidney, heart, and brain, as well as the plasma (Sathisha et al. Citation2011). Xanthine oxidase inhibitors (XOI) are very useful, since they possess fewer side-effects compared to uricosuric and anti-inflammatory agents. Allopurinol (1H-pyrazolo [3,4-d] pyrimidin-4-ol) is a substrate and a specific potent inhibitor for XO, and has been used for gout treatment for a number of years. The synthetic analogues of allopurinol, pyrazolopyrimidine, 1-phenylpyrazoles, and cytokines are also used as XO inhibitors (Tamta et al. Citation2005, Ishibuchi et al. Citation2001). Inhibition mechanism and molecular modeling studies of these analogues have also been conducted by scientists (Sheu et al. Citation1997, Tamta et al. Citation2006). Recently, several studies have indicated that allopurinol may induce hypersensitivity syndrome and Stevens–Johnson syndrome in patients (Hammer et al. Citation2001, Bashir et al. Citation2000, Hsieh et al. Citation2007).
The β-lactam structures known from penicillin and cephalosporin have been well developed as miracle drugs for the therapy of bacterial infectious diseases in clinics (Georg and Ravikumar Citation1992). Apart from clinical use as antibacterial agents, these compounds serve as synthons in the synthesis of other biological active heterocyclic (Ojima Citation1995), and in many examples the biological activity can be interpreted as an interaction between the β-lactam and a serine-containing enzyme (serine protease) like elastase (Clemente et al. Citation2001, Elriati et al. Citation2008). Therefore, the search for clinically useful β-lactams is a growing field of interest. In this study, we describe a number of carbazole groups containing β-lactams and study their properties as inhibitors of XO purified from bovine milk.
Experimental
Instruments
Melting points of the compounds were measured using an Electro thermal 9100 apparatus. 1H and 13C NMR spectra were recorded using a Varian 300 MHz Mercury Plus instrument using TMS as internal standards.
Materials
Sepharose 4B, L-tyrosine, benzamidine, protein assay reagents, and chemicals for electrophoresis were obtained from Sigma Chem. Co. All other chemicals obtained from Sigma and Merck were used without further purification.
Enzyme purification
Fresh bovine milk, without added preservatives, was cooled down to 4 °C overnight. EDTA and toluene were then added to give final concentrations of 2 mM and 3% (v/v), respectively. The milk was churned with a blender at top speed for 30 min at room temperature. During this time, the temperature rose from 4 to 45 °C. After cooling the churned milk to about 4 °C, the curning process was repeated and the sample was filtered. This sample was brought to 38% saturation by addition of solid ammonium sulphate (Özer et al. Citation1999). The suspension was centrifuged at 15000 rpm for 30 min and the precipitate formed was discarded. The supernatant was brought to 50% saturation with solid ammonium sulphate. The precipitate formed was collected by centrifugation at 15000 rpm for 60 min and dissolved in 0,1 M Tris-HCl, pH =7,6. The affinity column containing sepharose-4B- L-tyrosine-p-amino benzamidine was equilibrated in 0. 1 M glycine, 0. 1 M NaCl, pH =9. 0 (Beyaztaş and Arslan Citation2011). The sample was applied to the affinity gel, washed with 0, 1 M glycine, pH =9. XO, and then eluted with 25 mM benzamidine in 0. 1 M glycine, 0.1 M NaCl, pH =9.0 (McManaman et al. Citation1996). Fractions of 1.5 mL were collected and their absorbance measured at 280 nm.
Activity measurements
Xanthine oxidase activity was determined at 37 °C by the modified method of Massey et al. (Citation1969). The conversion of xanthine to uric acid was followed by monitoring the change in absorbance at 295 nm, using a CARY 1E, UV-Visible Spectrophotometer-VARIAN spectrometer (ε292 =9.5 mM21 cm21). The reaction mixture contained 50 mM Tris-HCl, pH =7.6, and 0.15 mM xanthine, at 37 °C. The assay was initiated by the addition of the enzyme. One unit of enzyme activity was defined as the amount of enzyme that converts one μmol of xanthine to uric acid per min under defined conditions (Özer et al. Citation1999).
In vitro inhibition kinetic studies
For the inhibition studies of some β–lactams derivatives, different concentrations were added to the enzyme activity. XO enzyme activity with β-lactams derivatives was assayed by following the oxidation of xanthine. Activity % values of xanthine oxidase for six different concentrations of β-lactams derivatives were determined by regression analysis using Microsoft Office 2000 Excel. Xanthine oxidase activity without a β-lactam was accepted as 100% active. The graphs determined that the inhibitor concentration caused up to 50% inhibition (IC50 values) on the enzyme (Isik et al. Citation2009).
Total protein determination
Quantitative protein determination was achieved by absorbance measurements at 595 nm according to the Bradford (Citation1976) method, with bovine serum albumin as a standard.
Sodium dodecyl sulphate-polyacrylamide gel electrophoresis
Sodium Dodecyl Sulphate (SDS) polyacrilamide gel electrophoresis was performed after having a purified enzyme. It was carried out in 10% and 3% acrylamide-bisacrylamide concentrations for the running and stacking gel, respectively, containing 0.1% SDS according to the Laemmli (Citation1970) method. The sample was applied to the electrophoresis medium. Gel was stained overnight in 0.1% Coomassie Brilliant Blue R- 250 in 50% methanol and 10% acetic acid, and then destained by frequently changing the same solvent, without using dye.
General procedure for the synthesis of imines.14.26 mmol of 3-amino-9-ethylcarbazole were dissolved in ethanol (30 ml) and added to 14.26 mmol Na2CO3 and 14.26 mmol aldehydes derivates. The reaction mixture was then refluxed for 24 hours at 90 °C. The solution was extracted with AcOEt with an aqueous phase. The organic phase was washed three times with water, dried with anhydrous magnesium sulphate, and evaporated under reduced pressure.
N-benzylidene-9-ethyl-9H-carbazol-3-amine (2a, C21H18N2).Yield was 96%, recrystallized from ether/hexane to give light-yellow crystals. M.p.: 96.5 °C; 1H NMR (300 MHz, CDCl3); 8.65 (1H,s), 8.12-8.10 (1H,d), 8.10-8.09 (1H,d), 8.03-8.02 (1H,d), 7.97-7.96 (1H,d), 7.95-7.94 (1H,t), 7.50-7.48 (1H,t), 7.47-7.46 (1H,t), 7.46-7.45 (2H,d), 7.41 (1H,s), 7.23-7.21 (2H,t), 4.37-4.32 (2Hq), 1.46-1.38 (3H,t); 13C NMR (75 MHz, CDCl3): 158.33, 144.03, 140.76, 139.05, 136.94, 131.12, 129.00(2C), 128.81(2C), 126.13, 123.71, 123.31, 120.83, 120.23, 119.12, 112.78, 109.00, 108.91, 37.95, 14.12.
