Abstract
Context: Cholera is a severe diarrheal disease that remains an important cause of illness and death in many parts of the world.
Objective: This study has been designed to check the immune-stimulating potential of antigens in their native and associated form as chitosan microparticles in vitro.
Material and methods: Chitosan microparticles were prepared by the ionic gelation technique. The cell envelope proteins (CEPs) isolated from Vibrio cholerae were loaded as antigenic material. The prepared microparticles were characterized for their morphology, loading efficiency, particle size, and zeta potential.
Results: The average particle size of CEPs-loaded chitosan microparticles was 2.24 µm and the zeta potential of loaded microparticles was less than blank microparticles. The in vitro release studies of CEPs from CEPs-loaded chitosan microparticles exhibited slow and extended release over a period of time. The higher release of cytokine profile, including interferon γ (IFN-γ), tumor necrosis factor α (TNF-α), and interlukin-6 (IL-6), was observed for CEPs-loaded chitosan microparticles in comparison to CEPs as native antigen.
Discussion: The particle size of microparticles was within the range for phagocytosis by macropahges, which affects the immunogenicity. The decrease in zeta potential from blank to loaded microparticles further confirms the loading of antigen. The slow and extended release of CEPs provides continuous stimulus of antigen for a longer period of time. The cytokine profiling has shown the advantage of loaded microparticles over native antigen.
Conclusion: The in vitro release studies and cytokine profiling strongly suggested that CEPs-associated chitosan microparticles could be a potential candidate for oral vaccination against Vibrio cholerae.
Introduction
Cholera is an acute diarrhoeal infection caused by ingestion of food or water contaminated with the bacterium Vibrio cholerae. It has been estimated that there are 3–5 million cholera cases worldwide and among these, almost 100,000–120,000 deaths occur due to cholera every year (World Health Organization undated).
Conventional vaccines require repetitive injection to achieve long-lasting immune response. In such cases, biodegradable polymeric microparticles have been intensively studied as an alternative approach to achieving extended immune response in single vaccine formulations (Aguado and Lambert Citation1992, Cleland et al. Citation1996, Men et al. Citation1996). Microcapsules or microparticles are among the most common forms used for controlled-vaccine delivery formulations. Antigens, delivered through an oral (mucosal) route, are not frequently immunogenic in nature. Thus, adjuvants are required to be administered along with the antigens to enhance the immune responses (Mi et al. Citation1999). Microencapsulation with polymer matrices has received much attention in the last decade, resulting in a number of biomedical and industrial applications (Wang et al. Citation2006). Polymeric microparticles have been demonstrated to significantly enhance the systemic and/or mucosal immune responses after mucosal vaccination (Eyles et al. Citation1998). The mucosal immune system is a critical line of defense against infectious diseases, as the majority of infections are initiated at mucosal sites, and it has been demonstrated that the oral ingestion of antigens induces humoral and cellular immune responses not only at the site of antigen exposure, but also in other mucosal compartments (McGhee et al. Citation1992a, McGhee et al. Citation1992b, Xu-Amano et al. Citation1992, Mestecky Citation1987, Azizi et al. Citation2010). Chitosan is a biocompatible, biodegradable, and mucoadhesive polymer, which makes it a valuable excipient for mucosal drug delivery systems (Aranaz et al. Citation2009). Cell envelope proteins may be of great importance for the development of vaccines in the case of V. cholerae, as a large amount of toxin may be membrane-bound if it is grown under optimal growth conditions (Fernandes and Smith Citation1977). Chitosan is a positively charged polymer at pH lower than its pKa (6.5); in these conditions, negatively charged proteins bind easily to chitosan (Strand et al. Citation2003). Several studies have shown that chitosan may be a useful carrier for oral vaccination as it protects the antigen from the acidic environment of the gastrointestinal tract. In addition, it releases the antigen slowly from the carrier system after uptake by M cells of the peyer patches (Van der Lubben et al. Citation2001). In this study, cell envelope proteins (CEPs) as multiple antigens were loaded into chitosan microparticles and controlled release of loaded protein from the chitosan microparticles was analyzed in vitro. Further, the immune-stimulating activity of CEPs-loaded chitosan microparticles was checked in phorbol myristate acetate (PMA)-induced macrophages in THP 1 (Human monocyte cell-line).
