Abstract
Objective: To determine if image-guided robotic stereotactic radiosurgery (IGR-SRS) by CyberKnife achieves acceptable local control in resectable but medically inoperable patients with non-small cell lung cancer (NSCLC) or pulmonary metastasis, and to evaluate control rates and toxicity.
Methods: Treatment details and outcomes were reviewed for 95 patients (age range 33–96 years) with 136 histologically proven cancers treated by IGR-SRS at the CyberKnife Center of Miami between March 2004 and March 2007. Tumor volumes ranged from 1.2 cc to 338 cc. Targeting was accomplished using combined skeletal alignment and real-time tracking via fiducials placed within the tumor. Total doses ranged from 15 to 67.5 Gy delivered in 1 to 5 fractions.
Results: Of the 95 patients treated, 78 (82%) are still alive at 1 to 36 months post-treatment. Nineteen patients have died, four from disease other than cancer progression. All patients but one achieved at least partial response to treatment and tolerated radiosurgery well. For the majority of our patients, fatigue had been the main side effect.
Conclusions: The delivery of precisely targeted high radiation doses with surgical precision to lung tumors in a hypo-fractionated fashion is feasible and safe. Image-guided robotic stereotactic radiosurgery (IGR-SRS) of lung tumors with the CyberKnife achieves excellent rates of local disease control with limited toxicity to surrounding tissues, and in many cases may be curative for patients for whom surgery is not an option.
Introduction
Achieving long-term survival in patients with non-small cell lung cancer (NSCLC) or pulmonary metastasis remains a difficult clinical challenge. Under our currently accepted standards of care, those patients who are not eligible for surgery are treated with irradiation or chemotherapy, either alone or in combination. However, there is little consensus on appropriate drugs, doses, or treatment schedules for either modality.
Since early NSCLC is not a systemic disease, high-dose precisely targeted radiosurgery may be an alternative approach to NSCLC, but its applicability has been limited by difficulties in targeting tumors that move with respiration. Several recently published series Citation[1–12] using stereotactic body radiotherapy (SBRT) have confirmed that dose escalation and hypofractionation can result in enhanced local tumor control with minimal untoward effects, if exposure to neighboring normal tissue and other critical structures is limited.
Historically, due to poor prognosis, radiotherapy has not been employed with curative intent for patients with pulmonary metastases. However, data exist to support aggressive local intervention for selected patients with pulmonary metastases. Reports of SBRT for treatment of lung tumors have included patients treated for metastatic disease. Dose fractionation and techniques employed for these patients were similar to those used for primary lung tumors.
Based on the encouraging results demonstrated by SBRT, the CyberKnife (Accuray, Inc., Sunnyvale, CA), an image-guided robotic stereotactic radiosurgery system (IGR-SRS) has been applied to both primary lung tumors and pulmonary metastases Citation[13]. CyberKnife's powerful robotic arms can position a lightweight x-band linear accelerator and deliver beams of 6 MV X-ray from many angles. The targeting accuracy is achieved through a combined orthogonal X-ray image guidance and optic real-time motion-tracking system (Synchrony). For tumor tracking purposes, radio-opaque markers (fiducials) are generally needed, though a recent technical development permits certain tumors to be treated without fiducials. The tracking technique is accurate to within 1.5 mm, allowing for tight planning treatment volume (PTV) margins, and thus minimizes the dose to the surrounding lung tissues and critical structures.
Methods
Patient selection and pretreatment evaluation
Prior to acceptance for treatment, each patient was evaluated by both a thoracic surgical oncologist and a radiation oncologist, and underwent a pretreatment workup consisting of a CT scan of the chest, abdomen, and pelvis; an integrated fluorodeoxyglucose (FDG) positron emission tomography (PET) scan combined in a PET-CT scan with standard uptake value (SUV) reported; pulmonary function tests (PFT); complete blood count (CBC) and chemistry; and screening for tumor markers such as carcinoembryonic antigen (CEA), if applicable. A fully informed consent and a release of information for research and publication were obtained prior to treatment.