N-(4-methoxybenzylidene)-9-ethyl-9H-carbazol-3-amine (2d, C22H20N2O).Yield was 92% recrystallized from dichloromethane/hexane to give as dark-yellow crystals. M.p.: 118.4 °C; 1H NMR (300 MHz, CDCl3) 8.57 (1H,s); 8.12-8.11 (1H,d), 8.09 (1H,s), 7.91-7.89 (1H,d), 7.46-7.44 (1H,t), 7.43-7.40 (2H,d), 7.38-7.37 (1H,d), 7.25-7.24 (1H,d), 7.22-7.20 (1H,t), 7.01-6.98 (2H,d), 4.39-4.32 (2H,q), 3.86 (3H,s), 1.46-1.43 (3H,t); 13C NMR (75 MHz, CDCl3): 162.11, 157.87, 144.40, 140.73, 138.81, 130.46 (2C), 129.99, 126.05, 123.70, 123.32, 120.80, 120.22, 119.03, 114.41 (2C), 112.55, 108.96, 108.87, 55.66, 37.93, 14.12.
N-(3-methoxybenzylidene)-9-ethyl-9H-carbazol-3-amine (2e, C22H20N2O).Yield was 50%, recrystallized from ether/hexane to give light-brown crystals. M.p.: 99.8 °C; 1H NMR (300 MHz, CDCl3): 8.66 (1H,s), 8.17-8.15 (1H,d), 8.08 (1H,s), 7.65-7.64 (1H,d), 7.54-7.52 (1H,d), 7.52-7.51 (1H,t), 7.50 (1H,s), 7.49-7.44 (1H,t), 7.44-7.43 (1H,d), 7.42-7.40 (1H,d), 7.31-7.28 (1H,t), 7.09-7.05 (1H,d), 4.39-4.32 (2H,q), 3.93 (3H,s), 1.48-1.43 (3H,t); 13C NMR (75 MHz, CDCl3): 160.28, 158.16, 143.91, 140.78, 139.10, 138.44, 129.99, 126.19, 123.74, 123.34, 122.40, 120.87, 120.27, 119.19, 118.08, 112.92, 111.86, 109.06, 108.98, 55.71, 37.94, 14.16.
N-(3-chlorobenzylidene)-9-ethyl-9H-carbazol-3-amine (2g, C21H17ClN2).Yield was 99%, recrystallized from ether/dichloromethane/hexane to give yellow crystals. M.p.: 102.5 °C; 1H NMR (300 MHz, CDCl3): 8.62 (1H,s), 8.14-8.13 (1H,d), 8.05-8.04 (1Hd), 8.00-7.79 (1H,d), 7.79-7.78 (1H,d), 7.51-7.50 (1H,t), 7.49-7.48 (1H,t), 7.48-7.47 (1H,d), 7.43 (1H,s), 7.43-7.41 (1H,d), 7.26-7.23 (1H,t), 7.25 (1H,s), 4.39-4.35 (2H,q), 1.48-1.43 (3H,t); 13C NMR (75 MHz, CDCl3): 156.25, 143.33, 140.77, 139.28, 138.75, 135.18, 130.94, 130.22, 128.30, 127.12, 126.23, 123.73, 123.26, 120.84, 120.21, 119.24, 112.99, 109.06, 108.96, 37.97, 14.12.
N-(3-nitrobenzylidene)-9-ethyl-9H-carbazol-3-amine (2i, C21H17N3O2). Yield was 98%, recrystallized from ether/dichloromethane/hexane to give orange crystals. M.p.: 144.2 °C; 1H NMR (300 MHz, CDCl3): 8.76 (1H,s), 8.74 (1H,s), 8.27-8.25 (1H,d), 8.25-8.24 (1H,d), 8.09-8.06 (1H,d), 7.64-7.61 (1H,t), 7.53-7.51 (1H,t), 7.51-7.49 (1H,d), 7.48-7.46 (1H,d), 7.42 (1H,s), 7.27-7.25 (1H,d), 7.25-7.22 (1H,t), 4.39-4.32 (2H,q), 1.46-1.40 (3H,t); 13C NMR (75 MHz, CDCl3): 154.35, 148.91, 142.59, 140.81, 139.57, 138.69, 133.98, 129.90, 126.36, 125.15, 123.76, 123.39, 123.24, 120.84, 120.26, 119.38, 113.29, 109.14, 109.04, 37.98, 14.11.
N-(2-nitrobenzylidene)-9-ethyl-9H-carbazol-3-amine (2j, C21H17N3O2). Yield was 90%, recrystallized from ether/dichloromethane/hexane to give red crystals. M.p.: 135.4 °C; 1 HNMR (300 MHz, CDCl3): 9.13 (1H,s), 8.41-8.39 (1H,d), 8.15-8.12 (1H,d), 8.11 (1H,s), 8.06-8.03 (1H,d), 7.74-7.71 (1H,t), 7.57-7.56 (1Hd), 7.54-7.53 (1H,t), 7.50-7.48 (1H,d), 7.43-7.40 (1H,d), 7.30-7.27 (1H,t), 7.26-7.24 (1H,t), 4.394.32 (2H,q), 1.47-1.42 (3H,t); 13C NMR (75 MHz, CDCl3): 152.75, 149.42, 142.86, 140.80, 139.63, 133.71, 131.81, 130.82, 129.76, 126.33, 124.77, 123.74, 123.27, 120.93, 120.35, 119.39, 113.87, 109.13, 109.02, 37.96, 14.12.
N-(2-bromobenzylidene)-9-ethyl-9H-carbazol-3-amine (2k, C21H17BrN2). Yield was 80%, recrystallized from dichloromethane/hexane to give dark-green crystals. M.p.: 120.2 °C; 1 HNMR (300 MHz, CDCl3): 9.07 (1H,s), 8.35-8.32 (1Hd), 8.17-8.10 (1H,d), 8.09 (1H,s), 7.66-7.64 (1H,d), 7.56-7.53 (1H,t), 7.51-7.48 (1H,t), 7.48-7.45 (1H,d), 7.44-7.42 (1H,t), 7.31-7.28 (1H,t), 7.27-7.25 (1H,d), 4.39-4.34 (2H,q), 1.46-1.44 (3H,t); 13C NMR (75 MHz, CDCl3): 156.81, 143.63, 140.80, 139.35, 135.32, 133.44, 132.11, 129.09, 127.96, 126.23, 126.09, 123.77, 123.33, 120.92, 120.32, 119.25, 113.42, 109.06, 108.96, 37.97, 14.12.
