Abstract
Background: Enzymatical conversion of A or B RBCs into group O RBCs (ECORBCs) was achieved by using α-N-acetylgalactosaminidase and α-galactosidase, respectively. Now, we initiated AB to O-RBC conversion by using these two enzymes together. But α-N-acetylgalactosaminidase and α-galactosidase's preserving and their reaction buffer were quite different. The aim of this study is to confirm an available system for converting AB to O RBCs, especially to study the maximal permission amount of PCS which was brought to the system-accompanied enzyme addition. Method: Enzyme activity was detected by using GalNAc-pNp or Gal-pNp as substrates. The efficiency of the conversion of A or B antigen was evaluated by routine method and measured by fluorescence-activated cell sorting analysis. The optimal buffer component and the doses of α-N-acetylgalactosaminidase and α-galactosidase were confirmed according to A and B antigen epitope removal efficiency. Results: The activity of α-N-acetylgalactosaminidase and α-galactosidase was not decreased drastically when they were kept in PCS Buffer in 4°C. The optimal reaction buffer composed of glycine 250 mM and NaCl 3 mM, pH 6.8 and PCS less than 10%(v/v). For converting A1B to O RBCs completely, the doses of α-N-acetylgalactosaminidase and α-galactosidase were confirmed as 0.015 mg/ml packed RBCs(pRBCs) for A1 antigen epitopes and 0.005 mg/ml pRBCs for B epitopes. Approximately 0.004 mg α-N-acetylgalactosaminidase and 0.005 mg α-galactosidase were required to convert 1 ml pRBCs. Conclusion: Our studies indicated that α-N-acetylgalactosaminidase and α-galactosidase were stable in PCS buffer and a modified protocol which was propitious to converting AB to O RBCs was provided.
Introduction
In clinical blood transfusion, group A, B, AB, and O RBCs were transfused to A, B, AB, and O recipients, respectively. In fact, except homotype transfusion, group A, B, and AB recipients are also able to accept group O red blood cells (RBCs). Thus, O RBCs are called ‘universal’ RBCs. Theoretically, α-N-acetylgalactosaminidase and α-galactosidase could remove immunodominant monosaccharides of A and B antigens, respectively, according to the structure characters of A and B immunodominant monosaccharides (a terminal α-1,3-linked N-acetylgalactosamine, GalNAc and an α-1,3-linked galactose, Gal). In fact, the former studies had proved that A or B RBCs can be converted to ECORBCs by treating with α-N-acetylgalactosaminidase or α-galactosidase, and the data of phase I and phase II clinical trials showed that B-ECORBCs were safe and functional (Lenny et al. Citation1991, Citation1994, Kruskall et al. 2000). Although, the study clearly demonstrated that enzymatic conversion of RBCs is feasible and that B-ECORBCs can function as well as ABO-matched untreated cells in transfusions, a major obstacle to clinical implementation of this method is the large quantity of enzyme required. Until 2007, an international team of scientists reported two bacterial glycosidase gene families that provide enzymes capable of efficient removal of A and B antigens at neutral pH with low consumption of recombinant enzymes. They have created a cheap and simple way to convert all donated blood into group O, which could eventually reduce blood shortages and make transfusions safer (Liu et al. Citation2007).
