Abstract
To have advantages of reduced dosing frequency, improved bioavailability and effective delivery system of Cefuroxime Axetil, a Chitosan based intragastric sustained release microbead formulation of Cefuroxime Axetil was developed. The drug delivery system was prepared by ionotropic gelation of Chitosan in presence of sodium tripolyphosphate as polyanion and optimized by box-behnken experimental design. Response surface methodology was applied to evaluate various vitro characteristics of prepared mucoadhesive microbeads. Multiple independent variables were optimized to achieve responses of interest, thereby to get the desired sustained release profile of Cefuroxime Axetil in gastric environment.
Introduction
Chitosan, a natural polysaccharide comprising copolymers of glucosamine and N-acetyl glucosamine, is being widely used as a pharmaceutical excipient. It is obtained by the partial deacetylation of chitin a natural polymer. Chitosan has a high charge density due to its linear polyamine structure, which adheres to negatively charged surfaces, leading to chelation of metal ions and formation of salts with acids (Singla and Chawla Citation2001). Chitosan has received considerable attentions as a possible pharmaceutical excipient in recent decades due to its biodegradability, biocompatibility, and antimicrobial properties, which can be utilized for numerous pharmaceutical applications (Olivia et al. Citation1998). To utilize the mucoadhesive ability of chitosan, we investigated the crosslinking of chitosan in the presence of a polyanion sodium tripolyphosphate (STP), as previously described (Bodmeier et al. Citation1989). Prepared mucoadhesive microbeads have been further utilized for their release rate controlling properties in designing an intragastric delivery system for cefuroxime axetil (CXA).
Cefuroxime is a broad-spectrum, β-lactamase stable cephalosporin antibiotic. It is poorly absorbed from the gastrointestinal (GI) tract but has a well-defined pharmacokinetics when administered intramuscular and intravenously in the form of sodium salt (Bethesda 2003, Wozniak and Hicks Citation1991). On the other hand, the esterified form of cefuroxime, i.e. CXA, can be administered orally for its valuable therapeutic potential (James and Elliott Citation1989). CXA is a 50:50 mixture of two diastereoisomers and,as a prodrug of cefuroxime, it has the bioavailability of 36% and 52%, respectively, in fasted and fed conditions. The esterases present in gut lumen are found to hydrolyze CXA to the non-absorbable cefuroxime, resulting in its incomplete bioavailability (Mosher et al. 1992). It is also known that the amorphous form of CXA possesses more bioavailability as compared to its crystalline form and, at the same time, when the amorphous form comes in contact with water, it forms a gel leading to prolonged disintegration and retarded dissolution of CXA in tablet formulations. The gelling tendency of CXA further depends upon temperature, pH, and ionic strength of the dissolution media (Somani et al. Citation2001). A formulation, which can improve the bioavailability of CXA by sustained drug release in the gastric region to justify the need of its improved delivery, could be highly desirable for a formulator. Previous attempts suggest development of intragastric formulation for CXA (Dhumal et al. Citation2006, Patel and Patel Citation2006). Acidic gastric conditions as the preferred site of absorption for CXA can be judged from the fact that the absorption of the cefuroxime ester has been found to be enhanced postprandially as delayed gastric emptying leads to its more complete dissolution. Similarly, reduction in bioavailability as a result of raising the intragastric pH on concomitant administration of ranitidine or sodium bicarbonate leads to diminished absorption due to incomplete dissolution of CXA (Sommers et al. Citation1984). Hence an intragastric sustained release (ISR) mucoadhesive system of CXA is believed to prolong its release to the site of absorption to prevent the saturation of a carrier-based transport mechanism. Absorption of CXA is confined mainly to the upper part of the GI tract, which can also be understood from its increased solubility, chemical and enzymatic stability, and enhanced absorption profile in acidic pH values of stomach. In order to protect the degradation of CXA, its poor absorption and to avoid conditions like antibiotic-associated colitis, a gastroretentive system that will also prolong drug release in the upper GI tract could be developed to ensure optimal bioavailability. A chitosan-based mucoadhesive approach seems to be best suited among the several chitosan-based approaches currently being used to prolong gastric retention time, including high-density systems and low-density, floating drug-delivery (Hejazi and Amiji Citation2003). CXA is given in terms of equivalents of cefuroxime to treat various bacterial conditions (Sweetman Citation2002) and an immediate release CXA form can be replaced by its ISR once-a-day formulation. Hence the present investigation aims at the development and in vitro evaluation of a chitosan-based intragastric, multiparticulate, mucoadhesive system for CXA which will provide greater safety by eliminating chances of dose dumping and easy dose conversion as a function of a number of multi units for different indications.
Materials and Methods
Materials
Cefuroxime axetil provided by M/s FDC India Ltd., Mumbai, India, was used as the model drug for study. Chitosan (652 and 222 grades) with a degree of deacetylation of 90% each with different viscosities of 3000 cps and 500 cps, respectively, were obtained from M/s DKSH India Pvt. Ltd., Mumbai, India. Empty, hard, gelatin capsule shells (size “1”) were supplied by M/s Sanofi-Aventis India Ltd., Goa. Sodium tripolyphosphate, acetic acid glacial, tween-80, and all other reagents and chemicals used were of analytical grade from M/s Loba Chemie Pvt. Ltd., Mumbai, India. Distilled water was used throughout the study for all procedures as required.