All patients were determined to have a technically resectable lesion but were deemed ineligible for surgery if they lacked adequate respiratory reserve, or had severe cardiac dysfunction or chronic heart disease, pulmonary hypertension, advanced diabetes mellitus with severe end organ damage, vascular disease, general frailty, or severe cerebral disease. Tumors larger than 5 cm in diameter were excluded. We did not exclude patients receiving other forms of antineoplastic therapy such as chemotherapy, biological therapy, and vaccine therapy. On the contrary, radiosurgery was used to treat the local disease while all patients were evaluated by a medical oncologist to determine whether systemic therapy was indicated to control spread of the disease.
The dose with curative intent for early stage NSCLC is commonly 60 Gy delivered in three fractions. The associated biological equivalent dose (BED) is 120 Gy, assuming the α ratio of 20 Gy for NSCLC. Such a dose, if delivered to lesions in the central and hilar regions of the lung, may result in severe complications, therefore lesions within 2 cm of the proximal bronchial tree adjacent to the central chest were excluded from the series of early stage NSCLC with curative intent. Patients with evidence of mediastinal disease (mediastinal lymph nodes >1 cm and abnormal hilar or mediastinal FDG uptake observed on PET scan, SUV > 3) or with pleural or pericardial effusions, whether malignant or not, or pneumothorax were also excluded from the early stage NSCLC series.
For metastatic lung patients, the limiting factors are primarily tumor size and tumor location. Dose selections are first based on tumor histology and on the published experience of SBRT for NSCLC. A BED of 120 Gy or greater than 100 Gy is taken as a guideline to project the optimal ablative dose for complete local control of the metastatic tumors, using the best available α ratio per tumor specification. For example, for a tumor with an α/β ratio of 5 Gy, a dose scheme of 1200 cGy × 3 fractions would yield a BED of 122 Gy. The projected optimal dose might be compromised if the risk of exceeding normal tissue tolerance is present or the patient's physical condition is deemed inadequate. The objectives in the metastatic series thus range from complete ablation to palliative control.
In both series of early stage NSCLC and pulmonary metatases, the guidelines on normal tissue tolerance recommended by RTOG-0236 were followed.
Between March 2004 and March 2007, 58 primary lesions in 57 patients (1 patient received treatment for synchronous primary lesions in stage 1B) and 31 patients (age range 33 to 93 years) with 56 lung metastases were treated at the CyberKnife Center of Miami. A fruther seven patients received CyberKnife boost treatments following external beam radiation therapy.
Treatment preparation and planning
A detailed description of treatment planning has been published previously Citation[13], Citation[14]. Briefly, patients selected for treatment were sent for placement of gold fiducial markers into the tumor under CT guidance using pre-loaded needles introduced transthoracically. Presently, we are using a fiducial-less technique (Xsight-Lung, Accuray, Inc., Sunnyvale, CA) for selective cases where the tumors can be well visualized by the X-ray tracking images.
An interval of 5–7 days between fiducial marker placement and the treatment planning CT scan allowed fiducial markers to stabilize and edema to subside, and assured that the fiducials had not migrated. Planning thin-slice CT scans (1.5-mm contiguous axial slices) with contrast (125 cc Omnipaque 350) were obtained while patients held their breath in expiration. Three-dimensional treatment plans were subsequently developed and evaluated by each member of the team. The prescribed dose and fractionation scheme were dependent upon tumor size, location, proximity to vital structures, and etiology, co-morbid disease, and physician preference.
To cover microscopic infiltration, macroscopic disease and tumor deformity, and to account for targeting uncertainty, margins of 8–10 mm and 3–5 mm beyond the gross tumor volume (GTV) are routinely used to define the planning treatment target volumes (PTVs) for primary tumors and metastatic tumors, respectively. The dose was prescribed to the 60–85% isodose line enclosing 100% of the GTVs and at least 95% of the PTVs. The prescribed dose delivered a heterogenous higher dose to the center of the tumor. The inverse planning module was often used to maximize dose conformality. Sufficient numbers of non-coplanar beams from a wide-spread solid angle are configured to ensure an acceptably high dose fall-off in all directions.
