Abstract
Metal-on-metal hip resurfacing arthroplasties represent an alternative to total hip arthroplasties for young and active patients, enabling the preservation of intact femoral bone and therefore improving the prognosis for future hip joint replacements. Follow-up studies have shown that the main reasons for early implant failure are mal-orientation of the implant stem in relation to the femoral neck axis, and notching of the femoral neck during femoral head preparation, as well as by exposed cancellous bone after implantation. A computer-assisted planning and navigation system for the implantation of femoral hip resurfacing implants has been developed which supports the surgeon during intraoperative fluoroscopy-based planning and navigation of implant positioning. This paper presents the results of a cadaver study performed to evaluate the system's functionality and accuracy.
Introduction
Hip resurfacing involves the removal and replacement of the surface of the femoral head with a hemispherical metal shell that fits into a metal acetabular cup. One of the claimed advantages of this technique over total hip arthroplasty (THA) is its ability to preserve femoral bone and therefore improve the outcome of future hip replacements Citation[1]. Other advantages, related to the implant design, are better postoperative joint stability, better kinematical properties, and low rates of articular bearing wear Citation[2–4].
In general, the hip resurfacing implantation technique is more complex in comparison to THA. The final implant position depends on the placement of a guide pin that is conventionally placed using a mechanical alignment instrument. This crucial step in the implantation procedure is quite time-consuming and requires much experience as well as careful handling of the alignment device. For example, Mont et al. Citation[5] reported 13 early implant failures (including 11 femoral neck fractures) among their first 50 cases but only one implant failure in the next 150 cases (follow-up range: 2 to 4 years).
In contrast, other follow-up studies (mean duration: 3.5 to 5.5 years) have shown that in conventional clinical practice hip resurfacing arthroplasties show good implant survival rates of 94–99%. However, the results of these studies also indicated that, in order to prevent femoral neck fracture or limitations affecting the patient's range of motion, it is important to position the implant in a neutral or slightly valgus orientation relative to the neck axis and avoid femoral neck notching or other cortical damage Citation[4], Citation[6–10].
lists the different computer-assisted approaches for planning and navigation of hip resurfacing arthroplasties that are already on the market, as well as those systems that will be introduced soon. So far, only a few clinical studies have reported on the accuracy and clinical benefits of computer-assisted hip resurfacing systems Citation[11], Citation[15]. The “image-less” approach is the most common, being based purely on optical tracking systems and some a priori model knowledge. A possible reason for its wide acceptance could be that, for these types of system, the costs in terms of radiation exposure and time can be reduced in comparison to CT- or fluoroscopy-based approaches. All the image-less systems listed in (e.g., the BrainLAB VectorVision® hip SR) propose specific procedures based on extensive mechanical palpation of the femoral neck in order to avoid femoral neck notching Citation[12–14]. However, this extensive femoral neck registration hinders minimal access surgery in comparison to the non-invasive registration procedures of fluoroscopy-based systems. Hess et al. reported good results in 31 cases (with less than two years of follow-up) using the fluoroscopy-based system by Medtronic® Citation[11], Citation[12]. Yet, in contrast to the image-less approaches, this system provides no specific procedure for avoiding femoral neck notching other than visual control by means of intraoperatively acquired X-ray images.
Table I. Computer-assisted hip resurfacing systems currently on the market.
In the field of research on computer-assisted hip resurfacing, several other approaches have been proposed Citation[16–19]; these share the pros and cons of the systems already on the market, as described above.
To support the surgeon in the planning and implantation of a femoral resurfacing prosthesis, a new computer-assisted planning and navigation system, based on optical tracking as well as on intraoperative calibrated X-ray images, has been developed Citation[20]. The system has been implemented on the basis of a navigation module library from SurgiTAIX AG (Aachen, Germany). As X-ray controls are commonly used in conventional procedures, fluoroscopic imaging induces no additional costs. The objective of our approach using calibrated multiplanar fluoroscopy in combination with adaptive model-based planning tools was to increase the reliability of the planning result with an optimal alignment of the implant while reducing the surgical access and the necessity for direct mechanical palpation of the femoral neck area. Concurrently, minimal access surgery should not increase the intraoperative efforts related to cost, time and radiation exposure. This paper presents the results of a cadaver study on the functionality and accuracy of our approach.