The molecular structure of A antigen is more complex than that of B antigen. There are two subgroups in A RBCs, A1 and A2 RBCs. Subgroup A1 has five times as much A antigens as subgroup A2. In addition, subgroup A1 cells have glycolipids with a repeated A structure, while A2 cells have GalNAcs binding to H structure by Glycosidic bond (Clausen et al. Citation1986, Citation1985). α-N-acetylgalactosaminidase from Meningosepticum, can remove A1 and A2 epitopes (Liu et al. Citation2007, Yu et al. Citation2008). Its mechanism is deemed to a sugar oxido-reductase-acquired glycosidase activity (Daniels and Withers Citation2007). α-galactosidase from Bacteroides fragilis can remove B epitope by its specific exo-glycosidases capability. The optimal reaction buffer was 250 mmol/L glycine, 3 mmol/L NaCl in our results (Gao et al. Citation2011a, Citation2011b). The problem was that when the concentration of α-N-acetylgalactosaminidase or α-galactosidase increased in glycine, the precipitation appeared and enzyme activity decreased even kept in 4°C, so glycine is not suitable for enzyme preserving. But our previous data of B to O conversion using α-galactosidase from coffee bean as hydrolase indicated that α-galactosidase was stable in PCS buffer (Na2HPO4 77.25 mmol/L, citric acid 11.375 mmol/L, NaCl 53.9 mmol/L), pH 6.8. Thus, we just preserved these two enzymes in PCS and the storage test indicated that the activity of α-N-acetylgalactosaminidase preserved in PCS in the ninth month retained about 86%. But the catalytic reaction was made in glycine buffer. In order to facilitate description, α-N-acetylgalactosaminidase is called A-zyme in this article, and α-galactosidase is called B-zyme. The reaction buffer (250 mmol/L glycine, 3 mm NaCl) is called glycine buffer, and enzyme-preserving buffer (Na2HPO4 77.25 mmol/L, citric acid 11.375 mmol/L, NaCl 53.9 mmol/L) is called PCS buffer.
After converting A and B RBCs to O RBCs successfully, we initiated the work of AB to O RBCs. We found that a few of PCS would be carried into the reaction system when enzymes were added, because of PCS buffer with higher iron concentration. If PCS exceeded its permission, the enzymatic activity would be infected. So we initiated to find an appropriate percentage of PCS and the enzyme doses for converting AB to O RBCs.
Materials and methods
Stability of α-N-acetylgalactosaminidase and α-galactosidase
To evaluate the stability assay of α-N-acetylgalactosaminidase and α-galactosaminidase, the activities of samples preserved for different lengths of time were detected. The maximum limitation of preserving time was nine months. Assays with monosaccharide p-nitrophenyl (pNP) structures were carried out at 26°C in reaction mixtures of 400 μL with 100 mmol/L NaPO4, 50 mmol/L NaCl and 2.5 mmol/L substrate. Reaction was terminated with 600 μL 1 M Na2CO3, and pNP formation was quantified at 405 nm.
Confirmation of the unique buffer
Ultrafiltration was used to change α-N-acetylgalactosaminidase's keeping buffer (glycine) to α-galactosidase's keeping buffer (PCS), then the characters of α-N-acetylgalactosaminidase and antigen epitope-removing efficiency were observed. At the same time, α-galactosidase was studied with buffer changing. To make the mechanism clearly, the enzymes associated with RBCs in A or B buffer were measured by western blot analysis. According to the result of A, B epitope-removing efficiency, the unique buffer showed intending to be a glycine buffer predominantly. While α-galactocdase is kept in PCS, if we put α-N-acetylgalactosaminidase and α-galactosidase together, a small amount of PCS buffer could be carried into reaction buffer. In order to ascertain the maximum permission of PCS, A and B antigen epitope-removing efficiency was measured with a series of mixture of buffer A and B ().
Table I. Conversion efficiency comparing in a series of different amount PCS in glycine system.
Confirmation of dose of enzyme A and B
The optimal dose of A and B enzymes were confirmed, by typing with standard blood-banking techniques using licensed monoclonal antibody reagents ().
Table II. Blood group typing ECO RBCs with enzyme dose titrations.
Enzymatic conversion of RBCs with enzyme A and B
Fresh whole blood was obtained from department of Transfusion, Affiliated Hospital of Academy of Military Medical Sciences and buffy coat was removed. Enzymatic conversion of AB-RBCs was performed in 1 ml reaction mixtures containing 250 mmol/L glycine, pH 6.8 and 3 mmol/L NaCl with 40% packed RBCs and enzymes as indicated. Briefly, RBCs were prewashed 1:1 and 1:4 (v/v) in conversion buffer before addition of enzymes A and B, and the reactions were incubated for 60 minutes with gentle mixing at 26°C, followed by four repeated washing cycles with 1:4 (v/v) of PBS by centrifugation at 2000 rpm for 5 minutes. The washed ECORBCs were ABO-typed according to standard blood-banking techniques with licensed monoclonal antibody reagents. Murine monoclonal anti-A and anti-B blood grouping reagents obtained from Changchun Brother Biotech Corporation Limited Changchun, China.