Methods
Drug Excipients Compatibility Studies
Compatibility between drug and excipients was investigated by Fourier Transform Infrared (FTIR) Spectroscopy and differential scanning calorimetry (DSC). For FTIR spectroscopy, spectra were recorded for wave number from 400 to 4000 cm21 against a KBr disc as a blank standard using an FTIR spectrometer (JASCO-460 Plus, Japan) and for DSC a thermal analyzer (Mettler TA 4000, Switzerland) was used and DSC was performed in a nitrogen atmosphere at a heating rate of 10°C/min over a temperature range of 30° 2300°C, employing an empty aluminum pan as a reference standard. The blend-containing drug and excipients (all the excipients together in proposed ratio) were directly exposed to the elevated conditions of temperature and relative humidity (40° ± 2°C and 75% ± 5% RH) for one month. Finally, the IR spectra and DSC thermograms recorded were compared with that of the pure drug, excipients, and unexposed drug-excipients blend. Additionally, X-ray powder diffraction (XRPD) patterns were recorded to confirm the amorphous nature of CXA (alone and in formulation) on the X-ray diffractometer (PW 1729, Philips, The Netherlands), where samples were irradiated with monochromatized Cu-Kα radiation (1.542 Å) and analyzed from 5° to 50° 2θ. The voltage and current used were 30 kV and 30 mA, respectively. The range and the chart speed were 5 × 103 cycles/sec and 10 mm/°2θ, respectively.
Preparation of CXA Microbeads
Chitosan-based cefuroxime axetil (CCXA) microbeads were prepared by the modified ionotropic gelation method as previously described (Bodmeier et al. Citation1989, Durkut et al. Citation2006). Briefly, the desired amount of chitosan was dissolved in 10 mL of aqueous acetic acid solution (2% v/v) by stirring for about 6 h to get a uniform and clear viscous solution; the weighed amount of CXA was then dispersed in a 4 mL aqueous tween-80 solution (0.5% w/v) and mixed until a uniform paste was formed. The paste was then added to the previously prepared chitosan solution and homogenized to remove any entrapped air. The resultant drug-chitosan gel was extruded drop-wise (with a controlled rate of 1 mL/min) through a 24-gauge needle into the excess (100 mL) of the aqueous STP solution of desired concentration under mild stirring while maintaining the falling distance constant at 10 cm from the surface of the STP solution. Spherical gel beads formed instantaneously were allowed to remain in the STP solution for up to 30 min for curing and were collected by filtration through a nylon cloth. The formed beads were gently rinsed with distilled water and kept for overnight drying in air, followed by oven drying at temperatures not exceeding 40°C for about 2 h. All the trial batches were prepared and evaluated in triplicate. A placebo batch without CXA was also prepared following similar procedures by extruding 4% w/v chitosan solution (in 2% v/v acetic acid) into 5% w/v STP solution. The final products were stored in a desiccator for future characterization and in-vitro studies.
Preliminary Trials
Ionotropic gelation is the most accepted method for the preparation of beads and the multiparticulate system being used mostly for calcium alginate beads. The drug-containing chitosan beads have been known for the last two decades and have been tried for several drug delivery approaches for various drug candidates, but CXA as a model drug has not been utilized before, therefore the preliminary trials and even the process design involved were critical. Due to the water-insoluble nature and pH-dependent characteristics, CXA was a good choice for the proof of concept studies. Preliminary trials were mainly carried out to identify factor levels for different variables yielding a desirable response by challenging concentration and viscosity grade of chitosan (wall material), quantity of tween-80, pH, and concentration and volume of STP (crosslinker) solution, agitation speed, rate of extrusion, crosslinking (curing) time, and drying patterns. Different characteristics of formed microbeads in terms of their in vitro characterization were evaluated leading to the further optimization studies based on the experimental design.
Response Surface Methodology (RSM)
Design of experiments has been widely accepted in the pharmaceutical field to study the effect of formulation variables and their interaction on response variables, hence a factorial design to optimize the experiments on the basis of response surface methodology was employed (Singh and Ahuja Citation2000, Lewis et al. Citation1999, Armstrong Citation2006). A three-factor, three-level box-behnken experimental design (BBD) was used in the optimization of the CCXA microbead formulation and experimental trials were performed using all suggested 12 combinations and, additionally, a central point combination was repeated five times to ensure precision in the experiments. Concentrations of chitosan solution (X1), CXA dispersion (X2), and STP solution (X3) were selected as independent variables. A suitable range of independent factors was chosen based on the preliminary trials in order to adequately measure its effects on the response (dependent) variables, which in the present study were % yield (Y), % entrapment efficiency (EE), % mucoadhesive strength (MS), and time required for 90% of drug release (T90). Three levels were determined for each independent variable and 17 trial batches were prepared according to BBD along with one control (Placebo) batch, factor codes, and actual values as outlined in , which were finally evaluated for characteristic parameters. Furthermore, all experiments were equally practical and feasible. BBD provides sufficient data to fit a quadratic polynomial analysis for evaluation of various dependent variables, followed by utilization of RSM to optimize the factor combinations using Design Expert® software (Version 7.1.1, Stat Ease Inc., Minneapolis, MN, USA). All tests were performed for at least 95% level of significance (α = 0.05); power ensured to be as low as 80% for desired effect. The adequacy of the final quadratic models was examined by fit summary, analysis of variance (ANOVA), 3D response surface graphs, and contour plots prepared using Design Expert®. Coefficients in the quadratic equation (b0, b1, b2, b3, b12, b13, b23, b11, b22, b33) were related to the effects and interactions of the various factors, where b0 is the arithmetic mean response of all 17 trials, b1 to b33 are regression coefficients computed from the observed experimental values of Yi, and terms X1, X2 and Xi2 (i = 1, 2, or 3) represent the interaction and quadratic terms, respectively. The interaction shows how the dependent variable changes when two or more independent factors are simultaneously changed from their low to high values, which can be explained by Equation (1).
