Abstract
Objective: We present a phantom study evaluating the use of a three-dimensional navigation system in the treatment of mandibular angle fractures with traction screw osteosynthesis. The insertion of the so-called “Herbert bone screw” was tested on phantom models of the mandible.
Materials and Methods: A total of ten synthetic models of the lower jaw were prepared with navigational markers. After inducing artificial fractures in the mandibular angle region, axial CT scans of the models with 1.5-mm slice thickness were performed. The CT data was then transferred to the workstation of the navigation system (STN-Zeiss Navigation System, Aalen, Germany). The access to the fracture and the drilling depth were planned preoperatively on the computer monitor using the software. After calibration of the drill wire, the cannulated traction screws were inserted under three-dimensionally directed computer navigation.
Results: The inferior alveolar nerve of the model was spared in all ten cases. Cortical perforation occurred twice in the ascending lower jaw branch, but secure anchorage of the screw was still possible in every case.
Conclusion: The navigation system is a helpful visualization tool that can prevent damage to the inferior alveolar nerve and enable secure anchoring of the traction screw centrally in the cortical bone without causing perforation. This computer guided insertion method enables stable fixation of the fracture via minimally invasive surgery. Positioning of a traction screw with additional tools belonging to the screw system (drill wire) is demonstrated.
Introduction
Treatment of mandibular angle fractures is challenging and results in the highest complication rate for fractures of the mandible. Optimal treatment includes intraoperative maxillomandibular fixation (MMF), along with rigid internal fixation via an enoral access Citation[1]. In recent years, monocortical noncompression miniplates placed along the superior oblique ridge according to the biomechanical findings of Champy et al. Citation[2], Citation[3] have also gained popularity Citation[4], Citation[5].
Until recently, preoperative planning in maxillofacial surgery was primarily carried out on polyurethane models milled according to preoperatively acquired CT data. Resection lines and operative access could be visualized on the model by putting special emphasis on minimal invasiveness Citation[6–9]. Thus, CT scans have been used as a diagnostic tool for preoperative planning but not as part of the surgical phase itself.
However, intraoperative navigation technology originally developed for neurosurgical applications has gained increasing acceptance even in maxillofacial surgery over the last few years Citation[10]. It enables target structures and structures at risk to be located intraoperatively with a high degree of accuracy Citation[11], Citation[12]. Furthermore, image-data-based navigation plays an ever-increasing role in minimally invasive surgery Citation[13], Citation[14]. Many studies have demonstrated high precision in placing instruments and screws with modern navigation systems Citation[15], Citation[16].
The traction osteosynthesis used in our study was first introduced by Herbert Citation[17]. It seems well suited for image guided insertion as the drill wire that comes with the set is readily calibrated and the cannulated Herbert bone screw can be inserted along this wire, thus avoiding the problems associated with open access to the fracture. The system was first described by Herbert and Fisher Citation[17] to circumvent problems with lag screws, such as non-achieved compression, limited mobility of the adjacent joint, and difficulty in pre-determining traction screw length Citation[18], Citation[19]. The Herbert bone screw has proved to be a successful means of rigid fixation in various fields of traumatology and orthopaedic surgery Citation[20–22], offering optimal adaptation and compression of bone fragments due to the so far unique design (). These qualities, together with the possibility of a computer assisted insertion along a drill wire, provide a new method of treating lower jaw fractures using CT-scan feedback and calibrated tools belonging to the Herbert screw set.
In this study, non-dislocated jaw angle fractures were treated with the Herbert bone screw using a 3D navigation technique and minimally invasive surgery in a model operation.
Material and methods
Preoperative assessment
For this study, ten target drillings were performed in ten polyurethane models of the edentulous jaw (Type 1337, Sawbones Europe AB, Malmö, Sweden). These models are manufactured from porous polyurethane foam (solid foam) according to ASTM F-1839, which is a standard description for solid polyurethane foams used for test models in orthopaedic surgery Citation[23]. The inferior alveolar nerve was represented by a radiologically detectable copper wire ( and ). Artificial fractures were created in each model in the mandibular angle region by cutting through the foam with a saw. Four titanium bone screws were then inserted laterally on each phantom model as target points for precision analysis.
Figure 2. Phantom model of the lower jaw with a radiologically detectable copper wire representing the inferior alveolar nerve and an artificial mandibular angle fracture. [Color version available online.]
![Figure 2. Phantom model of the lower jaw with a radiologically detectable copper wire representing the inferior alveolar nerve and an artificial mandibular angle fracture. [Color version available online.]](/cms/asset/02f88ab8-0024-4990-880f-37523d3d99a2/icsu_a_288426_f0002_b.gif)
Figure 3. X-ray of the phantom model. Four titanium screws in the alveolar bone act as reference markers.