N-(4-fluorobenzylidene)-9-ethyl-9H-carbazol-3-amine (2l, C21H17 FN2). Yield was 99%, recrystallized from methanol to give yellow-green crystals. M.p.: 120.4 °C; 1H NMR (300 MHz, CDCl3): 8.03-8.01 (1H,d), 7.48-7.46 (2H,d), 7.45 (1H,s), 7.45-7.44 (1H,t), 7.43-7.38 (1H,d), 7.37 (1H,s), 7.25-7.22 (1H,t), 7.21-7.19 (2H,d), 7.18-7.16 (1H,d), 6.95-6.92 (1H,d), 4.34-4.27 (2Hq), 1.43-1.38 (3H,t); 13C NMR (75 MHz, CDCl3): 140.64, 139.08, 134.79, 125.71 (2C), 123.89, 122.68, 120.69(2C), 118.27 (2C), 115.85 (2C), 109.27 (2C), 108.63 (2C), 106.65 (2C), 37.76, 14.11.
General procedure for the synthesis of β-lactams
A solution of Et3N in benzene was added drop-wise to a hot solution of imines and phenylacetylchlorid in benzene with continuous stirring. The reaction mixture was then refluxed at 85 °C for 24 hours. The solution was extracted with benzene with an aqueous phase. Organic phase was washed three times with water, dried with anhydrous magnesium sulphate, and evaporated under reduced pressure. 3a, 3b, 3d, 3e, and 3f were purified by recrystallization from acetone and other β-lactams were purified by column chromatography over silica gel using AcOEt/hexane as eluents followed by recrystallization from acetone.
1-(9-ethyl-9H-carbazol-3-yl)-3,4-diphenylazetidin-2-one (3a, C29H24N2O). Yield was 54%, giving light-yellow crystals. M.p.: 184 °C; 1H NMR (300 MHz, CDCl3): 8.16 (1H,s), 8.03-8.00 (1H,d), 7.50-7.48 (1H,d), 7.49-7.48 (1H,t), 7.47-7.45 (2H,t), 7.44-7.43 (1H,t), 7.41-7.40 (2H,d), 7.40-7.38 (2H,d), 7.37-7.37 (1H,d), 7.37-7.36 (2H,t), 7.36-7.35 (1H,d), 7.34-7.33 (1H,t), 7.21-7.19 (1H,t), 5.157-5.154 (1H,d), 4.346-4.321 (2H,q), 4.297-4.279 (1H,d), 1.607-1.601 (3H,t); 13C NMR (75 MHz, CDCl3): 165.45, 140.66, 138.11, 137.07, 135.34, 130.19, 129.53 (2C), 129.28 (2C), 128.85 (2C), 128.10 (2C), 127.82, 126.29 (2C), 123.22, 122.77, 121.01, 119.01, 116.17, 109.68, 108.94, 108.80, 65.24, 64.36, 37.82, 14.05.
1-(9-ethyl-9H-carbazol-3-yl)-3-phenyl-4-p-tolylazetidin-2-one (3b, C30H26N2O). Yield was 71%, giving white crystals. M.p.: 190.5 °C; 1 HNMR (300 MHz, CDCl3): 7.60 (1H,s), 7.46-7.44 (1H,d), 7.16-7.25 (1H,t), 6.95-6.94 (2H,d), 6.93-6.91 (1H,t), 6.9-6.90 (2H,d), 6.90-6.88 (2H,t), 6.87-6.85 (2H,d), 6.84-6.83 (1H,t), 6.81-6.80 (2H,d), 6.71-6.68 (1H,d), 4.60-4.59 (1H,d) (J =2.35), 3.83-3.80 (2H,q), 3.78-3.77 (1H,d), 1.83 (3H,s), 0.87-0.83 (3H,t); 13 CNMR (75 MHz, CDCl3): 164.92, 140.12, 138.16, 136.47, 134.93, 134.47, 129.75 (2C), 129.66, 128.86 (2C), 127.68 (2C), 127.38, 126.04 (2C), 125.94, 122.46, 122.04, 120.35, 118.67, 115.90, 109.07, 108.84, 108.67, 64.70, 63.41, 37.33, 21.05, 13.69.
1-(9-ethyl-9H-carbazol-3-yl)-3-phenyl-4-m-totylazetidin-2-one (3c, C30H26N2O). Yield was 82%, giving light-brown crystals. M.p.: 196.8 °C; 1H NMR (300 MHz, CDCl3): 8.14 (1H,s), 8.13,7.95 (1H,d), 7.50-7.48 (2H,t), 7.48-7.46 (1H,d), 7.42-7.41 (1H,t), 7.40-7.39 (2H,d), 7.37 (1H,s), 7.33-7.32 (1H,t), 7.30-7.28 (1H,t), 7.28-7.26 (1H,t), 7.24-7.21 (1H,d), 7.15-7.12 (1H,d), 7.11-7.10 (1H,d), 7.10-7.09 (1H,d), 5.29-5.28 (1H,d) (J =2.34), 4.37-4.36 (1H,d), 4.33-4.31 (2H,q), 2.26 (3H,s), 1.26-1.23 (3H,t); 13C NMR (75 MHz, CDCl3): 165.47, 140.66, 138.97, 138.49, 137.00, 135.77 (2C), 130.10, 129.81, 129.57, 129.52 (2C), 128.26 (2C), 127.49, 126.75, 124.20, 122.73, 122.35, 120.98, 119.34, 116.80, 110.09, 109.80, 109.76, 64.71, 63.14, 37.66, 21.74, 14.32.
1-(9-ethyl-9H-carbazol-3-yl)-4-(4-methoxyphenyl)-3-phenylazetidin-2-one (3d. C30H26N2O2). Yield was 36%, giving beige crystals. M.p.: 154.2 °C; 1H NMR (300 MHz, CDCl3): 8.15-8.15 (1H,s), 8.03-8.00 (1H,d), 7.51-7.50 (1H,d), 7.50-7.48 (1H,d), 7.48-7.47 (1H,t), 7.46-7.43 (2H,d), 7.41-7.39 (1H,t), 7.35-7.32 (2H,d), 7.31-7.26 (1H, t), 7.225-7.22 (1H,d), 7.19-7.17 (2H,t), 6.94-6.19 (2H,d), 5.03-5.02 (1H,d)(3J =2.35), 4.31-4.30 (1H,d), 4.34-4.27 (2H,q), 3.80 (3H,s), 1.41-1.36 (3H,t); 13C NMR (75 MHz, CDCl3): 165.64, 160.02, 140.62, 137.02, 135.42, 130.18, 129.90, 129.28 (2C), 128.05, 127.82 (2C), 127.60 (2C), 126.29, 123.17, 122.76, 121.03, 118.99, 116.20, 114.88 (2C), 109.72, 108.92, 108.81, 63.34, 64.10, 55.57, 37.82, 14.08.