Materials and Methods
Materials
Polymers and Reagents
Chitosan with average molecular weight of 150 K was supplied by Acros Organics (New Jersey, USA) and its deacetylation degree was ≥ 90 %. Sodium tripolyphosphate (TPP) was purchased from Sigma. All other chemicals were reagent grade chemicals.
Cell Lines
The THP 1 human monocyte cells were obtained from the National Centre for Cell Science (Pune, India). All reagents, including RPMI 1640 medium and supplements for cell culture, were purchased from Sigma (USA).
Preparation of antigens
Bacterial Strain
Vibrio cholerae MTCC 3906 (Serotype O139) were purchased from Institute of Microbial Technology (IMTECH), Chandigarh. The colony morphology and biochemical identification of strain was done using Thiosulfate citrate bile sucrose (TCBS) agar medium (selective media for identification and characterization of vibrio species) (McLaughlin Citation1995).
Isolation of Cell Envelope Proteins (CEPs) as an Antigenic Material
The cell envelope proteins (CEPs) were isolated according to the method developed by Manning et al. (Citation1982) with minor modifications. The selected strain of Vibrio cholerae was cultured in Luria Broth (LB) medium at 37° C for 24 h under shaking condition and then harvested. The harvested cells were suspended in TM buffer (10 mM Tris, 5 mM MgCl2, pH 7.5) and cell density was determined using a Mcfarland standard equivalent to 1011 cells/ml. The suspended cells were sonicated in a sonicator (Vibracell, Sonics & Materials, Inc., USA) at 20 kilo cycles/s until all cells were broken (six cycles of 30 s each). Then, the resulting suspension was centrifuged at 10,000 g for 1 min in a cold centrifuge to remove cell debris. After centrifugation, cell envelope proteins from the supernatant were pelleted by centrifugation at 10,000 g for 10 min and then, after washing, CEPs were freeze-dried (OPERON MPS -55, Yangchon-Myon, Korea) and stored at 4°C.
Preparation of chitosan microparticles
Chitosan microparticles were prepared by an ionotropic gelation technique according to the method developed by Park et al. (Citation2004). In this method, chitosan was dissolved in 1% aqueous acetic acid solution to achieve a polymer concentration of 0.25% w/v. Three ml of 1 % w/v sodium tripolyphosphate (TPP) anions were added drop-wise to 20 ml of 0.25% w/v chitosan solution under magnetic stirring and sonication (5 W, constant duty cycle). Further, chitosan microparticles were obtained by centrifugation at 3000 rpm for 15 min.
Characterization of microparticles
Loading of Cell Envelope Proteins (CEPs)
The freeze-dried CEPs (4mg/ml) were dispersed in 0.5 ml of phosphate buffered saline (PBS, pH 7.4) and added to 20 mg of chitosan microparticles. The resultant suspension was kept at 37°C for 24 h under shaking. After the incubation period, the suspension was centrifuged at 2500 rpm for 15 min. Further, the supernatant was analyzed for the unloaded CEPs by the Lowry protein assay method developed by Lowry et al. (Citation1951). The chitosan microparticles without CEPs were used as control and loading content was determined according to the following equation (Xu et al. 2003).
Scanning Electron Microscope (SEM) Study of Chitosan Microparticles and CEPs-loaded Chitosan Microparticles
Drops of chitosan microparticles and CEPs-loaded chitosan solution were placed on a conductive carbon tape. After air-drying at room temperature, the samples were gold-coated using a JEOL JFC-1600 Autofine coater (JEOL, Japan). The coated samples were observed using a JSM 6390LV Scanning Electron Microscope (JEOL, Japan).
Measurement of Dynamic Light Scattering (DLS)
The particle size of chitosan microparticles and CEPs-loaded chitosan microparticles was determined by a dynamic light scattering technique using Zetasizer (NanoZS; Malvern Instruments, Worcestershire, UK). The measurement conditions were as follows: He-Ne Red laser, 4.0 MW, 633 nm, temperature 25° C; refractive index 1.333; or with adjustment if needed. All measurements were done in triplicate using disposable polystyrene cuvettes (Malvern Instruments, UK).