. Levels and values for independent factors in Box-Behnken experimental design
Evaluation of Microbeads
Drug content
A UV spectrophotometric method (PharmaSpec UV-1700, Shimadzu, Japan) was developed for estimation of CXA content and validated appropriately. 1 gm of CCXA microbeads was finely powdered using a mortar-pestle and a quantity of fine powder equivalent to 50 mg of CXA was transferred in a 100 mL volumetric flask, about 80 mL of 0.1 N HCl was added to it and ultrasonicated (PCi, Mumbai, India) at a temperature below 20°C for 10 minutes. Final volume was made up with 0.1 N HCl and mixed properly; 10 mL solution then was filtered using a 0.45 μ nylon filter (N66, Pal Lifesciences, India) and analyzed spectrophotometrically at λmax 273 nm for available CXA content after appropriate dilutions were done.
Percent yield
Dried CCXA microbeads were weighed and percent yield (%Y) was calculated by comparing the final weight obtained with the initial weight of the ingredients taken for preparation of a particular batch using the formula given in Equation (2).
Entrapment efficiency
For determination of entrapment efficiency (EE), microbeads containing 50 mg CXA were dissolved in 100 mL of 0.1 N HCl by overnight shaking in a shaker incubator at 200 rpm. The solution was analyzed as described in the “Drug content” section for available CXA content and the entrapment efficiency was calculated by Equation (3). All the experiments were carried out in triplicate and placebo beads were used to prepare the blank.
Size, shape, and surface morphology
The shape and size of microbeads were determined through an optical microscope (LABO, Kolkata, India) with a stage micrometer and the surface morphology of the optimized and placebo microbead formulations was studied by scanning electron microscopy (SEM). The purpose of the SEM was to obtain a topographical characterization of the beads, which was performed by initially fixing the microbead samples on aluminum stubs with conductive double-sided adhesive tape coated with the gold by a sputter coater at 50 mA for 50 sec, followed by microscopy through (JEOL, JSM 26400, Tokyo, Japan) operated at an acceleration voltage of 5.0 kV at the required magnification to determine the size, shape, and surface morphology of the microbeads.
Swelling index
Swelling index of the microbeads was measured as a function of percent fluid uptake, which in turn is the capacity of the microbeads to absorb the media in proportion to their dry weights, and was determined by soaking 20 microbeads in 0.1 N HCl (pH 1.2) solutions at 37°C. Microbeads were removed every hour and weighed after removing the excess water using filter papers. The extent of swelling index was determined by repeating the above process until equilibrium was reached and no further weight gain was observed. The swelling index was determined by calculating the fluid uptake using Equation (4).
where W1 is initial weight of the dry microbeads prior to the soaking and W2 is the weight of wet microbeads at equilibrium with the media.
Mucoadhesive strength
Mucoadhesive strength of CCXA microbeads was calculated in terms of percentage of in vitro mucoadhesiveness determined by a slight modification of previously reported wash-off methods (Santus et al. Citation1997, Zhou et al. Citation2007, Patil et al. Citation2009). Briefly, in this technique a goat's small intestine (freshly collected from a local slaughterhouse) was cut longitudinally and washed with saline for 10 min; from that, a 7-cm separated portion was attached on a semi-cylindrical Plexiglas support inclined at a 45° angle as per the set-up shown (). Next, 50 previously hydrated (with little 0.1 N HCl) microbeads were subsequently placed gently (within 2 min of hydration) onto the mucosa without application of any force and left undisturbed for a period of 20 min (at constant temperature of 37°C and 90% relative humidity); following this period of incubation, the microbeads were eluted with 0.1 N HCl (maintained at 37°C) using a peristaltic pump over a period of 20 min maintaining the flux of 22 mL/min and the microbeads detaching from the mucosal surface were collected and counted. The experiment was repeated three time for a single batch of CCXA microbeads and the mean value of mucoadhesiveness or % mucoadhesive strength of microbeads was determined using the formula given in Equation (5). Similarly, the chitosan-only microbeads were also tested as a controlled experiment for mucoadhesive strength for comparison purposes.
where MA is the number of microbeads placed initially to mucosa and MD is the number of microbeads detached from the mucosa following elution.
In vitro drug release studies
Drug-loaded microbeads equivalent to 250 mg CXA were filled in size “1” hard gelatin capsule shells and dissolution studies (n = 6) were performed for them using dissolution conditions as per British Pharmacopoeia (Citation2006). 900 mL of 0.1 N HCl at 37 ± 0.5°C was taken as dissolution media and apparatus II, with paddles (with sinkers) using dissolution test apparatus (Disso 2000, LabIndia, Mumbai, India) at 50 rpm. 10 mL of samples were withdrawn (replenished duly with an equal volume of fresh medium) at each sampling point (1, 2, 3, 4, 5, 6, 8, and 10 h) and were analyzed as described in the “Drug content” section for the amount of CXA released during the dissolution studies. Additionally, drug release studies for the finally optimized formulation of CCXA microbead were performed in four different gastric physiological medium, viz. 0.1 N HCl (pH 1.2), 0.01 N HCl (pH 2.0), simulated gastric fluid (SGF, pH 1.2, USP 30, without pepsin), and acetate buffer (pH 4.5, USP 30) to check the pH-dependent release behavior of the microbeads.