1-(9-ethyl-9H-carbazol-3-yl)-4-(3-methoxyphenyl)-3-phenylazetidin-2-one (3e, C30H26N2O2). Yield was 41%,giving white crystals. M.p.: 197.2 °C; 1H NMR (300 MHz, CDCl3): 8.13 (1H,s), 8.12-8.02 (1H,d), 7.58-7.55 (1H,d), 7.46-7.43 (1H,t), 7.42-7.40 (1H,d), 7.40 (1H,s), 7.38-7.36 (1H,t), 7.35-7.34 (1H,d), 7.33-7.32 (1H,d), 7.31-7.28 (2H,t), 7.28-7.17 (1H,t), 7.15-7.13 (1H,t), 7.10-7.08 (2H,d), 6.90-6.87 (1H,d), 5.38-5.41 (1H,d)(3J =1.73), 4.41-4.43 (1H,d), 4.33-4.32 (2H,q), 3.65 (3H,s), 1.51-1.35 (3H,t); 13C NMR (75 MHz, CDCl3): 165.57, 160.35, 140.70, 140.24, 137.07, 135.81 (2C), 130.93, 129.98, 129.62 (2C), 128.33 (2C), 126.85, 122.68, 122.29, 121.04, 119.44, 119.27, 116.99, 114.36, 112.99, 110.33, 110.01, 109.91, 64.35, 62.84, 55.77, 37.66, 14.38
4-(4-chlorophenyl)-1-(9-ethyl-9H-carbazol-3-yl)-3-phenylazetidin-2-one (3f, C29H23ClN2O). Yield was 66%, giving white crystals. M.p.: 185.2 °C; 1H NMR (300 MHz, CDCl3): 8.125 (1H,s), 8.11-8.00 (1H,d), 7.46-7.44 (2H,d), 7.44-7.43 (2H,d), 7.42-7.41 (1H,t), 7.41-7.40 (1H,d), 7.40-7.39 (1H,t), 7.39-7.37 (2H,d), 7.37-7.36 (1H,t), 7.36-7.31 (1H,d), 7.28-7.22 (1H,d), 7.20-7.17 (2H,t), 5.05-5.04 (1H,d), 4.337-4.312 (2H,q), 4.288-4.280 (1H,d), 1.604-1.599 (3H,t); 13C NMR (75 MHz, CDCl3): 165.15, 140.68, 137.13, 136.64, 135.00, 134.66, 129.90, 129.78(2C), 129.35(2C), 128.24, 127.78(2C), 127.63 (2C), 126.40, 123.27, 122.69, 121.01, 119.09, 116.04, 109.63, 109.02, 208.85, 65.33, 63.70, 37.84, 14.04.
4-(3-chlorophenyl)-1-(9-ethyl-9H-carbazol-3-yl)-3-phenylazetidin-2-one (3g, C29H23ClN2O). Yield was 52% giving white powder. M.p.: 128.2 °C; 1 H NMR (300 MHz, CDCl3): 8.12 (1H,s), 8.11-8.12 (1H,d), 7.49-7.48 (2H,d), 7.48-7.46 (1H,t), 7.46-7.45 (1H,t), 7.44 (1H,s), 7.43,7.40 (2H,d), 7.39-7.37 (1H,d), 7.37 (1H,t), 7.35-7.32 (2H,t), 7.32-7.7.28 (1H,d), 7.28-7.26 (1H,d), 7.22-7.19 (1H,t), 5.03-5.02 (1H,d)(3J =3.51),4.36-4.29 (2H,q); 4.31-4.25 (1H,d); 1.51-1.35 (3H,t); 13 C NMR (75 MHz, CDCl3): 165.11, 140.65, 140.26, 137.13, 135.48, 134.88, 130.95, 129.83, 129.38(2C), 129.13(2C), 128.30, 127.81, 126.46, 126.39, 124.30, 123.24, 122.67, 121.05, 119.07, 116.01, 109.60, 109.05, 108.85, 65.26, 63.67, 37.85, 14.09.
1-(9-ethyl-9H-carbazol-3-yl)-4-(4-nitrophenyl)-3-phenylazetidin-2-one (3h, C29H23N3O3). Yield was 42%, giving orange crystals. M.p.: 125.8 °C; 1H NMR (300 MHz, CDCl3): 8.28-8.26 (2H,d), 8.09 (1H,s), 8.02-7.99 (1H,d), 7.64-7.61 (2H,d), 7.47,7.45 (1H,d), 7.44-7.42 (2H,d), 7.41-7.39 (1Hd), 7.39-7.38 (1H,t), 7.38-7.37 (1H,t), 7.36-7.29 (1H,d), 7.26-7.20 (2H,t), 7.19-7.17 (1H,t), 5.19-5.17 (1H,d)(3J =2.1), 4.39-4.35 (2H,q), 4.33-4.28 (1H,d), 1.54-1.38 (3H,t); 13 CNMR (75 MHz, CDCl3): 164.60, 148.26, 145.50, 140.70, 137.24, 134.42, 129.52 (2C), 128.58 (2C), 127.82 (2C), 127.14 (2C), 126.57 (2C), 124.93 (2C), 123.33, 122.55, 121.01, 119.20, 115.87, 109.53, 108.95, 65.35, 63.39, 37.88, 14.09.
1-(9-ethyl-9H-carbazol-3-yl)-4-(3-nitrophenyl)-3-phenylazetidin-2-one (3i, C29H23N3O3). Yield was 79%, giving yellow crystals. M.p.: 113.5 °C; 1H NMR (300 MHz, CDCl3): 8.34 (1H,s), 8.24-8.23 (1H,d), 8.07 (1H,s), 8.07-7.99 (1H,d), 7.82-7.79 (1H,d), 7.62-7.57 (1H,t), 7.48-7.47 (1H,d), 7.45-7.44 (1H,d), 7.42-7.40 (1H,t), 7.39-7.37 (1H,t), 7.36-7.30 (1H,d), 7.26-7.22 (2H,d), 7.19-7.17 (2H,t), 5.19-5.19 (1H,d)(3J =2.34), 4.33-4.32 (1H,d), 4.33-4.31 (2H,q), 4.29-4.28 (1H,d), 1.41-1.39 (3H,t); 13C NMR (75 MHz, CDCl3): 164.72, 149.12, 140.69, 140.49, 137.26, 134.42, 131.98, 130.82, 129.50(2C), 128.52(2C), 127.78(2C), 126.52, 123.95, 123.33, 121.59(2C), 121.00, 119.17, 116.01, 109.55, 109.20, 108.91, 65.40, 63.34, 37.86, 14.05.