Methods
For the evaluation of the system, 10 hips of Thiel-fixed cadaveric full-body specimens were used. The intraoperative planning and implantation of femoral hip surface prostheses under navigation control was performed in the lateral position via a posterior approach using the hip resurfacing instrumentation and implants from Implantcast (Implantcast GmbH, Buxtehude, Germany). After fixation of the dynamic reference base (DRB) on the proximal part of the femur (), the surgeon executed the following five planning and navigation steps:
Step 1: Field of view registration
To enable intuitive, image-guided freehand navigation of the guide pin during the navigation step, the surgeon has to define the field of view (FOV) determining the reference frame of his/her eye-hand coordination. Therefore, transcutaneous palpation of three points on the femur is necessary. The lateral as well as the medial epicondyle and the greater trochanter are used to compute the eye-hand coordinate transformation for an intuitive representation of the tracked tools during guide pin navigation (step 5) (). Additionally, during the image acquisition step, these points are used for non-rigid point-to-point registration of a simplified shape model necessary for the so-called “zero-dose” C-arm navigation (SurgiTAIX AG, Aachen, Germany) Citation[21]. The third computation on the basis of the three registration points is the determination of the frontal and lateral planes required during the image acquisition step.
Step 2: Intraoperative X-ray image acquisition
For the intraoperative planning of the implant position, at least two calibrated X-ray images have to be acquired (e.g., anterior-posterior and “Lauenstein”). Here, the surgeon is supported by the zero-dose C-arm navigation. This tool enables a virtual radiation-free preview of the X-ray images as well as the actual orientation of the C-arm relative to the predefined (step 1) frontal and lateral planes. Thus, the acquisition of useless or suboptimal image projections can be avoided. This simple C-arm navigation method substantially reduces time and costs with respect to operation time and radiation exposure for the patient and the OR team ().
Figure 2. Image acquisition with the zero-dose C-Arm navigation. (a) Preview of frontal view (acquired image in the background). (b) Preview of lateral view prior to second image acquisition. [Color version available online.]
![Figure 2. Image acquisition with the zero-dose C-Arm navigation. (a) Preview of frontal view (acquired image in the background). (b) Preview of lateral view prior to second image acquisition. [Color version available online.]](/cms/asset/69d7367f-cced-4bca-be72-b7a5542d6194/icsu_a_233514_f0002_b.gif)
Step 3: Safe zone definition
The definition of the 3D “safe zone” helps the surgeon to safely plan the final position of the prosthesis during the implant alignment step (step 4). Exceeding this zone could lead to femoral neck notching or cortical damage. Therefore, definition of the safe zone is a crucial step with regard to the success of the intervention, and can be divided into two parts. One part is the non-invasive approximation of the femoral neck anatomy using an adaptable cylinder (). Here, the surgeon has to position and scale the cylinder via a touch-screen so that in every acquired X-ray image the cylinder's position is completely inside the femoral neck. Zimolong et al. indicated that femoral neck perforations could be avoided by using a cylinder for femoral neck modeling during the planning procedure Citation[22].
Figure 3. (a) Safe zone definition. (b) Warning during implant alignment. (c) Final implant position. (d) Offset between planning and result. [Color version available online.]
![Figure 3. (a) Safe zone definition. (b) Warning during implant alignment. (c) Final implant position. (d) Offset between planning and result. [Color version available online.]](/cms/asset/26aa76d0-1601-4b23-8b8d-6f58cc6b9731/icsu_a_233514_f0003_b.gif)
The second part of safe zone definition is the optional registration of critical areas on the femoral neck that could lead to femoral neck notching ().