Flow cytometry
Flow cytometry analysis of native and enzyme-converted RBCs was performed by using a FACScan flow cytometer (CYTOMICS FC500, BECKMAN COULTER, USA) with FITC-conjugated monoclonal anti-A(BD Biosciences, USA), UEA1(anti-H, Sigma-Aldrich), anti-B blood-grouping reagents (Changchun Brother Biotech Corporation Limited, Changchun, China), FITC-conjugated AffiniPure Goat Anti-Mouse IgM (Jackson ImmunoResearch LABORATORIES, INC). Briefly, 10 μL cells were fixed over night at room temperature under gentle agitation by the addition of 100 μL of 2% paraformaldehyde (w/v, Sigma-Aldrich) in PBS to prevent agglutination of antigen-positive cells. 1 μL pRBCs were prewashed with PBS twice and resuspended in 100 μL PBS. Then 2 μL of undiluted primary antibody was added and incubated for 60 minutes in darkness at 25°C. After two washes and resuspension in 100 μL PBS, 2 μL of undiluted secondary antibody was added and incubated for 60 minutes in darkness at 25°C. Cells were then analyzed after another two washes (as above) and resuspended in 500 μL PBS. A total of 50,000 events were evaluated.
Results
Confirmation of the unique buffer
We observed that mild precipitates occurred when A-zyme and B-zyme were changed into glycine buffer, and activity analysis showed that A-zyme and B-zyme were more stable in PCS buffer than in glycine buffer (). In PCS buffer, A-zyme did not show high activity at all, B-zyme could work but its antigen epitope-removing efficiency was 50 times lower than in glycine buffer (Gao et al. Citation2011b). These results indicated that PCS fitted for A and B-zyme preserving while glycine buffer could provide an optimal enzymatic reaction condition. To further study how PCS does affect the enzyme activity, the enzyme in supernatant was detected by western blot after centrifugation with the mixture of enzymes, RBCs and buffer (). The result indicated that few enzymes were detected when A or B-zyme mixed with RBCs in glycine buffer, but almost all enzymes appeared in supernatant when in PCS buffer. These results agreed with Liu's results (Liu et al. Citation2007). To summarize the above results, the glycine buffer showed a suitable effect to enzyme A and B both, but PCS was only functional for enzyme preservation.
Figure 1. Stability of A-zyme in PCS in 9 months. α-N-acetylgalactosaminidase was stored in PCS buffer at 4°C. The activities of preserved samples were monitored every one month by using 4-Nitrophenyl N-acetyl-α-D-galactosaminide (GalNAc-pNp) as substrate. The results showed that activities did not decrease excessively, even in the ninth month, the activities still retained at about 86%.
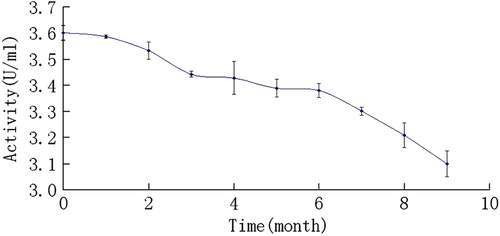
It seems that the PCS buffer and glycine buffer are different. A few of PCS would be carried into the system, once enzyme was added in. So PCS permission amount has to be confirmed first. This result showed that when the amount of PCS exceeded 10%, the enzyme A would not work well (). In agreement with our hypothesis, increased PCS negatively affected the conversion efficiency, showing that the interaction is important for efficiency. (). Therefore to convert AB to O RBCs completely, the total supply of PCS should not exceed 10%.