1-(9-ethyl-9H-carbazol-3-yl)-4-(2-nitrophenyl)-3-phenylazetidin-2-one (3j, C29H23N3O3). Yield was 36% and intense yellow. M.p.: 214.4 °C; 1H NMR (300 MHz, CDCl3): 8.13 (1H,s), 8.00-8.06 (1H,d), 8.00-7.97 (1H,d), 7.59-7.56 (1H,d), 7.56-7.51 (1H,d), 7.51-7.48 (2H,d), 7.48-7.46 (2H,t), 7.46-7.40 (1H,d), 7.42-7.37 (1H,t), 7.34-7.28 (1H,t), 7.27-7.26 (1H,t), 7.26-7.25 (1H,d), 7.25-7.23 (1H,t), 6.30-6.28 (1H,d)(3J =6.11), 5.30-5.38 (1H,d), 4.33-4.31 (2H,q), 4.29-4.28 (1H,d), 1.41-1.39 (3H,t); 13 CNMR (75 MHz, CDCl3): 165.85, 147.48, 140.70, 137.23, 133.94, 132.38, 131.70, 130.16, 129.72, 129.63 (2C), 128.93(2C), 128.41, 127.88, 126.51, 125.67, 123.30, 122.63, 121.09, 119.16, 115.98, 109.31, 109.17, 108.92, 61.37, 59.00, 37.920, 14.12.
4-(2-bromophenyl)-1-(9-ethyl-9H-carbazol-3-yl)-3-phenylazetidin-2-one (3k, C29H23BrN2O). Yield was 88% and white crystals. M.p.: 221 °C; 1H NMR (300 MHz, CDCl3): 8.13 (1H,s), 8.06-8.03 (1H,d), 7.67-7.64 (1H,d), 7.49-7.48 (1H,d), 7.47-7.46 (2H,d), 7.44-7.42 (1H,d), 7.43-7.41 (2H,t), 7.40-7.38 (1H,t), 7.37-7.35 (1H,t), 7.34-7.33 (1H,t), 7.30-7.29 (2H,d), 7.27-7.25 (1H,t), 7.25-7.13 (1H,t), 5.59-5.61 (1H,d)(3J =2.34), 4.32-4.40 (2H,q), 4.30-4.25 (1H,d), 1.51-1.35 (3H,t); 13C NMR (75 MHz, CDCl3): 165.38, 140.66, 137.19, 137.14, 135.23, 133.58, 130.01, 129.81, 129.12 (2C), 128.46 (2C), 128.09 (2C), 127.27, 126.39, 123.27, 123.08, 122.69, 121.08, 119.08 (2C), 116.10, 109.08, 108.84, 64.93, 62.53, 37.86, 14.09.
1-(9-ethyl-9H-carbazol-3-yl)-4-(4-fluorophenyl)-3-phenylazetidin-2-one (3l, C29H23FN2O). Yield was 63%, giving white crystals. M.p.: 188.2 °C; 1H NMR (300 MHz, CDCl3): 8.12 (1H,s), 8.02-8.00 (1H,d), 7.47-7.46 (2H,d), 7.45-7.43 (1H,t), 7.42-7.41 (2H,d), 7.40-7.38 (2H,d), 7.37-7.36 (1H,d), 7.31-7.28 (2H,d), 7.26-7.25 (1H,t), 7.22-7.19 (1H,t), 7.12-7.07 (2H,t), 5.06-5.05 (1H,d)(J =2.34), 4.33-4.31 (2H,q), 4.29-4.28 (1H,d), 1.41-1.39 (3H,t); 13C NMR (75 MHz, CDCl3): 165.31, 140.65, 137.08, 135.08, 133.82, 133.78, 129.91, 129.35 (2C), 128.22, 128.06, 127.95, 127.80 (2C), 126.38, 123.21, 122.68, 121.01, 119.07, 116.73, 116.44, 116.08, 109.65, 109.00, 108.85, 65.37, 63.72, 37.84, 14.08.
General reaction
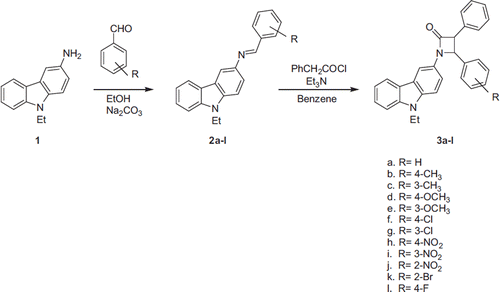
Results and discussion
All β-lactams were synthesized from the appropriate imines (2a-l), which were obtained by reaction between aldehydes and aromatic amine according to literature procedures. β-lactam ring formation was carried out by the addition of imines to ketenes in benzene. The effects of new β-lactam derivatives on the enzyme activity of a purified XO from bovine milk were assayed for enzyme activity in the presence of various concentrations of β-lactam derivatives. IC50 values were determined from the graphs () and are shown in .
Table I. Effects of new β-lactam derivatives on the enzyme activity of a purified XO from bovine milk.
XO, a cellular redox enzyme, is highly expressed in mammary epithelial cells (Stevens et al. Citation2000), but XO was extracted from fresh bovine milk without added preservatives using toluene and EDTA (Elriati et al. Citation2008). XO from bovine milk was purified using two-step procedures, namely ammonium sulphate precipitation and a Sepharose 4B-L-tyrosine-p-aminobenzamidine affinity chromatography (Beyaztaş and Arslan 2011). The purification procedure is described in the Experimental section and the results are summarized in . The purified enzyme was homogeneous by the criteria of denaturing and non-denaturing polyacrylamide gel electrophoresis (). Only one protein-staining band was detectable on gels loaded with up to 20 µg of protein.
Table II. Summary of the purification procedure of milk xanthine oxidase.
Figure 2. SDS-PAGE of xanthine oxidase. The poled fractions from affinity chromatography were analyzed by SDS-PAGE (12% and 3%) and revealed by Coomassie Blue staining. Experimental conditions were as described in the method. Lane 1 contained 5 μL of various molecular mass standards: 3-galactosidase, (116.0), bovine serum albumin (66.2), ovalbumin (45.0), lactate dehydrogenase (35.0), restriction endonuclease (25.0), 3-lactoglobulin (18.4), lysozyme (14.4). Only one protein-staining band was detectable on Line 2.
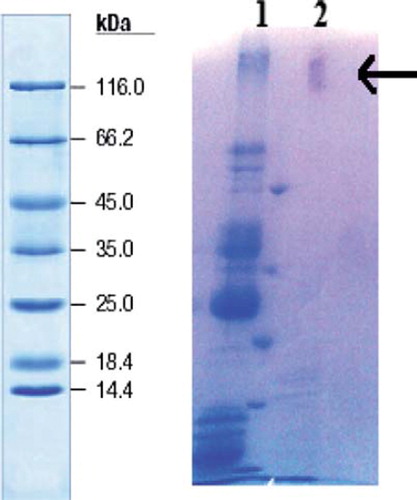
In this study, we examined the effect of new β- lactams derivatives on xanthine oxidase. The inhibitor molecule was bound in a long, narrow channel leading to the molybdenum-pterin active site of the enzyme. It filled up most of the channel and the immediate environment of the cofactor, very effectively inhibiting the activity of the enzyme through the prevention of substrate binding. Although the inhibitor did not directly coordinate to the molybdenum ion, numerous hydrogen bonds as well as hydrophobic interactions with the protein matrix were observed, most of which are also used in substrate recognition (Okamoto et al. Citation2003).