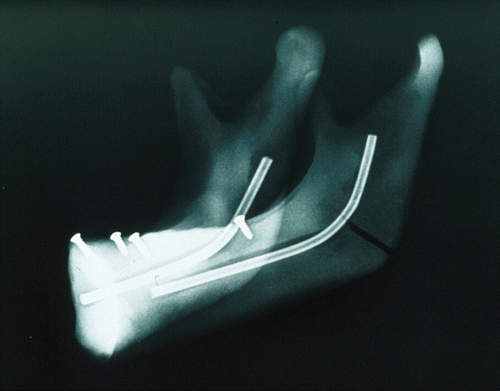
The models were scanned using a high-resolution ultrafast CT scanner (Imatron Electron Beam Tomography Scanner, Siemens, Forchheim, Germany). Reconstruction parameters of the volumetric CT data sets were optimized for use with the navigation system. The slice thickness was 1.5 mm and the increment 1 mm, resulting in approximately 40 slices with overlapping image formation. The images were transferred to a commercially available navigation system (STN-Zeiss Navigation System, Aalen, Germany) as standard DICOM files. The image-data processing software of the navigation system allows multiplanar reconstructions using an integrated computer workstation. A reference array was attached to the plastic model, so as to be directly visible to three infrared cameras linked to the computer workstation. Then the phantom was registered by aiming at the implanted bone screws with the tip of a calibrated pointer. Using the computer software, 3D reconstruction of the mandible on the workstation monitor was performed. Access to the fracture line, drilling depth and drilling direction were planned and registered on the computer monitor ().
Surgical navigation
A star-shaped passive infrared reference frame was set on the drilling handle so that it was visible to the infrared cameras. The drill wire was then calibrated intraoperatively by inserting it into the sterile rectangular calibrating station (). The instrument could be calibration in diameter and length, enabling us to locate the tip of the drill wire in all spatial dimensions with accuracy. The path of the drill wire was controlled three-dimensionally on the computer monitor. The preoperatively planned navigation path could be depicted intraoperatively from different points of view ( and ). The drilling direction was exactly determined by fitting the inner crosshair exactly into the larger outer crosshair () and could be followed via the image guidance on the workstation even without a direct view of the operation site.
Figure 5. The drill wire is calibrated in the calibration station. A trackable reference frame is fixed on the drilling handle.
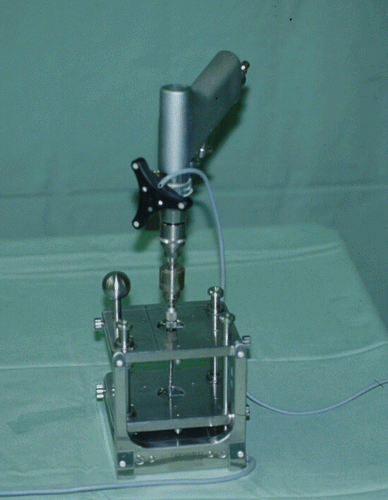
Figure 6. Insertion of the drill wire with a fixed mandibular model and a trackable reference frame.
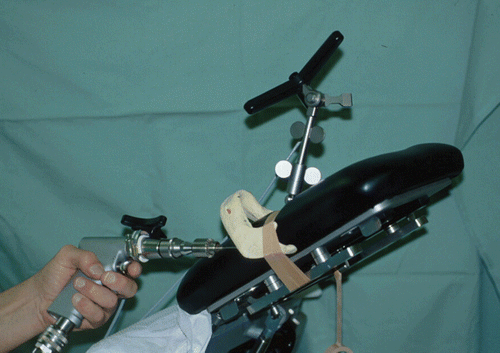
Figure 7. Corresponding view of the workstation monitor showing the preoperatively planned drilling path (yellow line) and the actual path of the drill wire (green line); the coronal layer with parallel follow-up along the preoperatively planned drilling pathway to the target (upper left); the sagittal layer (upper right); the axial layer (lower left); and the 3D depiction (lower right). [Color version available online.]
![Figure 7. Corresponding view of the workstation monitor showing the preoperatively planned drilling path (yellow line) and the actual path of the drill wire (green line); the coronal layer with parallel follow-up along the preoperatively planned drilling pathway to the target (upper left); the sagittal layer (upper right); the axial layer (lower left); and the 3D depiction (lower right). [Color version available online.]](/cms/asset/614a64d4-cada-4281-86f6-0b99c42e0f3b/icsu_a_288426_f0007_b.gif)
Figure 8. Depiction aid with superimposition of the inner and outer crosshairs on the workstation. [Color version available online.]
![Figure 8. Depiction aid with superimposition of the inner and outer crosshairs on the workstation. [Color version available online.]](/cms/asset/9cca94c5-67cc-4044-8b5a-180182f9de31/icsu_a_288426_f0008_b.gif)
Relative transposition of the drill tip was depicted through precise guidance of the drilling tool with a time delay of 50 ms. Following preparatory drilling and screw thread cutting, the canulated Herbert bone screw (Martin, Tuttlingen, Germany) was finally inserted along the drill wire.
Postoperative assessment
Damage to the inferior alveolar nerve or perforation of the buccal or lingual corticalis was checked macroscopically as well as radiologically on all ten jaw models ( and ). The preoperatively planned insertion paths of the screws were measured on the workstation using the computer software and compared to the actual lengths of the drill holes in the phantom models.