A skeletal tracing technique is used for the initial patient setup to reproduce global alignment. Tumor motion due to respiration is followed in real time using Synchrony©, a respiratory tracking system (Accuray, Inc.). The combination of advanced image guidance, robotic radiation delivery, and tumor motion tracking allows flexibility in meeting the stringent requirements for accurate treatment delivery.
Post-treatment follow-up
The patients were assessed at one month following treatment with a contrast-enhanced CT scan of the chest and then every three months with PET/CT scans for two years. The date and cause of death were recorded. Patients were recorded as dying from cancer if they had evidence of active cancer before expiration. Our primary endpoints were tumor control, defined as shrinkage or growth of the cancer or increasing or decreasing SUV as determined by follow-up PET/CT scanning. The response was defined as follows:
Complete response: disappearance of tumor, no longer FDG avid or very low SUV;
Partial response: a 30% decrease in tumor size and SUV;
Stable disease: less than 30% shrinkage, no increase in size, stable SUV;
Progression of disease: increasing size of tumor or metastasis, increasing SUV and recurrence/persistent disease.
In cases with ground-glass appearance on CT scans corresponding to the PTV and where the PTV remains FDG avid or has an increasing SUV it is often difficult to differentiate between recurrent/persistent cancer and radiation pneumonitis or fibrosis; an FNA is recommended as soon as the question of possible persistent or recurrent disease arises. Watchful waiting may leave one no better off and could allow for metastasis and a poorer prognosis.
Results
Primary tumors
Primary NSCLC cases were divided into two categories: Stage 1A and Stage 1B. A total of 58 primary lesions in 57 patients–29 lesions in Stage IA and 29 in Stage IB–were treated. One patient had synchronous primary lesions in the Stage 1B group and each received individual treatment. The average treatment volumes were 8 cc for the Stage IA and 34 cc for the Stage IB group. Since the treatment evolved over the years, the doses varied, depending mainly on the physician's preference. Four patients received prescribed doses of 64 to 67.5 Gy in 4–5 fractions, 20 lesions received 60 Gy in 3 fractions, 12 received 54 to 55.5 Gy in 3 fractions, and the remaining 20 lesions received less than 54 Gy total dose (7 in IA and 13 in IB).
In the Stage IA group, 29 patients with 29 lesions were treated. Two lesions received 65 to 67.5 Gy in 5 fractions, 14 lesions received 60 Gy in 3 fractions, 6 lesions received 45 to 55.5 Gy in 3 fractions, and the remainder received a lower dose. All 29 patients showed initial response at 3 months with the exception of a woman with a 5 cc tumor, which was treated with 55.5 Gy in 3 fractions. This patient had an increase in the size of her lesion with increasing FDG uptake at three months. FNA established that she suffered from persistent disease and she went on to undergo successful lobectomy in spite of her poor pulmonary function.
Of the Stage IA group, four patients died: two of co-morbidities and two of progressive disease. A total of 6 patients had disease relapse, with two local recurrences, two failures in the hilar and mediastinal lymph nodes, one case of persistent disease and one of distant metastases. Of the two local recurrences, both recurred within the PTV at 9 and 12 months, and the patients died of progressive disease at 20 and 22 months. Two patients with nodal recurrences underwent chemotherapy and are still alive at 13 and 14 months. The patient with distant metastasis died 10 months following treatment. Of the four local failures, two received 60 Gy in 3 fractions, one received 55.5 Gy in 3 fractions, and one received 36 Gy in 3 fractions. As of the date of this article, 16 patients have had a complete response.
In the Stage IB group, 28 patients with 29 lesions were treated. Three lesions received 64 to 67.5 Gy in 5 fractions, 8 lesions received 60 Gy in 3 fractions, 16 lesions received 45 to 55.5 Gy in 3 fractions, and 2 lesions received 36 Gy in 3 fractions. In this series no one was treated with a single fraction. Of this group, 17 patients are still alive with disease control with a mean follow-up of 18 months. Five patients have died: 2 from co-morbidities, 2 from progressive disease and one from distant metastases. Six patients are still alive with disease: 4 with local recurrence and 2 with distant metastases. From this cohort, 6 patients have failed locally at a dose of 36 Gy, 45 Gy, 48 Gy, 55.5 Gy and 60 Gy in 3 fractions. Of those followed for more than 3 months, 13 had a complete response, while 10 show a partial response.