Mathematical modelling of drug release profile
The dissolution profile of the optimized formulation was fitted to different model equations to ascertain the kinetic modelling of the in vitro drug release profile. Several mathematical models, as previously described (Costa and Lobo Citation2001), were applied using SigmaPlot® exact graphs and data analysis software (Version 10, Systat Software Inc., USA) and curve fittings were investigated in terms of slope (k), coefficient of correlation®, coefficient of determination (R2), adjusted coefficient of determination (Adj. R2), and best fitting kinetic models.
Batch reproducibility and stability studies
CCXA microbead batches of optimized formulation (MB 17), as per the results obtained from the experimental design and the RSM optimization process solution by the software, were repeated three times to check the reproducibility of the process and performance (through various in vitro characteristics, as discussed in the previous sections) and finally subjected to accelerated stability studies in accordance with the ICH guidelines (2003). All three batches were filled separately in hard gelatin capsules shells size “1” (250 mg cefuroxime equivalents per capsule), duly packed and sealed in triple-laminated aluminum pouches and charged at 40° ± 2°C and 75% ± 5% RH conditions (Environmental Test Chamber, HMG-INDIA, Mumbai, India) for a period of six months. The samples were withdrawn after completion of 1, 2, 3, and 6 months and analyzed for the drug content and in vitro drug release studies, as per the methods described in previous sections.
Results and Discussion
Drug excipients compatibility studies
FTIR studies reveal that all the excipients employed for the preparation of CCXA microbeads are compatible and well accepted by the drug, as shown in (A, B, C, and D), with all the characteristic bands and major stretchings in CXA FTIR spectra due to different functional groups and aromatic rings. All the stretch characteristics at 3479–3209 cm21[N-H stretch of primary and secondary amines], 1784 cm21 [band due to β-lactam ring], 1683 and 1535 cm21 [bands due to amido group], 1595 cm21 [2OCONH2 the carbamate stretch], 1377 cm21 [C-N stretch of β-lactam ring], 1074–1010 and 1155 cm21 [C-N stretchings due to side chains], 754 cm21 [S-C stretch of β-lactam ring], and 592 cm21 [stretches due to alkene and ether] were seen unchanged (found intact, without band broadening and stretch shifts) in subsequent spectra of unexposed and exposed blends of the drug with excipients when compared together and found predominantly absent in spectra of excipients, which implies the absence of any solid-state interaction between the drug and the excipients
Figure 2. FTIR Spectra of (A) Unexposed CXA + Excipients blend, (B) Exposed CXA + Excipients blend, (C). Excipients blend without CXA and (D) Untreated CXA.
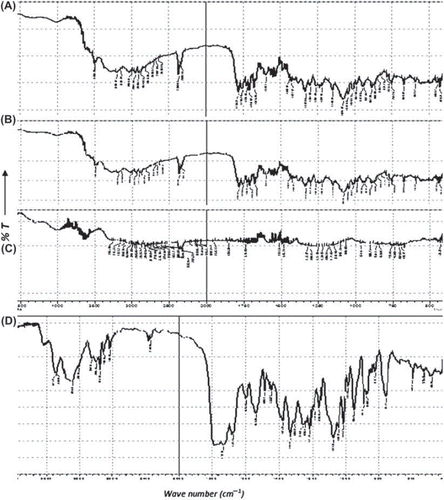
Drug excipient compatibility was also confirmed by DSC studies through thermal analysis of CXA and its blend with other excipients. It was critical to ensure that CXA was retained in the same form even in the presence of all the excipients. The DSC curve of CXA given in (A, B, C and D) shows a small endothermic peak at about 80°C, indicating the presence of one of the two amorphous forms of CXA, which melts at 80°C as previously reported (Woo and Chang Citation2000, Jo et al. Citation2002). There is also a small endothermic diffuse peak at around 180°C corresponding to its fusion temperature, confirming the amorphous nature of the drug, and finally a crystallization pattern at and above 200°C, which was also present in the blend containing drug and the excipients (but absent in the blend containing only excipients). The broad curve around 80°C, devoid of the small endothermic peak, can be seen in the exposed and unexposed formulation blend, which also confirms the stability of cefuroxime axetil.
Figure 3. DSC Th ermograms of (A) Untreated CXA, (B) Excipients blend without CXA, (C) Exposed CXA + Excipients blend and (D) Unexposed CXA + Excipients blend.