Figure 2. α-N-acetylgalactosaminidase combining with RBCs detection in the mixture of AB-RBCs enzymes and buffers. Supernate enzymes in the mixture of RBCs: enzymes and buffers were detected by western blot after centrifugation. A: Free α-N-acetylgalactosaminidase in different amount PCS added in glycine. The total volume was 500 μL&UnknownEntity1;in which, RBCs were 200 μL, PCS and glycine were 300 μl. Lane 1: PCS 0 μL, glycine 300 μL; Lane 2: PCS 10 μL, glycine 290 μL; Lane 3, PCS 20 μL, glycine 280 μL; Lane 4: PCS 30 μL, glycine 270 μL; Lane 5: PCS 40 μL, glycine 260 μL; Lane 6: PCS 50 μL, glycein 250 μL; Lane 7: no RBCs were added in the system. B: α-N-acetylgalactosaminidase binding with different blood group cells in different buffer. 1–4: α-N-acetylgalactosaminidase binding with A, B, AB, and O RBCs, respectively, in glycine buffer; 5–8. α-N-acetylgalactosaminidase binding with A, B, AB, and O RBCs, respectively, in PCS buffer; C: Free α-galactosidase in different amount PCS added in glycine. The total volume was 500 μL, in which, RBC was 200 μL, PCS and glycine were 300 μL. Lane 1:PCS 0 μL, glycine 300 μL; Lane 2: PCS 10 μL, glycine 290 μL; Lane 3,pcs 20 μL, glycine 280 μL; Lane 4: PCS 30 μL, glycine 270 μL; Lane 5: PCS 40 μL, glycine 260 μL; Lane 6:PCS 50 μL, glycein 250 μL; Lane 7: no RBCs were added in the system.
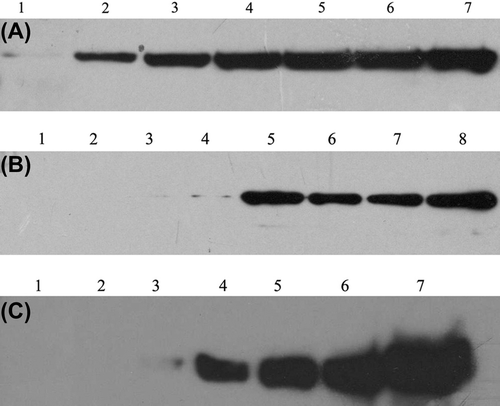
Optimal dose of enzyme A and B for AB converting
Optimal dose of enzyme A and B for A1B and A2B RBCs was tested respectively, by typing with standard blood-banking techniques using licensed monoclonal antibody reagents and methods as well as by sensitive fluorescence-activated cell sorting (FACS) analysis ( and ). For A1B, the consumption of enzyme A was 0.015 mg/ml pRBCs, and enzyme B was 0.005 mg/ml pRBCs. For A2B, the enzyme A was 0.004 mg/ml pRBCs, and enzyme B was 0.005 mg/ml pRBCs. Considering A1B or A2B was not identified in clinical transfusion, to convert A1B and A2B to O RBCs completely, the dose of enzyme A and B was required as 0.015 mg/ml pRBCs and 0.005 mg/ml pRBCs.
Figure 3. FACS of native and ECO RBCs. The x-axis represents the fluorescence intensity on a logarithmic scale whereas the y-axis shows the number of RBCs evaluated. Red color represents native RBCs; blue color represents enzyme-treated RBCs; pink color represents O-RBCs. A-a,B-a: A1B-RBCs, A2B-RBCs and A1B, A2B -ECORBCs were labeled by anti-A antibody, A antigen epitopes were detected in A1B and A2B –RBCs, but not found in A1B and A2B –ECORBCs and O-RBCs. A-b,B-b: A1B-RBCs, A2B-RBCs and A1B, A2B -ECORBCs were labeled by anti-B antibody, B antigen epitopes were detected in A1B and A2B –RBCs, but not found in A1B and A2B –ECORBCs and O-RBCs. A-c,B-c: A1B-RBCs,A2B-RBCs and A1B, A2B -ECORBCs were labeled by UEA1, H antigen epitopes were detected in A1B, A2B -RBCs, A1B, A2B –ECORBCs and O-RBCs.