Zeta Potential
The zeta potential of chitosan microparticles and CEPs-loaded chitosan microparticles was measured by photon correlation spectroscopy using Zetasizer (NanoZS; Malvern Instruments, Worcestershire, UK) equipped with a 4.0 mW He-Ne Red laser (633 nm). The preparations were diluted with double-distilled water for measurement of zeta potential. All measurements were done at 25°C in triplicate.
In vitro studies
In Vitro Release of CEPs from CEPs-loaded Chitosan Microparticles
The release of CEPs from CEPs-loaded chitosan microparticles was performed in a series of 1.5 ml microtubes. Each tube were filled with approximately 20 mg of microparticles in PBS (pH7.4) and kept at 37°C in a shaker incubator. An aliquot of 1ml was withdrawn from the release medium and equal volume was replaced with fresh PBS at each time of sampling. The amount of CEPs released was measured using the Lowry (1951) protein assay method. During release studies, integrity of CEPs was assessed in its native state and after the release from the microparticles using SDS-PAGE (12% gels), as described elsewhere (Almeida et al. Citation1993).
Measurement of Secreted Interferon γ (IFN-γ), Tumor Necrosis Factor α (TNF-α), and Interlukin-6 (IL-6)
THP 1 cells (human monocyte cell line) were cultured in RPMI 1640 medium supplemented with 10% fetal calf serum and 1% antimycotic-antibiotic mixture culture plates at 37°C, 5% CO2, and 95% relative humidity. Cells were seeded at a density of 2 × 105 cells/well in a 96-well plate and were induced to differentiate into macrophages by incubating in the presence of 20 nM of phorbol myristate acetate (PMA) for 14 h. Further, cells were washed and incubated in fresh medium (without antibiotic) for another 10 h. The CEPs and CEPs-loaded chitosan microparticles were added to designated wells to achieve a concentration of 1 µg/ml, respectively, in wells and incubated for three hours. The unloaded chitosan microparticles served as a control. Wells were then washed with incomplete RPMI-1640 medium to remove extracellular CEPs and CEPs-loaded chitosan microparticles and the complete media was replaced. The supernatants were harvested at different time intervals and, further, secreted IFN-γ, TNF-α, and IL-6 were measured by an enzyme-linked immunosorbent assay (ELISA) method (BD Biosciences) (Verma and Misra Citation2008).
Statistical analysis
All sample collections and experiments were done in triplicate under the same condition. One-way analysis of variance (ANOVA) followed by Bonferroni’s multiple comparison test were performed to determine the significant difference among the control, CEPs, and CEPs-loaded chitosan microparticles. The differences were considered significant if the probability values P < 0.05 were obtained.
Results
Characterization of chitosan microparticles
shows the typical scanning electron micrograph (SEM) of CEPs-loaded chitosan microparticles. The morphological studies through SEM indicated that CEPs-loaded chitosan microparticles were spherical while aggregated particles were asymmetrical in shape. The particle size distribution of CEPs-loaded chitosan microparticles and unloaded chitosan microparticles is shown in (a) and 2(b), respectively. It was observed that microparticles size increased after loading of CEPs. The average size of unloaded chitosan microparticles and CEPs-loaded chitosan microparticles was 1.10 ± 0.38 and 2.24 ± 0.31 µm, respectively. The result of zeta potential (NanoZS, Malvern Instruments, Worcestershire, UK) measurements revealed that CEPs were negatively charged with zeta potential of −25.3 mV and chitosan microparticles were positively charged with zeta potential of 25.82 mV. Further, the high loading content of CEPs (> 75 %) was observed with 0.25% concentration of polymer as used in this study.
Release of CEPs from CEPs-loaded chitosan microparticles in vitro
The release of CEPs from CEPs-loaded chitosan microparticles was determined in PBS (pH 7.4) at 37茄C using a shaker incubator as shown in . The release study was performed over a period of eight days and results indicated that approximately 91.75% of CEPs were released from CEPs-loaded chitosan microparticles. The SDS-PAGE analysis of CEPs is shown in . This analysis further confirmed the stability of the antigen as there was no change in the migration pattern of CEPs, suggesting that the antigen remains unaltered throughout the release studies.