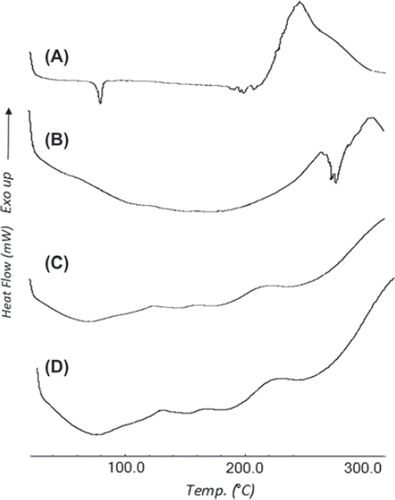
Additionally, to confirm the amorphous nature of CXA in microbeads, the XRPD pattern of the CCXA microbead formulation was compared with that of the untreated CXA and found to have similar patterns of amorphous CXA without any characteristic diffraction peak (), which supports the FTIR data, eliminating any kind of drug-excipients interaction.
Preliminary trials
As the chitosan-coated alginate beads are one of the most common systems (Huguet et al. Citation1996, Hari et al. Citation1996), initially alginate beads containing CXA were tried with secondary chitosan coatings for additional applications, but due to the increase in bulk and decreased availability of chitosan for its sustained release and mucoadhesive properties the approach was discontinued.
Preliminary studies to obtain only chitosan beads were carried out using (0.5, 1, 2, 3, and 4% w/v) chitosan in 1 or 2% v/v acetic acid solution as an internal phase and (1, 2, 3, 4, 5, 7.5, and 10% w/v) STP solution as external medium. Theuantity of CXA in the internal phase and its uniform dispersion with the use of tween-80 (as wetting agent) was also considered and higher concentrations were found to perform well.
Two different viscosity grades of chitosan were tried, but with less gel strengths (leading to formation of friable discs), even at higher concentrations, chitosan 222 (low viscosity grade) was not considered for further trials. 2% v/v acetic acid solution to get higher levels of chitosan 652 (high viscosity grade) solutions (2–4% w/v) was found to produce spherical and sufficiently strong microbeads in the presence of anionic counterions present in the STP solution. It was also found that all the concentrations above 2.5% w/v of STP solution were capable of crosslinking chitosan properly but the higher concentrations ( 7.5% w/v) tended to elevate the solution pH values 10, thereby creating more probability of CXA degradation (as described in the Introduction).
A floating approach was also tried to be incorporated in the present microbead systems to give additional support to the mucoadhesive intragastric formulation, but due to the use of sodium bicarbonate as a gas (CO2) generating agent, generally used as already reported (Talukder and Fassihi Citation2004, Somani et al. Citation2009), chitosan gels did not maintain the structure and dispersed in the external phase (a result of the increased pH of the chitosan gel and ionic interaction between sodium bicarbonate and acetate ions), ultimately leading to failed crosslinking.
The agitation speed of the stirrer during the formation of the microbead was kept as minimal as possible to avoid any disorder and damage to the surface of the microbeads; similarly, the rate of extrusion was fixed at 1 mL/min as it led to the optimum-sized microbeads without bubble formation or air entrapment and twinning. The same was true for drying patterns mainly attributed to the shape of the microbeads, hence overnight air drying was preferred followed by removal of additional moisture by oven drying at a temperature below 40°C, until loss on drying was below 1%w/w, which was monitored by a moisture analyzer (MA-100, Sartorius, Germany).
Optimization by RSM
BBD was utilized for the study as a tool to RSM to find the ideal process settings, thereby achieving optimal performance in terms of dependent variables. BBD was selected as it is especially employed for more than three levels and could go for up to 10 factors (3 factors were considered in the present study). BBD ensured limited experiments to be run (as 17 trial combinations were finalized for the study) and additionally allowed the use of quadratic models. Good workable concentrations (low to high levels) of all three independent variables were set in consequence of the preliminary trials. Y, EE, MS, and T90 values for the 12 + 5 batches (Trials MB 01 to MB 17 as per BBD) showed a wide variation, for which the results are shown in . The polynomial equations were used to draw conclusions after considering the magnitude of coefficients with positive or negative signs. High values of coefficient of determination (R2) indicate goodness of fit towards the control over the sources of variations and high values of Adjusted R2 and Predicted R2 (with minimum difference between the two) reveal very less probability of insignificant terms in the model. The data clearly indicate that the values of dependent variables are strongly dependent on the independent variables and equations obtained are highly significant in terms of P > F values (determined by ANOVA). Details of all interactions in form of mathematical relationships are discussed in . Apart from that, single factor correlation between one independent variable (keeping the other two independent variables constant at their mid-level values) and different response variables as per can also be used to predict the influence of the dependent variable over the response variables. Finally, selection of the optimized formulation was done on the basis of the results obtained from the BBD trials and various suggestions fed, in the form of constraints for the optimization solution exercised as a part of BBD-RSM. The constraints are discussed in along with the details of best possible solution and predicted best desired responses. Central point reproducibility can also be seen in terms of responses as per , which determines good precision and less experimental error in the design.
. Experimental design and corresponding responses in terms of dependent variables and evaluation parameters.
Table III. ANOVA for response surface quadratic models correlating different response variables.
. Details of constraints set for RSM, and optimized formula proposed by Design Expert® software.
Evaluation of microbeads
Drug Content
CXA content in the microbead formulation was estimated for all the optimization trial batches and found to lie between 97 to 100.8% in terms of cefuroxime. The concentration of STP solution had an impact on the drug content, as very low concentrations led to leaching the CXA into the external phase and extremely high concentrations of STP tended to degrade CXA during the preparation of the microbeads. Extremely high concentrations of chitosan solution had chances of increased solubility of CXA and leaching into the external phase, which could result in the low drug contents (e.g. 97% and 98.1% for trials MB 05 and MB 01, respectively), as evident from results in .