Lung metastases
Thirty-one patients (13 females and 18 males) with 56 lung metastases have been treated with CyberKnife. In this series, 43 lesions were treated for single solitary metastases and 13 patients were treated for multiple metastases with a range of 2 to 7 tumors. Thirty of 56 tumors were less than 14.16 cc in volume, ranging from 1.2 to 13.2 cc. Since most of the treatment was given for palliation, the dose and fractionation were relatively lower. Two of the 56 tumors received 60 Gy in 3 fractions, 6 tumors received from 45 to 60 Gy in 2 to 3 fractions, 19 tumors received 30 to 45 Gy in 2 to 3 fractions, and the remainder received less. Eighteen tumors were treated with a single fraction, and the rest with multiple fractions. Of those treated with a single fraction with a dose range from 10 to 27 Gy, all demonstrated persistent or recurrent disease. We now prefer to use multiple fractions of IGR-SRS.
Boost
In our series, 7 patients (3 females and 4 males) were retreated with the CyberKnife for PET-proven recurrence post conventional fractionated external radiotherapy. Two patients received two treatments, and two patients have been lost to follow-up. Two had complete responses, 3 had a partial response, and one demonstrated stable disease at 2 years post salvage. Two patients have died: one from progressive disease and one from co-morbidity. Two patients remain without radiographic evidence of persistent or recurrent disease. One patient received 25 Gy/5 fractions to a PTV measuring 33.8 cc and failed locally with persistent disease. One patient who had a history of prior radiotherapy and received IGR-SRS 10 Gy in 3 fractions experienced a local failure. He was salvaged with another course of IGR-SRS 12 Gy in 1 fraction.
Recurrent/persistent disease
Thirteen patients (6 females and 7 males) with 13 of 139 treated tumors were retreated for persistent or recurrent disease. Of these 13 patients, 6 had persistent disease following IMRT, and the other 7 patients had persistent disease following CyberKnife radiosurgery . The retreatment volumes were as follows: < 20 cc for 7 lesions; 21–100 cc for 4 lesions; and > 100 cc for 2 lesions.
Figure 1. Treatment plan, June 2005, for NSCLC. SUV 7.6, 3.5 cm in diameter. 3F × 18.5 Gy. 88 non-zero beams.
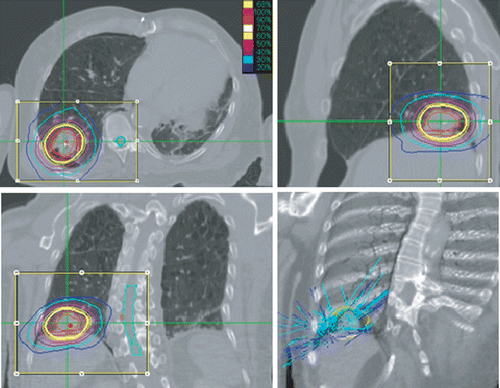
Figure 3. Same patient 11 months later. Note the hypermetabolic area adjacent to the area treated. SUV 5.6. Recurrent tumor is visible at the edge of the PTV.