Secretion of interferon γ (IFN-γ), tumor necrosis factor α (TNF-α), and interlukin- 6 (IL-6)
The cytokines profiling of interferon γ (IFN-γ), tumor necrosis factor α (TNF-α), and interlukin-6 (IL-6) using induced macrophages in the THP 1 cell line was carried out in order to investigate the immune-stimulating activity of CEPs and CEPs-loaded chitosan microparticles. The profiles of cytokine mediators, including IFN-γ, TNF-α and IL-6, are shown in , , and , respectively. Further, the release of IL-6 stimulated with CEPs-loaded chitosan microparticles from THP 1 cells was significantly increased (p< 0.05, n = 3) in a time-dependent manner and less cytokine was detected in THP 1 cells stimulated with blank chitosan microparticles. Additionally, higher secretion of TNF-α and IFN-γ was obtained from induced macrophages exposed to CEPs-loaded chitosan microparticles compared with those exposed to CEPs as the native antigen in ab unassociated state and blank chitosan microparticles.
Figure 5. Secretion of IFN-γ form THP1 cell line stimulated with (a) CEPs loaded chitosan microparticle; (b) CEPs; and (c) control (n = 3).
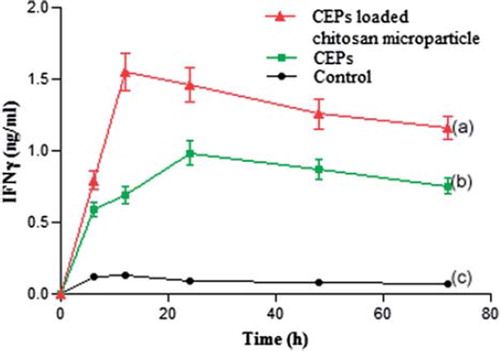
Discussion
The appropriate size of microparticles is an important parameter affecting immunogenicity and it is a well-established fact that particle sizes of less than 10 µm are significantly more immunogenic than larger ones (Eldridge et al. Citation1991, Eldridge et al. O’Hagan et al. Citation1993). In this study, it was observed that particle size distribution of CEPs-loaded chitosan microparticles was found to be well within the appropriate range to target macrophages present in gut-associated lymphoid tissues (GALTs). The zeta potential studies were carried out in order to study the charge alteration related to microparticles. It was found that blank and loaded chitosan microparticles were positively charged with zeta potential of + 25.82 mV and + 14.24 mV, respectively, and antigens were negatively charged with zeta potential of −25.3 mV. The positively charged blank chitosan microparticles allowed the CEPs to get easily associated through ionic interactions. The decrease in zeta potential of blank chitosan microparticles (+ 25.82 mV) to loaded chitosan microparticles (+ 14.24 mV) further confirmed the loading of antigens isolated from Vibrio cholerae. It was found that more than 75% of CEPs, antigens isolated from Vibrio cholerae, were associated with chitosan microparticles. The high loading content of the negatively charged antigens may be attributed to high molecular weight and high deacetylation degree of polymers used in this study. The in vitro release studies were performed to analyze the release of CEPs from CEPs-loaded chitosan microparticles. The results indicated that more than 90% of antigens were released over an extended period of eight days. The slow and extended release of antigens again may be attributed to the availability of a high number of amino groups due to the high degree of deacetylation. The freely available amino groups further increase the crosslinking between negatively charged antigens and positively charged chitosan microparticles (Dragat Citation1996). Further, SDS-PAGE analysis, as depicted in , also suggested that CEPs profile were similar during the process of microencapsulation and also during the release studies. Further in vitro experiments in THP 1 cell line were designed to achieve the targeted delivery of loaded microparticles within the macrophages to stimulate the immune response mediated through cytokines. The macrophage plays a central role in phagocytosis of antigens and transports them into ean ndocytic pathway for major histocompatibilty complex (MHC) presentation and T cell activation (East and Isacke Citation2002, Apostolopoulos and McKenzie Citation2001). Moreover, particles taken up by macrophages and dendritic cells lead to enhanced antigen presentation and release of cytokines to promote the induction of immune response (O’Hagan Citation1994). In this study, it was observed that there was significant difference in the level of IL-6 in the macrophages stimulated with loaded microparticles as compared to those stimulated with native antigen (P< 0.05, n = 3). Moreover, the higher release of cytokines, including