Step 4: Implant alignment
To plan the final implant position, 3D models as well as profile models of the hip resurfacing from an implant database are matched to the fluoroscopic images. The surgeon is able to change the transparency of these models to better recognize the contours of the joint in the X-ray images. To avoid femoral neck damage, the implant position must be aligned within the predefined safe zone. The system indicates any intersection between the implant model and the safe zone cylinder, as well as any intersection between the inner radius of the respective femoral head reamer and the registered notching areas (). The goal of this planning step is to find a valid implant position with respect to the general boundary conditions, including a slight valgus orientation of the implant axis as well as a good coverage of the femoral head, without leaving the safe zone ().
Step 5: Navigation of guide pin
Once the surgeon is satisfied with the planned implant position, he can continue with the image-guided freehand navigation of the guide pin. An optical tracking system is used which permanently tracks the position of the drilling machine. During the navigation step, the surgeon is supported by a compensatory display incorporating two cross-hair-like indicators representing the tool orientation (). Additionally, an X-ray projection with an overlay display of the navigated tool position enables plausibility checking. As the surgeon normally has the option to acquire more than two images for implant position planning, which generally increases the accuracy of the planning steps (steps 3 and 4), it appears logical that a purely fluoroscopic navigation would no longer be practicable. For a better adaptation of the navigation step to the preferences of the surgeon, the cross-hair navigation offers the possibility of changing the sensitivity of the display scale.
Results
In this study, 10 femoral heads were resurfaced. The main objective was to evaluate the general system accuracy, as represented by the difference between the implant position plan and the final implant position (). Hence, after each implantation, two additional X-ray images were acquired (frontal view and Lauenstein) and the 3D shape model of the implant was aligned to the implant in both of these X-ray images. Afterwards, the position of this alignment was compared to the previously planned implant position.
The mean distance error between planning and navigation was 3.2 mm (SD: 1.7 mm; minimum: 0.7 mm; maximum: 6.7 mm).
The mean angulation error was 2.4° (SD: 0.9°; minimum: 1.0°; maximum: 3.6°).
The mean time for all five planning and navigation steps was 17 minutes (SD: 6 min; minimum: 8 minutes; maximum: 28 minutes).
For further evaluation of the implant placement with respect to the femoral neck, the proximal parts of all femora were osteotomized and CT scans of each specimen were acquired. shows the results of the evaluation of the implant axis alignment in relation to the femoral neck margins.
Figure 5. CT evaluation: Implant axis alignment (frontal and lateral view) in relation to femoral neck margins.
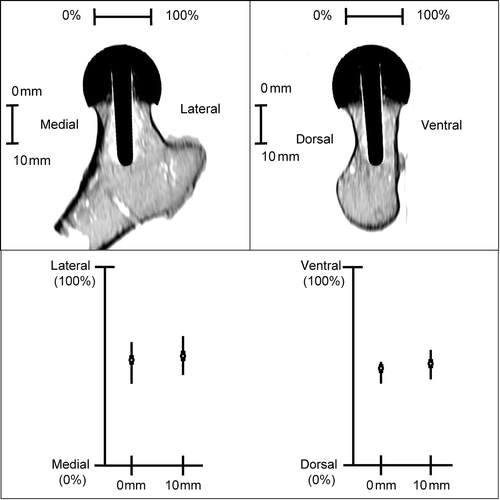
On the basis of conventional X-ray images as well as visual observation and CT scans, it was found that in one case the femoral neck was notched during neck reaming (). In two cases the implant position was too far cranial, and in another case too far dorsal. This implies that the fit of the metal shell of the implant in these probes is slightly unsymmetrical with respect to the femoral neck. In all cases, the implant alignment was neutral relative to the femoral neck axis. Apart from the notched probe, no other cortical damage occurred during this study.
Discussion
The evaluation of the system demonstrated the feasibility of fluoroscopically navigated implant placement. In contrast to image-less navigation approaches based on mechanical palpation of the femoral neck, our approach potentially supports minimal access surgery by means of non-invasive registration of the femoral neck based on X-ray projections and adaptive geometric modeling. As a result of this study, the maximum time required for all five planning and navigation steps, including the acquisition of the X-ray images, was 28 min. Taking into account the fact that the operating surgeon had no prior experience with the system being evaluated, this seems to be a remarkable result. Fluoroscopic image acquisition is efficiently supported by zero-dose C-arm navigation. The image acquisition procedure was optimized in such a way that the image quality could be maximized, while simultaneously minimizing both radiation exposure and time required.