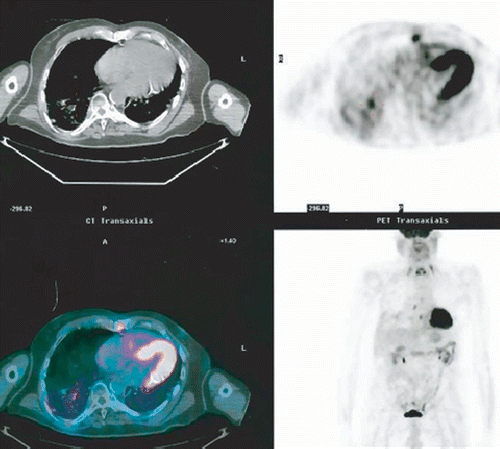
Three patients with initial Stage 1A tumor received 60 Gy in 3 fractions to tumors less than 1.5 cm. One patient with initial Stage 1A tumor treated by 36 Gy in 3 fractions showed recurrence in 3 months and was retreated by 16 Gy in 1 fraction. There was further recurrence at 8 months and the patient now awaits additional therapy. Five patients with initial Stage 1B tumor who had received 55.5 to 60 Gy in 3 fractions demonstrated persistent or recurrent disease. Four patients were treated for metastases. Seven occurred in patients with lesions receiving at least 48 Gy.
Complications
Toxicity occurred in the lung and esophagus, but none of these occurrences were grade 5. In this series, 3 of 4 cases of radiation pneumonitis occurred in the lower lobes and one in an upper lobe, similar to the findings of Seppenwoolde et al. Citation[15], who found that radiation pneumonitis was more frequent in the posterior lower lobes.Three patients had grade I-II radiation pneumonitis and one patient who developed grade III radiation pneumonitis required hospitalization following retreatment of a recurrent tumor. All four patients had focal pneumonitis corresponding to the area of PTV with a time interval of 3–6 months, and all of them resolved with supportive treatment.
Esophagitis developed in 3 patients and was treated with anit-inflammatory agents. The most common side effect was mild fatigue and this required no intervention even in this elderly frail population. Other major complications related to the placement of fiducial markers. Five patients developed pneumothorax, requiring a chest tube and/or hospitalization, and one experienced a cardiac arrest during fiducial placement. The latter patient was successfully resuscitated and she made a full recovery to receive CyberKnife treatment. In two patients, fiducials moved, requiring replacement of the markers. This happened during the period when three or more fiducials were being used for set-up. Since switching to a single fiducial marker in the tumor, the number of pneumothorax cases requiring a chest tube placement has diminished.
Discussion
With the advent of new technologies in radiation therapy, re-evaluation of radiation effects must be considered. Since concepts of radiation dose tolerance were largely developed in the era of wide-field, conventional dose schemes, tolerance must be revisited in the context of cellular responses, volume effects, and target tissue types, dose-rate and dose-delivery time for SRS.
The published studies of SBRT for lung tumors have shown promising results. IGR-SRS is akin to SBRT, but can deliver radiation from more angles using image guidance, providing better dose conformity and a steeper dose gradient. The Synchrony tracking system literally moves the radiation to follow the moving target. This represents a major advance in the treatment of moving targets such as lung tumors. Traditionally, if the motion of the treatment target cannot be determined with certainty, a relatively large margin has to apply. IGR-SRS with real-time tracking allows minimization of the dose margin beyond the clinical tumor volume (CTV), and is therefore advantageous in protecting normal tissues.
Although the patient population of this retrospective study presents a wide variety of clinical conditions, when divided into subgroups the clinical outcomes are similar to the published data for each specific subgroup.
As a new treatment modality for lung tumors, patient selection is crucial. For early stage NSCLC, 60 Gy/3 fractions or higher will be necessary for curative intent. This can only be done when the tumor size and location could warrant the dose to critical structures not exceeding tolerance Citation[18]. For patients with sub-optimal tumor conditions, a lower dose will be given with the understanding that the curative intent will be removed, and a partial response will be expected. This group of patients was treated only because there was no other treatment option left for them.
For metastatic lung tumors, the treatment objectives will dictate the treatment technique used. Complete local control is possible with a maximum dose scheme projected for a specific tumor type. A lower dose scheme is commonly used for palliative intent such that the associated complication will not further compromise the patient's already weakened physical condition.
It must be pointed out that radiosurgery for lung tumors is still in its infancy, and the optimal treatment parameters are yet to be determined for each indication. A better understanding of the radiobiology of SRS is urgently needed, such that the methodology of deriving the optimal dose schemes can rest on a better foundation. As of now, opinions still vary regarding many aspects of technical and clinical considerations.