TNF-α and IFN-γ, was exhibited in a time-dependent manner in loaded microparticles in comparison to native antigen and blank chitosan microparticles. The above findings suggest that antigen-loaded chitosan microparticles may serve as a suitable candidate for the targeted delivery of an efficient oral vaccine against Vibrio cholerae, as the conventional vaccine approach requires repetitive injection for long-lasting immune response (Aguado and Lambert Citation1992). The slow and controlled release of antigen from CEPs-loaded microparticles could be an alternate strategy to have extended immune response for longer duration, as it stimulated sustained release of the major cytokine mediators in a time-dependent manner, which is also evident from , , and , respectively. In the case of oral vaccination, antigens are frequently degraded in the acidic environment of the stomach. Hence, chitosan may be a useful carrier for oral vaccination as it protects the antigen from the acidic environment of the gastrointestinal tract with the advantage of releasing the antigens slowly within the macrophages (Van der Lubben et al. Citation2001). The slow release of antigens will further enhance the immune response for longer duration and it will also prolong the need for a booster dose as applicable in a conventional vaccine. The in vitro cytokine profiling was also supported by preliminary in vivo studies on mice carried out in our lab that showed a marked increase in immunogenic IgG level in serum as estimated through ELISA. Additionally, the detailed in vivo studies on mice will be further evaluated in the near future.
Conclusions
In this study, chitosan microparticles were prepared using an ionotropic gelation technique in order to encapsulate the cell envelope proteins isolated from Vibrio cholerae. The prepared microparticles were characterized to check particle size distribution, which governs the entry of microparticles within the macrophages. The in vitro release profile data suggested the slow and controlled release of antigens from chitosan microparticles, which may be exploited as a continuous stimulus of antigens for a longer period of time. The release of cytokine mediators in loaded microparticles has shown the advantage of immune-stimulating potential over native antigen. The results obtained from this study strongly suggest that CEPs-loaded chitosan microparticles could be a new candidate for oral vaccination to be used against the Vibrio cholerae species.
Acknowledgements
We sincerely appreciate the work supported by the Division of Pharmaceutics, Central Drug Research Institute, Lucknow, U.P., India.
Declaration of interest
The authors declare no conflicts of interest. The authors alone are responsible for the content and writing of the paper.
References
- Aguado MT, Lambert PH. 1992. Controlled-release vaccines- biodegradable polylactide/polyglycolide (PL/PG) microsphere as antigen vehicles. Immunobiology, 184: 113–25.
- Almeida AJ, Alpar HO, Brown MRW. 1993. Immune response to nasal delivery of antigenically intact tetanus toxoid associated with poly (L-lactic acid) microspheres in rats, rabbits and guinea pigs. J Pharm Pharmacol, 45: 198–203.
- Apostolopoulos V, McKenzie IF. 2001. Role of the mannose receptor in the immune response. Curr Mol Med, 1: 469-74.
- Aranaz I, Mengibar M, Harris R, Panos I, Miralles B, Acosta N, . 2009. Functional characterization of chitin and chitosan. Curr Chem Biol, 3: 203–30.
- Azizi A, Ghunaim H, Diaz-Mitoma F, Mestecky J. 2010. Mucosal HIV vaccines: A holy grail or a dud?Vaccine, 28: 4015–26.
- Cleland JL, Barron L, Berman PW, Daugherty A, Gregory T, Lim A, . 1996. Development of single-shot subunit vaccine HIV-1:2 defining optimal auto boost characteristics to maximize the humoral response. J Pharm Sci, 85: 1346–49.
- Draget KI. 1996. Association phenomenon in highly acetylated chitosan gel. Polym Gels Netw, 4: 143–51.
- East L, Isacke CM. 2002. The mannose receptor family. Biochim Biophys Acta, 1572: 364–86.
- Eldridge JH, Stass JK, Meulbroek JA, Tice TR, Gilley RM. 1991. Biodegradable and biocompatible poly(DL-lactide-co-glycolide) microspheres as an adjuvant for staphylococcal enterotoxin B toxoid which enhances the level of toxin- neutralizing antibodies. Infect Immun, 59: 2978–86.