However, it was found that the overall system accuracy was not as good as that already reported in a previous in-vitro study Citation[20]. One reason for this seemed to be the navigation of the guide pin (3 mm in diameter). During the navigation step, the pin was drilled directly into the femoral head without the use of a navigated sleeve. The pin could therefore bend, possibly resulting in an increase in the planning-implantation offset. A further crucial factor affecting the accuracy of the navigation step might be the use of the conventional instrumentation for femoral head preparation. As the system only provides navigational control for the initial guide-pin drilling, unintended drifting of the hollow drill or reamer during femoral head preparation would go unnoticed. Extension of the navigation step to include all conventional steps of femoral head preparation may also decrease the planning-implantation offset. Furthermore, such an inclusion would offer the opportunity to align the implant in five instead of only four degrees of freedom under navigational control.
Other sources of error are the accuracy of the X-ray image calibration, as well as that of the optical tracking system, and these aspects are the subject of further investigations. Various optical tracking systems are currently being tested under different boundary conditions, and the results of this study should help to increase the reliability and accuracy of optical tracking use. For the improvement of the calibration algorithm, we have already investigated other calibration principles that have yielded better results than the algorithm used in the cadaver study.
A further aspect that is solved neither in our approach nor in other navigational optical-tracking-based approaches is the necessity of fixing the dynamic reference base to the bone. Future developments should concentrate on the development of fixation methods that provide a rigid but minimally invasive fixation on the bone.
In general, the experience with the presented system was good, especially from the perspective of a possible integration of this navigation system into the clinical workflow. Future studies are planned to evaluate the performance of an improved version of the system in comparison to conventional hip resurfacing arthroplasty.
Acknowledgment
This work has been funded in part by the German Federal Ministry of Education and Research (BMBF) within the framework of the research program SOMIT “Sanftes Operieren mit innovativer Technik” through grants BMBF 01EQ0402, BMBF 01IBE 02A-02D, and BMBF 16SV2019.
References
- National Institute for Clinical Excellence. Guidance on the use of metal-on-metal hip resurfacing arthroplasty. National Institute for Clinical Excellence;, London 2002
- Beaule PE, Amstutz HC, Le Duff M, Dorey F. Surface arthroplasty for osteonecrosis of the hip: Hemisurfacing versus metal-on-metal hybrid resurfacing. J Arthroplasty 2004; 19(8 Suppl. 3)54–58
- Treacy RB, McBryde CW, Pynsent PB. Birmingham hip resurfacing arthroplasty; a minimum follow-up of five years. J Bone Joint Surg Br 2005; 87(2)167–170
- McMinn DJ, Treacy RB, Lin K, Pynsent PB. Metal-on-metal surface replacement of the hip. Experience of the McMinn prosthesis. Clin Orthop Rel Res 1996, 329 Suppl: 89–98
- Mont MA., Ragland P, Bezweda H, Thomas CM., Etienne G. The results of metal-on-metal resurfacing hip arthroplasty: Learning curve stratification of results. Presentation at the American Academy of Orthopaedic Surgeons. Washington, DC February 2005
- Amstutz HC, Beaule PE, Dorey FJ, Le Duff MJ, Campbell PA, Gruen TA. Metal-on-metal hybrid surface arthroplasty: Two to six-year follow-up study. J Bone Joint Surg Am 2004; 86: 28–39