An increasing number of researchers during the past decade have also suggested that XO plays an important role in various forms of ischemic and other types of tissue and vascular injuries, inflammatory diseases, and chronic heart failure (Pacher et al. Citation2006a, Harrison Citation2002, Harrison Citation2004, Berry and Hare Citation2004). Allopurinol and its active metabolite oxypurinol showed beneficial effects in the treatment of these conditions, both in experimental animal models and in small-scale human clinical trials. Although some of the beneficial effects of these compounds go beyond inhibition of XO, these studies generated renewed interest in the development of additional, novel series of XO inhibitors for various therapeutic indications (Borges et al. Citation2002).
Inhibition of XO was thought to inhibit oxidation of 6-MP and potentiate the antitumor properties. XO was one of the test enzymes in the early experiments in the laboratory; several nontoxic XO inhibitors, including a hypoxanthine analog, allopurinol, were available to directly confirm this suggestion. It was known that XO is involved in the formation of uric acid from xanthine and hypoxanthine (Pacher et al. Citation2006b). Allopurinol is a drug used as an XO inhibitor in gout treatment (Nguyen et al. Citation2006). It is a substrate and specific potent inhibitor for XO, but oxypurinol (1H-pyrazolo [3,4-d]-pyrimidine-4,6 diol) is the basic functioning ingredient found in allopurinol. XO could catalyze the allopurinol to oxypurinol, and inhibition occurs mainly through direct substrate competition in the breakdown of purine (Tamta et al. Citation2006). The reaction has the effect of reducing the amount of concentration of insoluble urates in tissues, plasma, and urine, which leads to the reversal of urate crystal deposits in tissues and uric acid stones (Rang et al. Citation1999). XO also could catalyze 6-aminopurine to 8-hydroxyadenine and 2,8-dihydroxyadenine (the final product) then inhibited XO (Manfredi and Holmes Citation1985). Based on the structure of 6-aminopurine, scientists have selected 6-aminopurine and its analogues for XO inhibitory activity assay (Elriati et al. Citation2008). Allopurinol is a clinically used XO inhibitor, and its IC50 value was 5.43 μM (Fernandes et al. Citation2002). Allopurinol, 2-chloro-6(methyl amino) purine, 6-aminopurine, and 4-aminopyrazolo [3,4-d]-pyrimidine showed a strong inhibitory effect on XO, and the IC50 of these compounds were 7.82, 10.19, 10.89, and 30.26 μM, respectively. Moreover, 5-nitrobenzimidazole and 6-thioguanine showed a slight inhibitory effect on XO, and the IC50 of these compounds were 86.84 and 92.42, respectively. However, the other 6-aminopurine analogues did not show any significant inhibitory effect on XO. The inhibitory property was of allopurinol, 6-aminopurine, 2-chloro-6(methylamine) purine, and 4-aminopyrazolo [3,4-d] pyrimidine on XO. The inhibitory effects of allopurinol, 6-aminopurine, 2-chloro-6(methylamine) purine, and 4-aminopyrazolo [3,4-d] pyrimidine on XO were tested at different concentrations. XO inhibition increased significantly with the addition of allopurinol. Furthermore, similar trends were also observed in the results of 2-chloro-6(methylamine) purine, 6-aminopurine and 4-aminopyrazolo [3,4-d] pyrimidine. The XO inhibition reached a maximum when 30 μM of inhibitors was added, while the XO inhibition of allopurinol, 6-aminopurine, 2-chloro-6(methylamine) purine, and 4-aminopyrazolo [3,4-d] pyrimidine was 93.8, 61.3, 78.6, and 49.8%, respectively. The results showed that low concentrations (2–5 μM) of 2-chloro-6(methylamine) purine and 6-aminopurine demonstrated a higher XO inhibition than allopurinol and 4-aminopyrazolo [3,4-d] pyrimidine, while high concentrations (20–30 μM) of allopurinol and 2-chloro-6(methylamine) purine demonstrated a higher XO inhibition than 6-aminopurine and 4-aminopyrazolo [3,4-d] pyrimidine (Elriati et al. Citation2008). Scientists observed that the metabolism of 4-aminopyrazolo [3,4-d] pyrimidine has been studied in several normal and neoplastic mouse tissues (Henderson and Junga Citation1961). Moreover, the administration of 4-aminopyrazolo [3, 4-d]-pyrimidine decreased serum cholesterol level in the rat (Andersen and Dietschy Citation1976).
During the past decade, definite progress has also been achieved in the understanding of the XO enzyme structure, and rational drug development approaches led to the discovery of new, powerful XO inhibitors of various classes, including purine analogs, imidazole and diazole derivatives, and flavonoide, among many others (Borges et al. 2002). Two of these very potent new compounds, febuxostat [2-[3-cyano-4-(2-methylpropoxy) phenyl)-4-methylthiazole-5-carboxylic acid] and Y-700 (1-[3-cyano-4-(2,2-dimethylpropoxy) phenyl]-1H-pyrazole-4-carboxylic acid), were reported to have a favorable toxicology profile, high bioavailability, and more potent and longer-lasting hypouricemic action than allopurinol. These new compounds are currently in human clinical trials for the treatment of hyperuricemia and gout (Andersen and Dietschy Citation1976, Okamoto et al. Citation2004, Yamamoto Citation2003, Becker et al. Citation2004, Becker et al. Citation2005, Fukumari et al. Citation2004, Hoshide et al. Citation2004, Komoriya et al. Citation2004, Yamada et al. Citation2004, Hashimoto et al. Citation2005, Mayer et al. Citation2005, Takano et al. Citation2005, Naito et al. Citation2000). Therefore, the effects of new β-lactams derivatives have initially been studied on the purified xanthine oxidase enzyme activity in vitro. New β-lactams derivatives, whose effects we investigated in our studies, showed that different levels affected the xanthine oxidase. For the β–lactams’ shown inhibition effect, the IC50 values of the chemicals causing inhibition were determined by means of activity percentage-[I] diagrams. In this study, all β-lactams indicated inhibitory effect on the enzyme activity.
Conclusions
Enzyme inhibition is an important issue for drug design and biochemical applications (Aydemir and Kavrayan Citation2009, Gencer and Arslan Citation2011, Gencer and Arslan Citation2009, Sinan et al. Citation2007, Kiranoglu et al. Citation2007, Sinan et al. Citation2006). The results showed that new β-lactams derivatives inhibited the xanthine oxidase enzyme activity. β-lactams derivates are 3a, 3b, 3c, 3d, 3e, 3f, 3g, 3h, 3i, 3j, 3k, and 3l. The values of IC50 were 34.77, 47.42, 36.73, 31.64, 38.08, 21.65, 38.69, 32.79, 24.47, 30.37, 58.04, and 41.24 μM, respectively. The most effective compound for XO was 3f. In addition, IC50 values of β-lactams were similar to each other. Furthermore, the IC50 of β-lactams were similar to that of other XO inhibitors, which were well-known XO inhibitors clinically used for gout treatment. Therefore, our results suggested that new β-lactams derivatives are likely to be adopted as candidates to treat gout and may be taken for further evaluation in in-vivo studies.