Percent Yield and Entrapment Efficiency
The Response variables Y and EE were found to be significantly dependent on virtually all the independent variables as evident from the single factor correlation () and as can be interpreted from the 3D response graphs and contour plots (), as well as from the experimental results displayed in , which all are outcomes of RSM. Both Y and EE were found to have similar impacts from all three independent variables, viz. X1, X2 and X3. The role of chitosan has been critical in the case of these two response variables as optimum concentration of chitosan solution is required to get the maximum value of Y, since higher as well as lower concentrations result in the lower percent yield and entrapment efficiency (e.g. Trial MB 01, 07, 10, and 13), which may be due to formation of extremely low and high consistency CCXA gels during the preparation of the microbeads. However, higher concentrations of CXA dispersion and STP solution are desired to get higher values of Y and EE since CXA acts as a bulk former for the microbeads and STP solution fastens the crosslinking of CCXA gel, hence lower amount of both the variables may lead to the improper or distracted CCXA microbeads. To get better percent yield and entrapment efficiency, higher values of CXA and STP are desired, although optimum concentration of chitosan will be required.
Size, Shape, and Surface Morphology
The beads of all the batches (except preliminary trials) were well within the 700–850 µm size range, including the control formulation. The size of CCXA microbeads was intentionally kept in this range to attain good mucoadhesion as well as a desired sustained release profile, as a larger size might lead to lower MS by virtue of its high weight and alternately a too small size will definitely not control the CXA release rate. The size of microbeads was precisely controlled by a proper selection of the needle orifice and the rate of extrusion. CCXA microbeads of optimization trial batches were equally uniform, spherical, and had a fibrous surface as evident by SEM images (). It can also be illustrated by the SEM images that CXA is responsible for the integrity of microbeads (uniform size and spherical shapes), as in and B, as the microbeads without CXA content, i.e. control formulation (MB 18), neither possessed size uniformity nor spherical structure, as seen in and E. Similarly, roughness and the fibrous nature of the microbead surface of the control formulation (MB 18) may be found to be attributed to the high chitosan content, as seen in . Alternately, optimized formulation MB 17 in shows a comparatively smooth and less fibrous microbead surface. Since the roughness and fibrous nature of the microbead are highly desired for improved mucoadhesion, the chitosan content should be as high as possible along with the higher levels of CXA to get the spherical shape and uniform size.
Swelling Index
The swelling behavior of microbeads is particularly due to chitosan content (being only a crosslinking polymer utilized for microbead preparation). Swelling occurs when microbeads are placed in aqueous media as a result of relaxation of crosslinked chains of chitosan and generation of pores leading to imbibing of media through capillary action. Swelling capacity or swelling index represents the hydration capacity of the microbead, which plays a major role in the bioadhesion with mucosal surface as it ultimately strengthens the mucoadhesive strength. Release of CXA from the microbead occurs due to controlled erosion of the swelled microbead, which can be modulated by appropriate selection of chitosan and CXA contents. Similarly, rate of hydration of microbeads can be controlled by the amount of crosslinking agent used. The swelling index was found to govern the MS and T90, but there is no correlation of swelling index with Y and EE, as can be seen from the optimization trials in . Although CCXA microbeads followed pH-dependent swelling characteristics, resulting in pH-dependent release characteristics, both the swelling index and release were found to have an inverse correlation due to the rapid ionization of D-glucosamine residues of chitosan at lower pH, resulting in extensive swelling and faster release (and vice versa), in contrast to the release mechanism that is generally utilized by most of the swollen hydrogel matrices (Siepmann and Peppas Citation2001). Hence ultimate understanding has been to have the microbeads with rapid rate of hydration but slow rate of swelling to get optimum effects in terms of mucoadhesion and the drug release rate.
Mucoadhesive Strength
Mucoadhesive strength of the CCXA microbeads is the function of their chitosan content. The mucoadhesive properties of chitosan are well known and have been studied extensively. High degree of deacetylation and high molecular weight result in high degree of mucoadhesion, which further strengthens the electrostatic interactions between positively charged chitosan and anionic glycoproteins (sialic acids) of the mucin (Bonferoni et al. Citation2009). As an intragastric system, gastric mucosal tissue (from the goat stomach region) was used initially to evaluate the mucoadhesiveness of the microbeads as per the method described, but due to its complex, web-like structure at the mucosal side it was found difficult to predict the MS of microbeads as these tend to stick up in the complex structure and do not wash off while rinsing with the aqueous media; hence it was decided to carry out all the experiments using goat intestinal mucosa, which could offer realistic results. MS of the microbeads was dependent on chitosan concentration to a great extent as can be predicted by single-factor correlation (), through the sharp inclination of 3D response surface and straight lines in contour plots (). There is no or minimal impact (inversely proportional) of CXA and STP concentrations on the MS due to its direct correlation with chitosan concentrations. Most of the optimization trials were found to have good MS (higher by MB 01, 04, 13, 16, and 17 batches), probably due to the higher concentrations of chitosan solution used in accordance with the preliminary trials. Only a few formulations (MB 08 and MB 11) were found to have the lowest MS due to minimum chitosan content in the microbeads. There is no correlation of MS with the other dependent and independent variables, as shown by the optimization trial results in ; furthermore, MS is the most desirable variable, hence given maximum importance during the setting up of constraints for an optimization study through RSM, as the microbeads prepared only with chitosan also revealed better mucoadhesion strength.