The persistence or recurrence of disease, whether this is due to hypoxic or other extraordinarily radioresistant cells or to cells lying outside the PTV, requires reassessment. The optimal treatment margin beyond the GTV is still under investigation. The primary factors include microscopic extension and targeting uncertainties Citation[5], Citation[6], Citation[17], Citation[19]. Giraud et al. Citation[17] found a 95% chance of malignant cells at 6–8 mm outside the standard defined GTV of NSCLC. This suggests that the addition of a 5-mm margin to the GTV was not large enough. In our experience, we had recurrence at the periphery of the PTV in some patients using a 5-mm extension. This raises the following question of whether dose escalation would be needed if the PTV were to be extended. We are currently applying margins of 8–10 mm beyond the GTV for primary NSCLC, and 3–5 mm beyond the GTV for metastatic lesions. The acceptable dose coverage over the PTV is traditionally 95% or better; however, the classic intracranial SRS aims at 100% coverage. There is good reason to extend the same strategy to lung SRS to cover 100% of the PTV in order to further rule out the possibility of peripheral recurrence.
The optimal dose schemes (total dose and fractionation) have been widely debated. The rationale used for single-fraction intracranial SRS may not be simply imitated in the case of lung tumors. The maximum tolerated dose by IGR-SRS for Stage IA NSCLC has not been reported. In the absence of a well-established single ablative dose, existing studies seem to favor a multiple fractionation scheme, with 20 Gy × 3 fractions being most commonly used for NSCLC Citation[20–24]. The consensus is that fractionation not only reduces the normal tissue toxicity but is also advantageous in allowing reoxygenation of hypoxic cells, although the oxygen effect might be less profound with large fractional doses. It should be noted that, depending on the isodose level chosen for the prescribed peripheral dose, the central dose could be much higher. We have not observed the statistical significance with the variation of prescribed isodose levels.
At least in theory, treatment delivery time should be minimized to avoid the loss of biological efficiency associated with prolonged delivery times Citation[25–27]. An increase in dose conformity may result in a prolonged treatment time with an increased number of beams. The dose delivery is not continuous, and the beam-off time throughout the treatment will increase with more beams. The radiobiological data suggest that such prolongation of delivery might allow for significant radiation repair during the treatment course, with consequent lower radiation toxicity in normal tissue but lower radiation effectiveness in tumor tissue. Different SBRT or IGR-SRS systems could lead to significantly different treatment delivery times, depending on the technique used. Although there has not been a systematic study to determine the differences in clinical outcomes arising from variation in treatment delivery time, it is highly desirable to keep fractional treatment time within 1 hour.
In reality, the treatment margin, dose fractionation and treatment delivery time are inter-related in affecting the clinical outcome. An optimal dosing strategy can only be derived by a global consideration of all three factors. Naturally, this effort could only be brought to fruition with an accurate dose-computation algorithm, which also demands improvement.
Pulmonary toxicity is of great importance. In SBRT and IGR-SRS there is a component of bronchial injury in addition to the more typical pneumonitis seen in conventional radiation therapy. Damage to both parallel and serial functioning tissue must be taken into consideration of lung injury after SBRT and IGR-SRS Citation[16]. There is an apparent need to better understand the dose-volume relationship with pulmonary toxicity when large fractional doses are delivered. Although the traditional concept of V20 (the volume of functioning lung receiving ≥ 20 Gy), corrected for NTD (normalized total dose), can be used as a general guideline Citation[19], a more effective lung toxicity index is needed for SBRT or SRS. Recently, keeping the mean NTD of the normal lung below 20 Gy has been proposed as a new measure to minimize radiation pneumonitis Citation[19]. The Cyberknife is capable of creating a very steep dose gradient using multiple non-coplanar beams. This results in better control of normal lung exposure. The V15 of normal lung for our first 30 patients ranged from 1.1 to 11.2%.