- Amstutz HC, Campbell PA, Le Duff MJ. Fracture of the neck of the femur after surface arthroplasty of the hip. J Bone Joint Surg Am 2004; 86: 1874–1877
- Daniel J, Pynsent PB, McMinn DJ. Metal-on-metal resurfacing of the hip in patients under the age of 55 years with osteoarthritis. J Bone Joint Surg Br 2004; 86(2)177–184
- Beaule PE, Lee JL, Le Duff MJ, Amstutz HC, Ebramzadeh E. Orientation of the femoral component in surface arthroplasty of the hip. A biomechanical and clinical analysis. J Bone Joint Surg Am 2004; 86: 2015–2021
- Shimmin AJ, Back D. Femoral neck fractures following Birmingham hip resurfacing: A national review of 50 cases. J Bone Joint Surg Br 2005; 86-B: 463–464
- Hess T, Gampe T, Köttgen C, Szawlowski B. Intraoperative navigation for hip resurfacing. Methods and first results [in German]. Der Orthopäde 2004; 33(10)1183–1193
- Hess T, Henke H, Gampe T. Der navigierte Oberflächenersatz am Hüftgelenk – Entwicklung. Anwendung, Erfahrungen 2006, http://www.ortho24.com
- Müller H, Bracke B, Dick R. Automatic detection of femoral neck axis for hip resurfacing surgeries. Proceedings of the 5th Annual Meeting of the International Society for Computer Assisted Orthopaedic Surgery (CAOS-International 2005), F Langlotz, BL. Davies, D Schlenzka, HelsinkiFinland June 2005; 326–328
- Springorium HP. Entwicklung der Navigation beim Oberflächenersatz der Hüfte. Z Orthop Ihre Grenzgeb 2006; 144: 129–131
- Sandhu HS., Middleton RG. Hip resurfacing with computer navigation. Initial experience at the Royal Bournemouth Hospital, UK. Proceedings of the 5th Annual Meeting of the International Society for Computer Assisted Orthopaedic Surgery (CAOS-International 2005), F Langlotz, BL. Davies, D Schlenzka, HelsinkiFinland June 2005; 403–405
- Hodgson AJ, Inkpen KB, Shekhman M, Anglin C, Tonetti J, Masri BA, Duncan CP, Garbuz DS, Greidanus NV. Computer-assisted femoral head resurfacing. Comput Aided Surg 2005; 10(5/6)337–343
- Inkpen KB., Shekhman M, Anglin C, Greidanus NV., Tonetti J, Masri BA., Hodgson AJ., Duncan CP., Garbuz DS. Computer-assisted femoral head resurfacing. Proceedings of the 5th Annual Meeting of the International Society for Computer Assisted Orthopaedic Surgery (CAOS-International), F Langlotz, BL. Davies, D Schlenzka, HelsinkiFinland June 2005; 183–185
- Barrett ARW., Cobb JP., Jalopec M, Harris SJ., Rodriguez y Baena FM., Davies BL. The Tubes™ for minimally invasive computer-assisted hip resurfacing surgery. Proceedings of the 5th Annual Meeting of the International Society for Computer Assisted Orthopaedic Surgery (CAOS-International 2005), F Langlotz, BL. Davies, D Schlenzka, HelsinkiFinland June 2005; 22–25
- Long WJ., Rudan J, Ellis RE. Computer aided hip resurfacing arthroplasty. Proceedings of the 5th Annual Meeting of the International Society for Computer Assisted Orthopaedic Surgery (CAOS-International 2005), F Langlotz, BL. Davies, D Schlenzka, HelsinkiFinland June 2005; 280–282
- Schkommodau E, de la Fuente M, Froebe S, Wirtz DC., Portheine F, Radermacher K. A new approach for planning surface replacement prosthesis surgery on the hip. Proceedings of the 4th Annual Meeting of the International Society for Computer Assisted Orthopaedic Surgery (CAOS-International 2004), F Langlotz, BL. Davies, SD Stulberg, Chicago, IL June 2004; 310–311
- de la Fuente M, Belei P, Wirtz DC., Portheine F, Wirtz DC., Radermacher K. Zero-dose C-arm navigation reducing intraoperative radiation. Proceedings of the 5th Annual Meeting of the International Society for Computer Assisted Orthopaedic Surgery (CAOS-International 2005), F Langlotz, BL. Davies, D Schlenzka, HelsinkiFinland June 2005; 83–84
- Zimolong A, Portheine F, Friedrichs D, Traub F, Radermacher K. Evaluation of deformable models for femoral neck surgery. Comput Aided Surg 2004; 9(3)71–79