In-vitro Drug Release Studies
Since the extended and complete release of the CXA from microbeads was the ultimate object, only T90 as the release parameter has been considered for the study, in spite of the time needed to get initial 25% or 50% drug release values. 0.1 N HCl as dissolution media was selected since it is the media used for IR dosage forms of CXA and, as the intragastric system, the same was found to be most suitable. Also, the media with higher pH values (e.g. pH 6.8 phosphate buffer) was found not to be working (in terms of drug release characteristics) for the CCXA microbead system and also not simulating gastric conditions. Overall, responses in terms of time in hours required to get the desired cumulative drug release were found to be dependent on the concentrations of chitosan and CXA together, as both the ingredients are required to obtain the desired profile. It is clearly evident from the contour plots and response graphs in that the amount of CXA is mainly responsible for obtaining the sustained release profile (due to its lower solubility in aqueous media), while optimum chitosan concentrations (ranging lower to medium) are required to get the additional retardation by virtue of the complex crosslinked structure in the microbeads. On the other hand, concentration of STP hardly had any influence on the T90 values as the necessary anions were available for crosslinking of the CCXA gel (factor might govern T90 values), even at the minimum concentrations, although it would depend on the volume of STP solution taken as the external phase during the formation of the microbead, as it was decided to keep the volume in substantial excess in accordance with the observations in preliminary trials. Further, the release characteristics of optimized CCXA microbeads (MB 17) were investigated in four different dissolution media with gastric physiological pH ranges (CCXA microbeads being the intragastric system) and found to have a pH-dependent release profile (faster release in more acidic conditions), as per , where faster dissolution profiles were obtained in 0.1 N HCl and SGF (without pepsin), both having pH 1.2, and comparatively slower release profiles in 0.01 N HCl (pH 2) and pH 4.5 acetate buffer due to the elevated pH values. The pH-dependent release characteristics tend to be attributed mainly to the acid solubility of both chitosan and CXA, hence producing more extended release at elevated pH conditions. It can also be predicted that the fed conditions will definitely impart further retardation in release along with enhance absorption due to increased residence time.
Curve fitting with mathematical models
The dissolution profile of the optimized formulation (MB 17) in 0.1 N HCl was fitted for various mathematical model equations (using nonlinear regression analysis) and found to be fitting well to Hixon Crowell and First Order release kinetics (with significant and acceptable values of k, R, R2, and Adj. R2). The Hixon-Crowell model indicated an erosion-type release mechanism as the geometrical shape of formulation diminished proportionally (surface erosion) over time, leading to a dissolution-rate-dependent controlled release. First-order release kinetics confirmed that the release of drug from a formulation is proportional to the amount of drug remaining in its interior, hence microbead formulations were found to be following both the release mechanisms as the release profile fit both the model curves completely. Further, the Hixon Crowell and first-order release kinetics allowed an initial loading dose and ensured proper drug release (40–50% drug releases in initial 2–3 h), partially eliminating the impact of gastric transit apart from having the added advantages of a multiparticulate system such as easy dose conversion (function of number of microbeads).
Batch reproducibility and stability studies
Reproducibility of CCXA microbead formulations was confirmed by repeating the MB 17 batch three times and performing various in vitro tests for all four response variables. These were found to behave well according to the results predicted by optimization trials by RSM. Y and EE of the reproducibility batches ranged from 94–96% and 96–98%, respectively, whereas MS ranged from 95–96% and T90 was found to be 8 h for all three batches. All three formulations were found to have good stability over accelerated conditions for up to six months as ascertained by evaluation of drug content and in vitro drug release of exposed formulations, and were found to have no significant changes in physical and chemical attributes compared to initial values.
Conclusion
The CCXA microbeads were developed and optimized using a Box-Behnken experimental design and found to have sufficient in vitro characteristics to behave as an intragastric mucoadhesive delivery system for site-specific delivery of CXA. In vitro evaluation studies were found to be very effective and indicative to prove pH-dependent swelling and thereby mucoadhesion and drug release characteristics of CCXA microbeads. Optimization of the multiparticulate mucoadhesive drug delivery approach overcomes the drawback of the all-or-none principle of gastric emptying for single unit systems and also eliminates the risk of dose dumping. The approach has sustained release (8 h profile) properties to ensure extended systemic availability of cefuroxime. However, the proposed delivery systems seem to be working effectively in fed gastric conditions and further evaluation in terms of its in-vivo behavior will be sought in future. Moreover, the developed chitosan-based system is stable and cost-effective due to the minimum number of excipients involved and can be easily manufactured without the use of sophisticated equipment. The approach can also be utilized for intragastric delivery of several other drugs.
Declaration of interest
The authors report no conflicts of interest. The authors alone are responsible for the content and writing of the paper.
References
- Armstrong AN. 2006. Pharmaceutical experimental esign and interpretation, 2nd ed. Boca Raton, FL: Taylor & Francis Group.
- Bodmeier R, Oh KH, Pramar Y. 1989. Preparation and evaluation of drug-containing chitosan beads. Drug Dev Ind Pharm. 15:9:1475–94.