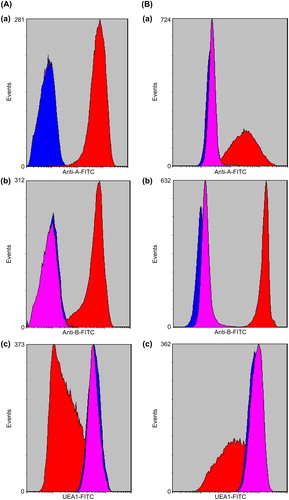
Finally, enzymatic conversion reactions were performed in 1 ml reaction mixtures containing 250 mM glycine, pH 6.8 and 3 mM NaCl with 40% packed red blood cells and 0.015 mg enzyme A and 0.005 mg enzyme B. The conversion reaction was incubated for 60 minutes with gentle mixing at 26°C, followed by four repeat washing cycles with 1:4 (v/v) of PBS. The washed enzyme-converted RBCs were stored in monoammoniumphosphate (MAP) nutrient solution with 70–75% hematocrit at 4°C. In these reaction mixtures, the amount of PCS carried into was less than 0.2%, it was confirmed that this concentration does not affect the group conversion. The residual enzyme load associated with washed cells was consistently less than 10 ng/ml of packed RBCs as measured with a sensitive capture enzyme-linked immunosorbent assay using total detergent lysates of cells. RBCs from more than 20 group A1B and A2B donor units have been analyzed and all were converted efficiently. In addition, the enzymatic conversion process does not affect physiologic and metabolic parameters of RBCs, including osmotic fragility, levels of 2,3-DPG, ATP, deformability acetylcholinesterase and methemoglobin (data not shown).
Discussion
Development of practical enzymatic converting universal blood could improve blood shortage situation and avoid the occurrence of accidental transfusion of ABO- incompatible red blood cells in clinic. The study was pioneered by Goldstein and colleagues at the New York Blood Center in the early 1980s (Goldstein et al. Citation1982, Goldstein Citation1989). But due to the low activity and high cost of recombinant coffee bean α-galactosidase, the clinical application of BECORBCs was hampered. So far, the reason is mainly due to the lack of appropriate glycosidase and the more complexity of A antigen, enzymatic conversion of group A to O RBCs has lagged behind. After more than 20 years of studies, α-N- acetylgalactosaminidase and α-galactosidase from bacteria were identified recently (Liu et al. Citation2007, Citation2008). These molecules have high efficient and selective characters and they could convert group A or B to O RBCs in neutral pH conditions in a short time at room temperature, respectively. This discovery may make, the ECORBCs study intensive around the world with a potential clinical application. So far, the technology to convert the blood group A and B to O RBCs has been well established.
In our study, we have found that A-zyme and B-zyme have the activities in which GalNAc-pNp or Gal-pNp act as major substrate. We have studied the stabilities of α- N-acetylgalactosaminidase or α-galactosidase in PCS and confirmed they have a basic effect of enzyme catalytic on GalNAcα-pNp or Gal-pNp. However, the structure of blood type antigen is more complicated than that of GalNAcα-pNp and Gal-pNp. The results of this type of activity could not reflect the efficiency of antigen epitope removal of enzyme A and B. So the conversion efficiency of enzyme A and B was evaluated by their abilities of removing antigen epitope from A or B RBCs by FACS.
The results showed that A-zyme and B-zyme were stable in PCS buffer, so PCS was an optimal buffer for enzyme A and B preserving. AB to O conversion is a part of ECORBCs’ study, and it needs both enzymes A and B. In theory, if we mix A-zyme and B-zyme together, the AB to O conversation should be achieved. But in fact, our experiments showed different results. First, we found that A-zyme's and B-zyme's preserving buffer has too high iron intension to manage their optimal permission in the reaction buffer. Second, A RBCs have two sub-groups with different antigen characters, so the doses of enzyme for A1 group and A2 group would be different.