Acknowledgements
The authors are grateful to the Sakarya BAP-2010-02-04-012 for financial support.
Declaration of interest
The authors report no conflicts of interest. The authors alone are responsible for the content and writing of the paper.
References
- Andersen JM, Dietschy J. 1976. Cholesterogenesis: derepression in extrahepatic tissues with 4-aminopyrazolo (3,4-d) pyrimidine. M. Science 193:903–905.
- Aydemir T, Kavrayan D. 2009. Purification and characterization of glutathione-S-transferase from chicken erythrocyte. Artif Cells Blood Substit Immobil Biotechnol 37:92100.
- Banner DW, Hadvary PJ. 1991. Crystallographic analysis at 3.0-A resolution of the binding to human thrombin of 4 active site-directed inhibitors. J Biol Chem 266:20085.
- Bashir S, Shah SM, Babar IJ. 2000. Allopurinol induced Stevens- Johnson syndrome: A case report. Pak Med Assoc 50:207.
- Becker MA, Kisicki J, Khosravan R, Wu J, Mulford D, Hunt B, MacDonald P, and Joseph-Ridge N. 2004. (TMX-67), a novel, non-purine, selective inhibitor of xanthine oxidase, is safe and decreases serum urate in healthy volunteers. Nucleo. Nucleotides Nucleic Acids 8–9:1111–116.
- Becker MA, Schumacher HR Jr, Wortmann RL, MacDonald PA, Eustace D, Palo WA, Streit J, and Joseph-Ridge N. 2005. Febuxostat compared with allopurinol in patients with hyperuricemia and gout. N. Engl. J. Med. 353:2450–2461.
- Berry CE and Hare JM. 2004. Xanthine oxidoreductase and cardiovascular disease: Molecular mechanisms and pathophysiological implications. J. Physiol. (Lond) 555:589–606.
- Beyaztas S, and Arslan O. 2011. Effects of some antibiotics on xanthine oxidase enzyme activities in vitro. Hacettepe J. Biol. & Chem. 39(2):205.
- Borges F, Fernandes E and Roleira F. 2002. Progress towards the discovery of xanthine oxidase inhibitors. Curr. Med. Chem. 9:195–217.
- Bradford MM. 1976. Rapid and sensitive method for the quantitation of microgram quantities of protein utilizing the principle of protein-dye binding. Anal Biochem 72:248.
- Clemente A, Domingos A, Grancho AP, Iley J, Moreira R, Neres J, Palma N, Santana AB, Valente E. 2001. Design, synthesis and stability of n-acyloxymethyl- and n-aminocarbonyloxymethyl-2-azetidinones as human leukocyte elastase inhibitors. Bioorg Med Chem Lett 11:1065.
- Elriati A, Loose J, Mayrhofer R, Bergmann H J, and Otto HH. 2008. β-lactam derivatives as enzyme inhibitors: Halogenated β-lactams and related compounds. Monatsh Chem 139:835.
- Fernandes E, Carvalho F, Silva AM, Santos CM, Pinto DC, Cavaleiro JA, Bastos Mde LJ. 2002. 2-styrylchromones as novel inhibitors of xanthine oxidase: A structure-activity study. J. Enzyme Inhib. Med. Chem. 17:45–48.
- Fukunari A, Okamoto K, Nishino T, Eger BT, Pai EF, Kamezawa M, Yamada I, and Kato N. 2004. Y-700 [1-[3-Cyano-4-(2,2-dimethylpropoxy)phenyl]-1H-pyrazole-4-carboxylic acid]: a potent xanthine oxidoreductase inhibitor with hepatic excretion. J. Pharmacol. Exp. Ther. 311:519–528.
- Gencer N, Arslan O. 2011. in vitro effects of some pesticides on PON1Q192 and PON1R192 isoenzymes from human serum. Fresen. Envir. Bull. 20:590–596.
- Gencer N, Arslan O. 2009. Purification human PON1(Q192) and PON1(R192) isoenzymes by hydrophobic interaction chromatography and investigation of the inhibition by metals J. Chrom. B-Anal. Tech. Biomed. Life Sci. 877:134–140.
- Georg GI, Ravikumar VT. 1992. The Organic Chemistry of β-lactams. Weinheim: VCH Publishers.
- Hammer B, Link A, Wagner A, Bohm M. 2001. Fatal allopurinol- induced hypersensitivity syndrome in asymptomatic hyperuricaemia. Dtsch Med Wochenschr 126:1331.
- Harris MD, Siegel LB, Alloway JA. 1999. Gout and hyperuricemia. Am. Fam. Physician. 59:925.
- Harrison R. 2002. Structure and function of xanthine oxidoreductase: Where are we now? Free Radic Biol. Med. 33:774–797.
- Harrison R. 2004. Physiological roles of xanthine oxidoreductase. Drug Metab. Rev. 36:363–375.
- Hashimoto T, Fukunari A, Yamada I, Yanaka N, Chen D, and Kato N. 2005. Y-700, a novel inhibitor of xanthine oxidase, suppresses the development of colon aberrant crypt foci and cell proliferation in 1,2-dimethylhydrazine-treated mice. Biosci. Biotechnol. Biochem. 69:209–211.
- Henderson JF, Junga IG. 1961. The metabolism of 4-aminopyrazolo(3,4-d)pyrimidine in normal and neoplastic tissues. Cancer Res. 21:118–129.
- Hoshide S, Takahashi Y, Ishikawa T, Kubo J, Tsuchimoto M, Komoriya K, Ohno I, and Hosoya T. 2004. PK/PD and safety of a single dose of TMX-67 (febuxostat) in subjects with mild and moderate renal impairment. Nucleo. Nucleotides Nucleic Acids 23:1117.
- Hsieh JF, Wu SH, Yang YL, Choong KF and Chen ST. 2007. The screening and characterization of 6-aminopurine-based xanthine oxidase inhibitors. Bioorg Med Chem 15:3450.
- Ishibuchi S, Morimoto H, Oe T, Ikebe T, Inoue H, Fukunari A, Kamezawa M, Yamada I, Naka Y. 2001. Synthesis and structure-activity relationships of 1-phenylpyrazoles as xanthine oxidase inhibitors. Bioorg Med Chem Lett 11:879.