Results
All ten bone screws could be inserted successfully under image guidance, thus stabilizing the artifical fractures. The mean width of the mandible was 7.0 mm at the point of insertion of the lag screw and 6.07 mm at the tip of the screw. The mean length of the virtual insertion path amounted to 26.8 mm on the workstation and 26.63 mm in reality on the plastic models. The average length of the screws used in this study was 25.8 mm. The mean virtual insertion path on the computer monitor was 0.17 mm longer than the mean insertion path length as measured postoperatively in the phantom models ().
Table I. Results of ten target drillings in phantom models of the lower jaw. The mean planned insertion depth on the computer monitor was 0.17 mm longer than the mean actual depth measured in the phantom models.
Damage to the mandibular nerve could be avoided in all ten cases. Two cases of perforation of the cortical bone of the ascending ramus occurred. In both cases, the tip of the screw had trespassed into the outer cortical layer by approximately 0.5 mm. Considering a screw diameter of 4 mm, the remaining part of the distal thread anchored within the bone provided enough stability to guarantee a stable fixation of both fragments.
Discussion
In recent years, CT-based navigation has not only been used in neurosurgery Citation[24] but also increasingly in the area of oral and maxillofacial surgery Citation[8], Citation[9], Citation[25]. This modality enables the surgeon to transfer the preoperative plan directly into the operating theater and work with the acquired data set. Significant progress has been made with this technique in the last decade, particularly in the treatment of midface fractures Citation[26], Citation[27].
The Herbert bone screw has mainly been used in treatment of scaphoid fractures, and has shown numerous advantages in achieving stable fracture fragment fixation by minimally invasive surgery Citation[17]. It therefore seemed an obvious idea to use this screw in the treatment of lower jaw fractures too, as the Herbert bone screw set contains additional tools which can be used successfully in conjunction with 3D navigation systems. The hand piece can easily be equipped with a trackable reference frame and may be used to guide the drill wire under image guidance on the computer monitor. The high precision of avigation systems Citation[26], Citation[28] enables intra-osseous navigation without visual control of the navigated area so that antomically important structures such as nerves and vessels are not damaged along their intra-osseous paths. In our study, the access to the artificial fracture and the position of the screw were planned preoperatively on the workstation monitor. The presurgical plan could be executed with high precision in the phantom operation, avoiding damage to the alveolar nerve and showing stable fracture fixation in all ten cases.
This surgical approach also enables the performance of minimally invasive flapless procedures, avoiding the extraoral approach. Moreover, computerized navigation allows real-time reporting, which offers the possibility of modifying the surgical procedure intraoperatively without sacrificing the original principles of the treatment plan. However, it must be mentioned that inaccuracy increases with the length of the implant, as the drill wire has only a limited diameter and is highly flexible.
The osteosynthesis system used in this study offers numerous advantages, such as low cost, easy insertability, easily found operative access, and short postoperative immobilization Citation[29], Citation[30], which seem to make this bone screw suitable for use in oral and maxillofacial surgery. In this field, traction screw osteosyntheses according to Krenkel Citation[31] and Eckelt Citation[32] are already in use, especially in the treatment of lower jaw fractures. The disadvantage of these lag screws is the necessity of a second operation to remove the implant. A further disadvantage is the wedging force of the screw head applied to the bone Citation[33]. Krenkel Citation[31] reduced this problem by introducing a biconcave washer between head of the screw and the bone fragment. The advantage of the Herbert screw lies in the fact that a second operation for screw removal is not necessary and that there is no wedging force acting on the cortical bone as the screw head can be completely immersed in the bone. An as yet unsolved problem concerns the treatment of dislocated fractures which, as of now, cannot be treated by minimally invasive surgery. Thus, the indication for minimally invasive image-guided treatment of lower jaw fractures is limited to non-dislocated fractures, which can sometimes be observed in greenstick fractures or as postoperative complications following third molar surgery. A prerequisite for treatment of dislocated fractures is the possibility of acquiring CT scans intraoperatively after repositioning and immobilization of the dislocated fragments by intermaxillary fixation. Following this study of the phantom operation, the practical implementation of three-dimensionally guided insertion of the Herbert screw with minimally invasive surgery from the extra-oral approach in the case of non-dislocated fractures of the lower jaw must be established, and the surgical outcomes evaluated.
References
- Gear AJL, Apasova E, Schmitz JP, Schubert W. Treatment modalities for mandibular angle fractures. J Oral Maxillofac Surg 2005; 63: 655–663
- Champy M, Wilk A, Schnebelen JM. Treatment of mandibular fractures by means of osteosynthesis without intermaxillary immobilization according to F.X. Michelet's technic. Zahn Mund Kieferheilkd Zentralbl 1975; 63: 339–341
- Champy M, Lodde JP, Jaeger JH, Wilk A. Mandibular osteosynthesis according to the Michelet technic. I. Biomechanical bases. Rev Stomatol Chir Maxillofac 1976; 77: 569–576
- Ellis E, Walker LR. Treatment of mandibular angle fractures using one noncompression miniplate. J Oral Maxillofac Surg 1996; 54: 864–871
- Potter J, Ellis E. Treatment of mandibular angle fractures with a malleable noncompression miniplate. J Oral Maxillofac Surg 1999; 57: 288–292
- Vannier M, