Abstract
Computer aided musculoskeletal tumor surgery is a novel concept. Ideally, computer navigation enables the integration of preoperative information concerning tumor extent and regional anatomy to facilitate execution of a surgical resection. Accurate resection helps oncological clearance and facilitates precise fitting of a custom prosthesis. We adapted a commercially available computer navigation platform for spine, and used it to plan and execute pelvic bone resection and custom pelvic prosthetic reconstruction in a patient with a metastatic tumor affecting the acetabulum. The surgery was simulated and validated using a plaster bone model based on the patient's preoperative CT data, before performing the procedure on the patient.
Introduction
Functionally rewarding results following limb salvage surgery in patients with primary tumors of the extremities depend largely upon near-anatomic restoration of function using megaprostheses Citation[1–3]. Such an approach is also being adopted in the management of pelvic tumors Citation[4–6]. While modular prostheses are available for extremity reconstruction, the complex geometry of the pelvis and acetabulum has restricted the development of such an alternative option for peri-acetabular reconstructions. The restoration of a near-anatomic hip articulation using custom pelvi-acetabular prostheses fabricated specifically for the individual patient undergoing a Type II resection is one of the acceptable methods of reconstruction within this difficult region. It can preserve normal leg length and promote early functional recovery Citation[7].
The anatomic extent of the tumor is first determined using CT and MR images, and an oncologically acceptable level of resection is planned. The patient's preoperative CT data is used to design and manufacture the prosthesis, with the expectation that it will fit the remnant of the pelvis at the determined level of resection. While advances in computer aided design (CAD) and manufacturing technology have enabled rapid prototyping of custom prostheses with a high degree of accuracy, achieving precision in surgical resection is fraught with difficulties. In pelvic tumor surgery, the complex geometry of the pelvic anatomy makes it difficult to perform a bony resection with the particular desired orientation that is crucial to the accurate fitting of the custom prosthesis. Indeed, a high degree of precision in resection of the bone is necessary, given that the prosthesis is made to exact dimensions. Errors in the plane of resection may result in unsatisfactory resection margins and failure of fixation to the remaining pelvic bone. The ensuing mismatch at the bone-prosthesis junction is likely to affect the stability and functional outcome of the reconstruction.
Computer navigation has been effectively used in the treatment of orthopaedic trauma Citation[8], spinal procedures Citation[9], Citation[10] and joint replacement surgery Citation[11], Citation[12], and hardware–software solutions catering to each of these disciplines are commercially available. Use of computer navigation has improved surgical precision, enabling accurate placement of screws and prosthetic devices Citation[9–13].
We have been using custom pelvic prosthetic reconstruction in patients with primary or metastatic tumors affecting the para-acetabular region. Difficulties in achieving the desired precision in bone resection and in visualizing the intraosseous extent of the tumor during surgery led us to consider using computer navigation to facilitate such surgery.
There is no commercial navigation prototype available for pelvic bone resection, and we describe here how a commercially available navigation platform for the spine was adapted for use in the planning and execution of pelvic bone resection in a patient with a metastatic tumor affecting the acetabulum. This adaptation was validated by simulating the surgery on a plaster bone model based upon the patient's preoperative CT data, before using it successfully to resect the actual tumor.
Case report
A 47-year-old Chinese male was diagnosed with rectal carcinoma in 1998. He underwent an abdomino-perineal resection and was given postoperative chemotherapy and radiotherapy. He developed left hip pain in 2005 and was unable to bear weight on the left side. Radiological investigations of the pelvis showed an osteolytic destruction of the left acetabulum ( and biopsy revealed a metastatic adenocarcinoma, most likely from the rectum. Staging investigations revealed localized disease. The patient did not respond to radiotherapy and continued to experience severe pain. To allow symptom relief and immediate postoperative walking, limb salvage following pelvic resection followed by prosthetic reconstruction was considered.
Figure 1. Plain radiograph (A), CT scan (B) and MRI scan (C) of patient's pelvis, showing a destructive bony metastasis involving the left acetabular region.
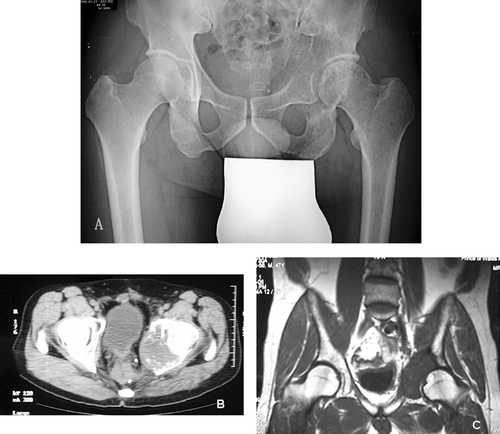
Design of the custom prosthesis
CT and MR studies of the pelvis were performed to determine the appropriate level of resection of the ilium. CT images annotated with respect to the resection level were sent to Stanmore Implants Worldwide (SIW) (Stanmore, England) to enable the manufacture of a CAD custom pelvic prosthesis. An appropriate implant was designed by the SIW design team using CAD software (). The prosthetic design comprised an acetabular metal socket component and a body section that was to be attached to the remaining ilium using two intramedullary titanium pins. Hydroxyapatite coating was added at the bone implant junction of the body sections to facilitate osseous integration. A constrained acetabular polyethylene liner was secured to the metal pelvic cup with bone cement, and a cemented femoral stem with a 32-mm femoral head was used for the femoral replacement.
Figure 2. Computer-aided design custom pelvic prosthesis for skeletal reconstruction after periacetabular tumor resection. [Color version available online.]
![Figure 2. Computer-aided design custom pelvic prosthesis for skeletal reconstruction after periacetabular tumor resection. [Color version available online.]](/cms/asset/58fa0e0f-ac72-416a-bb2e-1a4eb2fa1aa6/icsu_a_253465_f0002_b.gif)
The custom pelvic prosthesis dataset was visualized using CAD software (MIMICS® – Materialise's Interactive Medical Image Control System) that allowed the surgical team to understand the desired orientation of the bone resection. This was to ensure that the superior surface of the prosthesis would fit precisely to the opposing cut surface of the ilium during the surgery.
Surgical navigation and preoperative simulation
The success of surgical navigation depends not only upon the ability to transfer preoperative information regarding the tumor location and the plane and dimensions of the resection, but also on using it in real time for the execution of the surgery.
The computer aided operation was performed using standard spine navigation software, as provided with the Stryker Navigation system (CT spine, Version 1.60). Because no specific software application has yet been developed for tumor resection, we used one developed originally for the spine (for pedicle screw placement). Likewise, there were no specific instruments for precision marking, and we attached the navigation tracker to a conventional diathermy handle and used the needle point as an “end-effector”. All navigation procedures were practiced and validated on a plaster pelvic model. The MIMICS software was used to transform DICOM data of the patient's preoperative CT images to a file format which was compatible with the model fabrication system. An accurate and solid plaster model was then generated by rapid prototyping techniques.
The dataset of the custom pelvic prosthesis design was in CAD format. Distances between specific identifiable bony landmarks and the resection level were measured using the MIMICS software (). A 3D virtual pelvic model was constructed in the navigation system using preoperative CT data. As the spine navigation software did not provide direct planning of a plane or easy measurement of the distance between two bony points, the plane of the iliac osteotomy was defined and planned by using multiple virtual screws inserted at the selected bony points on the virtual CT pelvic images. Registration of the system was then performed to ensure correspondence between the virtual pelvic image and the plaster model. Four bony landmarks, easily located during surgery, were identified as paired points for matching. An additional 50 points for surface matching were selected along the acetabular rim. The mean error after paired-points registration was 2.3 mm, but decreased to 0.3 mm after surface matching. The resection level was defined upon identification of the position of the virtual screws under navigational guidance. The “tumor” was resected in the plaster model, and the cut surface of the ilium in the model was identical to that expected at the predetermined level on CAD data ( ). The prosthesis matched very well with the opposing surface of the remaining ilium, thus validating our use of computer navigation assisted pelvic tumor resection on the plaster model.
Figure 3. The most important and easily identifiable bony landmark was point 1, which was the intersection point between the acetabular rim and the anterior inferior iliac spine. Other points and measurements were all based on point 1. Point 3 and other points at the acetabular rim were arbitrary. The custom pelvic prosthesis was designed in such a way that orientation of the superior surface of the prosthesis is parallel to the ground when the patient is in a standing position. When a person stands upright, the plane formed by the two anterior superior iliac spines and pubic tubercles is perpendicular to the ground. Therefore, orientation of the prosthesis and bony resection could be defined and planned using this plane. Based on points 2 and 4 and the above information, the plane and level of bony resection could be defined with points 1–4 in the navigation planning. Other points and distances were used to verify the accuracy of this plane definition. [Color version available online.]
![Figure 3. The most important and easily identifiable bony landmark was point 1, which was the intersection point between the acetabular rim and the anterior inferior iliac spine. Other points and measurements were all based on point 1. Point 3 and other points at the acetabular rim were arbitrary. The custom pelvic prosthesis was designed in such a way that orientation of the superior surface of the prosthesis is parallel to the ground when the patient is in a standing position. When a person stands upright, the plane formed by the two anterior superior iliac spines and pubic tubercles is perpendicular to the ground. Therefore, orientation of the prosthesis and bony resection could be defined and planned using this plane. Based on points 2 and 4 and the above information, the plane and level of bony resection could be defined with points 1–4 in the navigation planning. Other points and distances were used to verify the accuracy of this plane definition. [Color version available online.]](/cms/asset/64f9b109-4d24-4c8a-8fbe-9d39b84fb6be/icsu_a_253465_f0003_b.gif)
Details of surgery
Angiography and embolization of feeding vessels was performed one day before the surgery. The patient was placed in the semi-lateral position, and an ilioinguinal approach with femoral extension was used. Femoral neurovascular bundles were identified and protected. Greater trochanteric osteotomy and detachment of muscle at the anterior superior iliac spine allowed adequate exposure of the ilium. The iliopsoas tendon and abductor muscles were preserved. A dynamic reference base tracker was attached to the iliac crest (, panel D). Registration was performed using a paired-points method based on the selected 4 bony landmarks and 50 surface matching points on the patient's pelvic bone. Verification was accurate with a mean error of 0.36 mm. A needle diathermy electrode with a navigation tracker was calibrated within the system. With the navigated diathermy electrode, the tumor extent at the superior pubic ramus and posterior column of the acetabulum was visualized in the virtual CT images displayed on the navigation monitor screen. Clear tumor margins were identified and marked simultaneously using the navigated diathermy electrode. The locations of the planned screws at the resection level were identified and marked (). These diathermy burn-in points were then joined to form the resection line at the iliac region. Three osteotomies were made in the pelvis, using an oscillating saw, and the tumor was removed. The total time spent on navigation procedures was 50 minutes. The custom pelvic prosthesis was then implanted and adapted well to the bone cut. A constrained acetabular liner was cemented into the prosthetic cup, then a cemented femoral stem was inserted, and muscle and greater trochanteric osteotomy were reattached accordingly.
The cross-section of the pelvic bone after iliac osteotomy was identical to that visualized on CAD images and to that achieved on the plaster bone model (). Histology confirmed that a tumor-free resection margin was achieved. A postoperative plain radiograph of the pelvis showed satisfactory prosthetic alignment ().
Figure 4. The cut surface of the ilium after pelvic resection under navigational guidance (A); after preoperative simulation of the pelvic resection in the plaster model (B); and at the predetermined level of pelvic resection on CAD data (C). [Color version available online.]
![Figure 4. The cut surface of the ilium after pelvic resection under navigational guidance (A); after preoperative simulation of the pelvic resection in the plaster model (B); and at the predetermined level of pelvic resection on CAD data (C). [Color version available online.]](/cms/asset/273b751e-52fa-488e-b5ba-088974a7ade6/icsu_a_253465_f0004_b.gif)
Figure 5. Intraoperative screen images on the navigation monitor. The location of the navigated diathermy electrode (white line) was seen in relation to the peri-acetabular tumor. The proximal margin and location of planned screws could be identified in coronal images (A), obliques images (B), and reconstructed three-dimensional images (C). In panel D, a dynamic reference base tracker is seen attached to the left iliac crest, with a navigated diathermy electrode being used to identify the virtual margin and the resection level on the navigation monitor. [Color version available online.]
![Figure 5. Intraoperative screen images on the navigation monitor. The location of the navigated diathermy electrode (white line) was seen in relation to the peri-acetabular tumor. The proximal margin and location of planned screws could be identified in coronal images (A), obliques images (B), and reconstructed three-dimensional images (C). In panel D, a dynamic reference base tracker is seen attached to the left iliac crest, with a navigated diathermy electrode being used to identify the virtual margin and the resection level on the navigation monitor. [Color version available online.]](/cms/asset/c161e679-a16e-4abb-b921-021267464ddf/icsu_a_253465_f0005_b.gif)
Figure 6. Postoperative plain radiograph showing satisfactory alignment of the custom pelvic prosthesis.
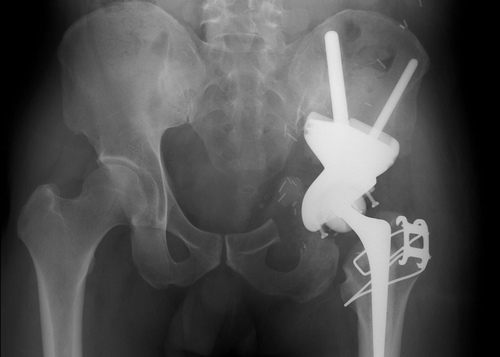
The postoperative course was uneventful, with no neurovascular complications or wound infection. The patient was allowed to walk, with protected weight-bearing and a hip brace, two weeks after surgery, and recovered good hip and abductor control by the fourth week. The brace was then discarded, and the patient could walk bearing his full weight. He could walk independently without aids at 3 months after surgery.
Discussion
Although computer navigation is popular in some areas of orthopaedic surgery, its use in musculoskeletal tumor surgery has been relatively limited.
The integration of preoperative planning and real-time generation of a virtual model during surgery is of particular value in planning the plane and location of osteotomies in regions of complex anatomy such as the pelvis. During the surgery, we were able to visualize and correctly identify the exact extent to which a tumor had infiltrated the pelvis. This ensured an oncologically safe resection as exact as that planned preoperatively. Such precision is difficult to attain without the benefit of accurate visualization of tumor extent. An accurate resection ensured correct seating of the custom prosthesis: The identical cross-sections of the osteotomy in the preoperative CAD model and of the resected specimen confirmed the feasibility of using navigation in pelvic tumor surgery.
Hüfner et al. Citation[14] successfully used a navigated chisel to identify tumor extent in the resection of sacral tumors in 3 patients, while Krettek et al. Citation[15] used computer aided pelvic tumor resection in 2 patients; one with a recurrent sacral chordoma and the other with a peri-acetabular chondrosarcoma. In these cases, the resection was facilitated by inserting K-wires under navigational guidance, in an appropriate direction, at the sites of planned bone resection. The tumor was then resected, and a custom-made pelvic prosthesis was manufactured according to a rapid prototype model made from the resected tumor specimen. The manufactured prosthesis was implanted one week after the first surgery.
Registration is a critical step in CT-based navigation. It forms a link between the preoperative imaging data, the patient's anatomy, and independent surgical devices used to identify anatomical landmarks Citation[16]. Resection and removal of a pelvic tumor requires extensive operative exposure, but this wide exposure makes the registration procedures (both paired-points matching and surface matching) simpler and more accurate. The surgeon must be aware that the instrument positions and real anatomy may differ from those shown in the navigation display, and repeated verifications may be necessary during navigation procedures. The trial and rehearsal of registration procedures in a plaster pelvic model helped to identify the most reliable bony locations for successful registration and greatly reduced the time spent on the intraoperative registration process.
The custom-made pelvic prosthesis is based on the patient's preoperative CT data and the predetermined resection level at the iliac region. The iliac osteotomy must be in the correct orientation in order to fit to the bone-implant junction of the prosthesis and accommodate the two intramedullary pins. The CAD dataset of the custom-made prosthesis could not be transferred to the navigation system directly because of general software incompatibility between CAD modeling software and the DICOM format. Therefore, an indirect method was used instead. The distances between the resection level and some bony points were initially measured on the CAD model, then marked on corresponding points on the virtual model generated in the navigation system. There is a possibility of errors arising during this manual step, so the introduction of software which can transfer key data from a CAD format to DICOM format is desirable.
A “navigation-enabled” oscillating saw would have made the plane of pelvic osteotomy easier to attain. However, a needle diathermy electrode was an acceptable adaptation. We found it convenient to calibrate and register the needle diathermy electrode in the navigation system. Also, the needle tip enabled easier and more precise location and marking of the desired points, and made registration easy and accurate. Unlike Krettek et al. Citation[15], we did not use K-wire insertion to define the resection plane because it was felt that it would be difficult to cut along a plane created by the insertion of two K-wires. A navigated saw or a cutting jig with a built-in cutting slot may enable accurate bony cuts along a desired plane under navigational guidance.
A dedicated navigation platform for musculoskeletal tumor surgery may need to incorporate the capabilities to (a) accept file formats containing CAD data of custom prostheses; (b) allow navigation of an osteotomy plane; and (c) use fusion of CT and MRI images for real-time navigation. Such a platform would merit application not only in difficult pelvic tumor surgery, but also in joint-sparing extremity resections for tumors and pelvic re-alignment osteotomies for dysplasias around the hip, amongst other indications.
Conclusion
Resection and reconstruction of malignant pelvic tumors is difficult and challenging. However, with the advances in computer aided design and modern manufacturing technology, it is possible to fabricate preoperatively a custom prosthesis for limb salvage of pelvic tumors. Computer navigation enabled the integration of preoperative information on tumor extent and regional anatomy to facilitate execution of the surgical resection using a virtual model. The planned resection was executed to perfection and an accurate fitting of the prosthesis was thus achieved. Special navigation software for musculoskeletal tumor surgery may help in attaining the level of precision in tumor resection that is required in areas of complex anatomy, and when a custom prosthesis is used for reconstruction of the skeletal defect.
Acknowledgments
We acknowledge the great assistance of Mr. Eric Wai-kin Ng and Mr. Keith Kam-shing Lee (ACAOS-ITAV team, Department of Orthopaedics and Traumatology, Prince of Wales Hospital, Hong Kong) in the setup of the navigation system and the documentation of the operative procedures. We also appreciate the assistance of Mr. Man-ho Lee (Technical officer, Department of Rapid Prototyping & Tooling Unit, Hong Kong Polytechnic University) in using CAD software and in fabrication of the plaster pelvic model.
References
- Ham SJ, Schraffordt Koops H, Veth RP, Van Hom JR, Molenaar WM, Hoekstra HJ. Limb salvage surgery for primary bone sarcoma of the lower extremities: Long-term consequences of endoprosthetic reconstructions. Ann Surg Oncol 1998; 5(5)423–436
- Mittermayer F, Krepler P, Dominkus M, Schwameis E, Slug M, Heinzl H, Kotz R. Long-term followup of uncemented tumor endoprostheses for the lower extremity. Clin Orthop Relat Res 2001; (388): 167–177
- Torbert JT, Fox EJ, Hosaljar HS, Ogilvie CM, Lackman RD. Endoprosthetic reconstructions: Results of long-term followup of 139 patients. Clin Orthop Relat Res 2005; 438: 51–59
- Aljassir F, Beadel GP, Turcotte RE, Griffin AM, Bell RS, Wunder JS, Isler MH. Outcome after pelvic sarcoma resection reconstructed with saddle prosthesis. Clin Orthop Relat Res 2005; 438: 36–41
- Cottias P, Jeanrot C, Vinh TS, Tomeno B, Anract P. Complications and functional evaluation of 17 saddle prostheses for resection of periacetabular tumors. J Surg Oncol 2001; 78(2)90–100
- Ozaki T, Hoffmann C, Hillmann A, Gosheger G, Lindner N, Winkelmann W. Implantation of hemipelvic prosthesis after resection of sarcoma. Clin Orthop Relat Res 2002; (396): 197–205
- Abudu A, Grimer RJ, Cannon SR, Carter SR, Sneath RS. Reconstruction of the hemipelvis after the excision of malignant tumor: Complications and functional outcome of the prosthesis. J Bone Joint Surg Br 1997; 79B: 773–779
- Grützner PA, Suhm N. Computer aided long bone fracture treatment. Injury 2004; 35(Suppl 1)S-A57–S-A64
- Gebhard F, Weidner A, Liener UC, Stöckle U, Arand M. Navigation at the spine. Injury 2004; 35(Suppl 1)S-A35–S-A45
- Hott JS, Deshmukh VR, Klopfenstein JD, Sonntag VK, Dickman CA, Spetzler RF, Papadopoulos SM. Intraoperative Iso-C C-arm navigation in craniospinal surgery: The first 60 cases. Neurosurgery 2004; 54(5)1131–113;, discussion 1136–1137
- Anderson KC, Buehler KC, Markel DC. Computer assisted navigation in total knee arthroplasty: Comparison with conventional methods. J Arthroplasty 2005; 20(7 Suppl 3)132–138
- Wixson RL, MacDonald MA. Total hip arthroplasty through a minimal posterior approach using imageless computer-assisted hip navigation. J Arthroplasty 2005; 20(7 Supp 3)51–56
- Stôckl B, Nogler M, Rosiek R, Fischer M, Krismer M, Kessler O. Navigation improves accuracy of rotational alignment in total knee arthroplasty. Clin Orthop Relat Res 2004; (426): 180–186
- Hüfner T, Kfuri Jr M, Galanski M, Bastian L, Loss M, Pohlemann T, Krettek C. New indications for computer-assisted surgery: Tumor resection in the pelvis. Clin Orthop Relat Res 2004; 426: 219–225
- Krettek C, Geerling J, Bastian L, Citak M, Rucker F, Kendoff D, Hüfner T. Computer aided tumor resection in the pelvis. Injury 2004; 35: S-A79–S-A83
- Simon DA, Lavallée S. Medical imaging and registration in computer assisted surgery. Clin Orthop 1998; 354: 17–27