- Isik S, Kockar F, Aydin M, Arslan O, Guler OO, Innocenti A, Scozzafava A, Supuran CT. 2009. Carbonic anhydrase inhibitors: Inhibition of the beta-class enzyme from the yeast saccharomyces cerevisiae with sulfonamides and sulfamates. Bioorg Med Chem 17(3):1158.
- Kiranoglu S, Sinan S, Gencer N, Kockar F, Arslan O. 2007. In vivo effects of oral contraceptives on paraoxonase, catalase and carbonic anhydrase enzyme activities on mouse. Biolog. Pharm. Bull. 30:1048–1051.
- Komoriya K, Hoshide S, Takeda K, Kobayashi H, Kubo J, Tsuchimoto M, Nakachi T, Yamanaka H, and Kamatani N. 2004. Pharmacokinetics and pharmacodynamics of febuxostat (TMX-67), a non-purine selective inhibitor of xanthine oxidase/xanthine dehydrogenase (NPSIXO) in patients with gout and/or hyperuricemia. Nucleo. Nucleotides Nucleic Acids 23:1119–1122.
- Laemmli UK. 1970. Cleavage of structural proteins during the assembly of the head of bacteriophage T4. Nature 227:680.
- Manfredi JP and Holmes EW. 1985. Purine salvage pathways in myocardium. Annu. Rev. Physiol. 47:691–705.
- Massey V, Brumby PE, Komai H. 1969. Studies of milk xanthine oxidase: Some spectral and kinetic properties. J Biol Chem 244:1682.
- Mayer MD, Khosravan R, Vernillet L, Wu JT, Joseph-Ridge N, and Mulford DJ. 2005. Pharmacokinetics and pharmacodynamics of febuxostat, a new non-purine selective inhibitor of xanthine oxidase in subjects with renal impairment. Am. J. Ther. 12:22–34.
- McManaman JL, Shellman V, Wright RM, Repine JE. 1996. Purification of rat liver xanthine oxidase and xanthine dehydrogenase by affinity chromatography on benzamidine-sepharose. Archives of Biochemistry and Biophysics, 332(1):135–141.
- Naito S, Nishimura M, and Tamao Y. 2000. Evaluation of the pharmacological actions and pharmacokinetics of BOF-4272, a xanthine oxidase inhibitor, in mouse liver. J. Pharm. Pharmacol. 52:173–179.
- Nguyen MT, Awale S, Tezuka Y, Ueda JY, Tran Q, Kadota S. 2006. Xanthine oxidase inhibitors from the flowers of Chrysanthemum sinense. Planta Med. 72:46–51.
- Ojima I. 1995. Recent advances in the beta-lactam synthon method. Acc. Chem. Res. 28:383.
- Okamoto K, Eger BT, Nishino T, Kondo S, Pai EF and Nishino T. 2003. An extremely potent inhibitor of xanthine oxidoreductase crystal structure of the enzyme-inhibitor complex and mechanism of inhibition. J Biolog Chem 278:1848–1855.
- Okamoto K, Matsumoto K, Hille R, Eger BT, Pai EF, and Nishino T. 2004. The crystal structure of xanthine oxidoreductase during catalysis: Implications for reaction mechanism and enzyme inhibition. Proc. Natl. Acad. Sci. USA 101:7931–7936.
- Özer N, Müftüoglu M, Ataman D, Ercan A, Ögüs IH. 1999. Simple, high-yield purification of xanthine oxidase from bovine milk. J Biochem Biophys Methods 39:153.
- Pacher P, Nivorozhkin A, and Szabo C. 2006a. Therapeutic effects of xanthine oxidase inhibitors: Renaissance half a century after the discovery of allopurinol. Pharmacol Rev 58:87–114.
- Pacher P, Nivorozhkin A, and Szabo C. 2006b. Therapeutic effects of xanthine oxidase inhibitors: Renaissance half a century after the discovery of allopurinol. Pharmacol. Rev. 58:87–114.
- Rang HP, Dale MM, Ritter JM. 1999. In: Ritter, J. Ed.; Pharmacology. New York: Pergamon Press. pp. 239–246.
- Sandstrom E. 1989. Antiviral therapy in human immunodeficiency virus-infection. Drugs 38:417.
- Sathisha KR, Khanum SA, Chandra JNNS, Ayisha F, Balaji S, Marathe GK, Gopal S, Rangappa KS. 2011. Synthesis and xanthine oxidase inhibitory activity of 7-methyl-2-(phenoxymethyl)-5H-[1,3,4]thiadiazolo[3,2-a]pyrimidin-5-one derivatives. Bioorg Med Chem 19:211.
- Sheu SY, Lin YC, Chiang HC. 1997. Inhibition of xanthine oxidase by synthetic cytokinin analogues. Anticancer Res 17:1043–1049.
- Sinan S, Gencer N, Turan Y, Arslan O. 2007. in vitro inhibition of the carbonic anhydrase from saanen goat (capra hircus) with pesticides. Pest. Biochem. Phys. 88:307–311.
- Sinan S, Kockar F, Gencer N, Yildirim H, Arslan O. 2006. Amphenicol and macrolide derived antibiotics inhibit paraoxonase enzyme activity in human serum and human hepatoma cells (HepG2) in vitro. Biochem.Moscow 71(1):46–50.
- Stevens CR, Millar TM, Clinch JG, Kanczler JM, Bodamyali T, Blake DR. 2000. Antibacterial properties of xanthine oxidase in human milk. The Lancet 356:829.
- Takano Y, Hase-Aoki K, Horiuchi H, Zhao L, Kasahara Y, Kondo S, and Becker MA. 2005. Selectivity of febuxostat, a novel non-purine inhibitor of xanthine oxidase/xanthine dehydrogenase. Life Sci. 76:1835–1847.
- Tamta H, Kalra S, Mukhopadhyay AK. 2006. Biochemical characterization of some pyrazolopyrimidine-based inhibitors of xanthine oxidase. Biochemistry Mosc. 71:49–54.
- Tamta H, Thilagavathi R, Chakraborti AK, Mukhopadhyay AKJ. 2005. 6-(N-benzoylamino)purine as a novel and potent inhibitor of xanthine oxidase: Inhibition mechanism and molecular modeling studies . Enz Inhib Med Chem 20:317.
- Tamta H, Kalra S, Mukhopadhyay AK. 2006. Biochemical characterization of some pyrazolopyrimidine-based inhibitors of xanthine Xoxidase. Biochemistry Mosc 71:S49–545.
- Yamada I, Fukunari A, Osajima T, Kamezawa M, Mori H, and Iwane J. 2004. Pharmacokinetics/pharmacodynamics of Y-700, a novel xanthine oxidase inhibitor, in rats and man. Nucleo. Nucleotides Nucleic Acids 23:1123–1125.
- Yamamoto T. 2003. Development antihyperuricemic candidates. Nippon Rinsho 61(Suppl 1):209–212.