Thus far, the existing literature on lung SBRT and IGR-SRS is fragmented in the sense that the techniques employed were not uniform. A set of guidelines dependent on the hardware used would greatly benefit our continuing search for the optimal treatment parameters of SBRT and IGR-SRS for lung tumors.
Conclusions
The new technology offered by CyberKnife for the treatment of lung tumors yields similar results to those reported in other SBRT studies. Our 3-year data demonstrates that for early stage lung cancer, local control and overall survival rates for IGR-SRS are comparable to those for surgical resection in some cases. IGR-SRS could evolve to be the standard treatment for inoperable early stage NSCLC. In patients with limited pulmonary metastases, IGR-STS is well tolerated, with markedly better local control compared with conventional radiation therapy. Additional trials and longer follow-up are needed to evaluate results and develop better treatment guidelines.
Disclosure
Dr. Schwade is the owner of CyberKnife Miami. None of the other authors have any financial interest.
References
- McGarry RC, Song G, des Rosiers P, Timmerman R. Observation-only management of early stage, medically inoperable lung cancer: Poor outcome. Chest 2002; 121: 1155–1158
- Qiao X, Tullgren O, Lax I, Sirzen F, Lewensohn R. The role of radiotherapy in treatment of stage I non-small cell lung cancer. Lung Cancer 2003; 41: 1–11
- Sibley GS, Jamieson TA, Marks LB, Anscher MS, Prosnitz LR. Radiotherapy alone for medically inoperable stage I non-small-cell lung cancer: The Duke experience. Int J Radiat Oncol Biol Phys 1998; 40: 149–154
- Narayan S, Henning GT, Ten Haken RK, Sullivan MA, Martel MK, Hayman JA. Results following treatment to doses of 92.4 or 102.9 Gy on a phase I dose escalation study for non-small cell lung cancer. Lung Cancer 2004; 44: 79–88
- Blomgren H, Lax I, Naslund I, Svanstrom R. Stereotactic high dose fraction radiation therapy of extracranial tumors using an accelerator. Clinical experience of the first thirty-one patients. Acta Oncol 1995; 34: 861–870
- Timmerman R, Papiez L, McGarry R, Likes L, DesRosiers C, Frost S, Williams M. Extracranial stereotactic radioablation: Results of a phase I study in medically inoperable stage I non-small cell lung cancer. Chest 2003; 124: 1946–1955
- Wulf J, Haedinger U, Oppitz U, Thiele W, Mueller G, Flentje M. Stereotactic radiotherapy for primary lung cancer and pulmonary metastases: A noninvasive treatment approach in medically inoperable patients. Int J Radiat Oncol Biol Phys 2004; 60: 186–196
- McGarry RC, Papiez L, Williams M, Whiteford T, Timmerman RD. Stereotactic body radiation therapy of early-stage non-small-cell lung carcinoma: Phase I study. Int J Radiat Oncol Biol Phys 2005; 63: 1010–1015
- Nyman J, Johansson KA, Hulten U. Stereotactic hypofractionated radiotherapy for stage I non-small cell lung cancer - mature results for medically inoperable patients. Lung Cancer 2006; 51: 97–103
- Onishi H, Araki T, Shirato H, Nagata Y, Hiraoka M, Gomi K, Yamashita T, Niibe Y, et al. Stereotactic hypofractionated high-dose irradiation for stage I nonsmall cell lung carcinoma: clinical outcomes in 245 subjects in a Japanese multiinstitutional study. Cancer 2004; 101: 1623–1631
- Radiation Therapy Oncology Group. RTOG 0236: A Phase II Trial of Stereotactic Body Radiation Therapy (SBRT) in the Treatment of Patients with Medically Inoperable Stage I/II Non-Small Cell Lung Cancer. 2004.