- Bonferoni MC, Sandri G, Rossi S, Ferrari F, Caramella C. 2009. Chitosan and its salts for mucosal and transmucosal delivery. Expert Opin Drug Del. 6:9:923–39.
- British Pharmacopoeia. 2006. Cefuroxime axetil tablet monograph. London: British Pharmacopoeia Commission.
- Costa P, Lobo JMS. 2001. Modeling and comparison of dissolution profiles. Eur J Pharm Sci. 13:123–33.
- Dhumal RS, Rajname ST, Dhumal ST, Pawar AP. 2006. Design and evaluation of bilayer floating tablets of cefuroxime axetil for bimodal release. J Sci Ind Res. 65:812–16.
- Durkut S, Elcin YM, Elcin EA. 2006. Biodegradation of chitosan-tripolyphosphate beads: In vitro and in vivo studies. Artificial Cell Blood Subst Biotechnol. 34:263–76.
- Hari PR, Chandy T, Sharma CP. 1996. Chitosan/calcium alginate microcapsules for intestinal delivery of nitrofurantoin. J Microencap. 13:3:319–29.
- Hejazi R, Amiji M. 2003. Chitosan based gastrointestinal delivery systems. J Control Release. 89:151–65.
- Huguet ML, Neufeld RJ, Dellacherie E. 1996. Calcium-alginate beads coated with polycationic polymers: Comparison of chitosan and DEAE-dextran. Process Biochem. 31:4:347–53.
- International Conference on Harmonisation of Technical Requirements for Registration of Pharmaceuticals for Human Use. 2003. Stability testing of new drug substances and products Q1A (R2). Current Step 4 version: dated 6 February 2003. Available at: http://www.ich.org/LOB/media/MEDIA419.pdf.
- James MB, Elliott LG. 1989. Pharmaceutical composition comprising Cefuroxime Axetil, United States Patent 4,865,851, September 12, 1989.
- Jo GH, Yu JH, Yun JH, Hwang SJ, Woo JS. 2002. Preparation of amorphous form of cefuroxime axetil using supercritical fluid processing. Controlled Release Society 29th Annual Meeting Proceedings, Seoul, South Korea, July 20–25, 2002.
- Lewis GA, Mathieu D, Phan-Tan-Luu R. 1999. Drugs and the pharmaceutical sciences: Pharmaceutical experimental design. New York, Marcel Dekker Inc.
- Mosher GL, McBee J, Shaw DB. 1992. Esterase activity toward the diastereomers of Cefuroxime Axetil in the rat and dog. Pharm Res. 9:5:687–89.
- Olivia F, Pierre B, Robert G. 1998. Chitosan: A unique polysaccharide for drug delivery. Drug Dev Ind Pharm. 24:11:979–93.
- Patel VF, Patel NM. 2006. Intragastric floating drug delivery system of cefuroxime axetil: In vitro evaluation. AAPS PharmSciTech. 7:1:17:E118–24.
- Patil DA, Patil GB, Deshmukh PK, Belgamwar VS, Fursule RA. 2009. Chitosan coated mucoadhesive multiparticulate drug delivery system for gliclazide. Asian J Pharm Clin Res. 2:2:62–68.
- Santus G, Lazzarini C, Bottoni G, Sandefer EP, Page RC, Doll WJ, . 1997. An in vitro, in vivo investigation of oral bioadhesive controlled release furosemide formulations. Eur J Pharm Biopharm. 44:39–52.
- Siepmann J, Peppas NA. 2001. Modeling of drug release from delivery systems based on hydroxypropyl methylcellulose (HPMC). Adv Drug Del Rev. 48:139–57.
- Singh B, Ahuja N. 2000. Book review on pharmaceutical experimental design. Int J Pharm. 195:247–48.
- Singla AK, Chawla M. 2001. Chitosan: Some pharmaceutical and biological aspects - An update. J Pharm Pharmacol. 53:1047–67.
- Somani JK, Bhushan I, Sen H. 2001. Bioavailable oral dosage form of cefuroxime Axetil, United States Patent 6,323,193, November 27, 2001.
- Somani VG, Shashi SR, Udavant YK, Atram SC, Satpute R, Shinde MN. 2009. A floating pulsatile drug delivery system based on hollow calcium pectinate beads. Asian J Pharm. 3:120–24.
- Sommers DK, Wyk MV, Moncrieff J, Schoeman HS. 1984. Influence of food and reduced gastric acidity on the bioavailability of Bacampicillin and Cefuroxime Axetil. Br J Clin Pharmacol. 18:535–39.
- Sweetman SC, ed. 2002. Martindale: The Complete Drug Reference, 33rd ed. London: The Pharmaceutical Press, 178.
- Talukder R, Fassihi R. 2004. Gastroretentive delivery systems: A mini review. Drug Dev Ind Pharm. 30:10:1019–28.
- Woo JS, Chang HC. 2000. Non-crystalline cefuroxime axetil solid dispersant, process for preparing same and composition for oral administration thereof. United States Patent 6,107,290. August 22, 2000.
- Wozniak T, Hicks J. 1991. Cefuroxime sodium. In: Florey K, ed., Analytical Profiles of Drug Substances. San Diego, CA: Academic Press, 209–237.
- Zhou HY, Guang CX, Zhang WF. 2007. In vitro and in vivo evaluation of mucoadhensiveness of chitosan/cellulose acetate multimicrospheres. J Biomed Mater Res A. 83:4:1146–53.