No method about AB to O conversion in one system has been reported yet, and no report about optimal preserving system of enzyme A or B has been published either. Our previous studies indicated that glycine buffer was the catalytic reaction buffer of A-zyme and B-zyme, but did not suit for enzyme preserving. The optimal preserving buffer of A or B-zyme was PCS. A-zyme and B-enzyme were preserved in PCS. Based on the fact that components are different between preserving and reaction buffer of A-zyme and B-zyme, we began to investigate the conversion efficiency of two enzymes in PCS buffer and glycine buffer. In the series of buffer studies, we found that PCS buffer negatively affected the conversion efficiency of AB to O conversion. Furthermore, we detected that the reason of conversion efficiency of A-zyme and B-zyme decreased in PCS was that their binding capacity to RBCs decreased. So we compared the A-zyme and B-zyme conversion efficiency in glycine system with different PCS amount. Finally, we determined that PCS permission should be less than 10% of the reaction volume. In fact, the amount of PCS carried into was less than 0.2%, so it was confirmed that this concentration does not affect the group conversion.
Our data provide a new protocol for converting blood type AB to O with potential clinical applications. Especially, α-N-acetylgalactosaminidase and α-galactosidase are both stable in high iron intensity condition at 4°C.
Declaration of interest
The authors report no conflicts of interest. The authors alone are responsible for the content and writing of this paper.
References
- Clausen H, Levery SB, Kannagi R, Hakomori S. 1986. Novel blood group H glycolipid antigens exclusively expressed in blood group A and AB erythrocytes (type 3 chain H). J Biol Chem. 261:1380–1387.
- Clausen H, Levery SB, Nudelman E, Tsuchiya S, Hakomori S. 1985. Repetitive A epitope (type 3 chain A) de&UnknownEntity2;ned by blood group A1-speci&UnknownEntity2;c monoclonal antibody TH-1: chemical basis of qualitative A1 and A2 distinction. Proc Natl Acad Sci USA. 82:1199–1203.
- Daniels G, Withers SG. 2007. Towards universal red blood cells. Nat Biotechnol. 25:427–428.
- Gao HW, Li SB, Bao GQ, Tan YX, Wang YL, Zhang YP, . 2011a. Properties of a novel α-galactosidase from B. Fragilis and its potential for human blood-type B to O conversion. Scientia Sinica Vitae. 41:1030–1036.
- Gao HW, Li SB, Bao GQ, Tan YX, Wang LY, Jin SH, . 2011b. A reconstructed B. Fragilis-derived recombinant α-galactosidase developed for human blood type B→O conversion. Zhongguo Shi Yan Xue Ye Xue Za Zhi. 19:503–507.
- Goldstein J, Siviglia G, Hurst R, Lenny L. 1982. Group B erythrocytes enzymatically converted to group O survive normally in A, B, and O Individuals. Science. 215:168–170.
- Goldstein J. 1989. Conversion of ABO blood groups. Transfus Med Rev. 3:206–212.
- Kruskall MS, AuBuchon JP, Anthony KY, Herschel L, Pickard C, Biehl R, . 2000. Transfusion to blood group A and O patients of group B RBCs that have been enzymatically converted to group O. Transfusion. 40:1290–1298.
- Lenny LL, Hurst R, Goldstein J, Benjamin LJ, Jones RL. 1991. Single-unit transfusions of RBC enzymatically converted from group B to group O to A and O normal volunteers. Blood. 77:1383–1388.
- Lenny LL, Hurst R, Goldstein J, Galbraith RA. 1994. Transfusions to group O subjects of 2 units of red cells enzymatically converted from group B to group O. Transfusion. 34:209–214.
- Liu QY, Sulzenbacher G, Yuan H, Bennett EP, Pietz G, Saunders K, . 2007. Bacterial glycosidases for the production of universal red blood cells. Nat Biotechnol. 25:454–464.
- Liu QY, Yuan H, Bennett EP, Levery SB, Nudelman E, Spence J, . 2008. Identification of a GH110 Subfamily of 1,3-Galactosidases:novel enzymes for removal of the alpha 3Gal xenotransplantation antigen. J Bio Chem. 283:8545–8554.
- Yu CY, Xu H, Wang LS, Zhang JG, Zhang YP. 2008. Human RBCs blood group conversion from A to O using a novel alpha-N- acetylgalactosaminidase of high specific activity. Chin Sci Bull. 53:2008–2016.