- Le QT, Loo BW, Ho A, Cotrutz C, Koong AC, Wakelee H, Kee ST, Constantinescu D, Whyte RI, Donington J. Results of a phase I dose-escalation study using single-fraction stereotactic radiotherapy for lung tumors. J Thoracic Oncol 2006; 1: 802–809
- Whyte RI, Crownover R, Murphy MJ, Martin DP, Rice TW, DeCamp MM, Jr, Rodebaugh R, Weinhous MS, Le QT. Stereotactic radiosurgery for lung tumors:preliminary report of phase I trail. Ann Thoracic Surg 2003; 75(4)1097–1101
- Brown WT, Perman M, Wu X, . Image guided robotic stereotactic radiosurgery for lung tumors. Robotic Radiosurgery. Vol. I, RF Mould, et al. The CyberKnife Society Press, Sunnyvale, CA 2005; 255–269
- Seppenwoolde Y, Lebesque JV, de Jaeger K, Belderbos JS, Boersma LJ, Schilstra C, Henning GT, Hayman JA, Martel MK, Ten Haken RK. Comparing different NTCP models that predict the incidence of radiation pneumonitis. Normal tissue complication probability. Int J Radiat Oncol Biol Phys 2003; 55(3)724–735
- Gopal R, Tucker SL, Komaki R, Liao Z, Forster KM, Stevens C, Kelly JF. Starkschall G. The relationship between local dose and loss of function for irradiated lung. Int J Radiat Oncol Biol Phys 2003; 56: 106–113
- Giraud P, Antoine M, Larrouy A, Milleron B, Callard P, De Rycke Y, Carette MF, Rosenwald JC, et al. Evaluation of microscopic tumor extension in non-small-cell lung cancer for three-dimensional conformal radiotherapy planning. Int J Radiat Oncol Biol Phys 2000; 48: 1015–1024
- Krol AD, Aussems P, Noordijk EM, Hermans J, Leer JW. Local irradiation alone for peripheral stage I lung cancer: Could we omit the elective regional nodal irradiation?. Int J Radiat Oncol Biol Phys 1996; 34(2)297–302
- Fowler JF, Tome WA, Fenwick JD, Mehta MP. A challenge to traditional radiation oncology. Int J Radiat Oncol Biol Phys 2004; 60(4)1241–1256
- Timmerman R, McGarry R, Yiannoutsos C, Papiez L, Tudor K, DeLuca J, Ewing M, Abdulrahman R, DesRosiers C, Williams M, et al. Excessive toxicity when treating central tumors in a phase II study of stereotactic body radiation therapy for medically inoperable early-stage lung cancer. J Clin Oncol 2006; 24: 4833–4839
- Slotman BJ, Antonisse IE, Njo KH. Limited field irradiation in early stage (T1-2 N0) non small cell lung cancer. Radiotherap Oncol 1996; 41: 41–44
- Mehta MP, Wang W, Johnson D, Wagner H, Schiller J, Veeder M, Belani C. Induction chemotherapy followed by standard radiotherapy vs. hyperfractionated accelerated radiotherapy for patients with unresectable stage IIIA and B non small cell lung cancer: Phase III study of Eastern Cooperative Oncology Group (ECOG 2597). Int J Radiat Oncol Biol Phys 2003; 57(2 Suppl)S141
- Cheung PCF, Yeung LTF, Basrur V, Ung YC, Balogh J, Danjoux CE. Accelerated hypofractionation for early stage non-small cell lung cancer. Int J Radiat Oncol Biol Phys 2002; 54(4)1014–1023
- Maguire PD, Marks LB, Sibley GS, Herndon JE, II, Clough RW, Light KL, Hernando ML, Antoine PA, Anscher MS. Gy and beyond: Hyperfractionated accelerated radiotherapy for non small lung cancer. J Clin Oncol 2001; 19(3)705–711
- Yan D. Adapt radiotherapy to temporal biology targets assessed using biological images. Proceedings of the 14th International Conference on the Use of Computers in Radiotherapy (ICCR). SeoulKorea 2004
- Fowler JF, Welsh JS, Howard SP. Loss of biological effect in prolonged fraction delivery. Int J Radiat Oncol Biol Phys 2004; 59: 242–249
- Fowler JF. Rapid repopulation in radiotherapy: A debate on mechanism. The phantom of tumor treatment - continually rapid proliferation unmasked. Radiother Oncol 1991; 22(3)156–158