- Eyles J, Sharp G, Williamson E, Spiers I, Alpar H. 1998. Intranasal administration of polylactic acid microsphere coencapsulated Yersinia pestis subunits confers protection from pneumonic plague in the mouse. Vaccine, 16: 698–707.
- Fernandes PB, Smith HL. 1977. Effect of anaerobiosis and bile salts on the growth and toxin production by Vibrio cholerae. J Gen Microbiol, 98: 77–86.
- Lowry O, Rosebrough N. 1951. Protein measurement with the folin phenol reagent. J Biol Chem, 193: 265–275.
- Manning PA, Imbesi F, Haynes DR. 1982. Cell envelope proteins in Vibrio cholerae. FEMS Microbiol Lett, 14: 159–66.
- McGhee JR, Kiyono H. 1992. Mucosal immunity to vaccines: Current concepts for vaccine development and immune response analysis. Adv Exp Med Biol, 327: 3–12.
- McGhee JR, Mestecky J, Dertzbaugh MT, Eldridge JH, Hirasawa M. 1992. The mucosal immune system: From fundamental concepts to vaccine development. Vaccine, 10: 75–88.
- McLaughlin JC. 1995. Vibrio. In: Murray PR, Baron EJ, Pfaller MA, Tenover FC, Yolken RH, eds., Manual of clinical microbiology. Washington DC: ASM Press, 1995, 465–76.
- Men Y, Gander B, Merke HP, Corradin G. 1996. Induction of sustained and elevated immune response to weakly immunogenic synthetic malarial peptides by encapsulation in biodegradable polymer microspheres. Vaccine, 14: 1442–50.
- Mestecky J. 1987. The mucosal immune system and current strategies for induction of immune responses in external secretions. J Clin Immunol, 7: 265–276.
- Mi FL, Shyu SS, Chen CT, Schoung JY. 1999. Porous chitosan microspheres for controlling the antigen release of Newcastle disease vaccine: Preparation of antigen- adsorbed microsphere and in vitro release. Biomaterials, 20: 1603–12.
- O’Hagan DT. 1994. Microparticles as oral vaccines. Novel delivery system for oral vaccines.Boca Raton, FL: CRC Press, 175–205.
- O’Hagan DT, Jeffery H, Davis SS. 1993. Long term antibody responses in mice following subcutaneous immunization with ovalbumin entrapped in biodegradable microparticles. Vaccine, 11: 965–69.
- Park IK, Jiang HL, Yun CH, Choi YJ, Kim SJ, Akaike T, . 2004. Release of Newcastle disease virus from CMs in vitro and in vivo. Asian- Australas J Anim Sci, 17: 543–47.
- Strand SP, Varum KM, Ostgaard K. 2003. Interactions between chitosans and bacterial suspensions: Adsorption and flocculation. Colloids Surf B, 27: 71–81.
- Van der Lubben IM, Verhoef JC, Van Aelst AC, Borchard G, Junginger HE. 2001. Chitosan microparticles for oral vaccination: Preparation, characterization and preliminary in vivo uptake studies in murine Peyer’s patches. Biomaterials, 22: 687–694.
- Verma RK, Misra A. 2008. Intracellular time course, pharmacokinetics and biodistribution of isoniazid and rifabutin following pulmonary delivery of inhalable microparticles to mice. Antimicrob Agents Chemother, 52: 3195–201.
- Wang W, Liu X, Xie Y, Zhang H, Yu W, Xiong Y, . 2006. Microencapsulation using natural polysaccharides for drug delivery and cell implantation. J Mater Chem, 16: 3252–67.
- World Health Organization. Cholera Fact Sheet. Available at http://www.who.int/mediacentre/factsheets/fs107/en/index.html. Accessed on 15 September 2011.
- Xu-Amano J, Beagley KW, Mega J, Fujihashi K, Kiyono H. 1992. Induction of T helper cells and cytokines for mucosal IgA responses. Adv Exp Med Biol, 327: 107–17.
- Xu YM, Du Y. 2003. Effect of molecular structure of chitosan on protein delivery properties of chitosan nanoparticles. Int J Pharm, 250: 215–26.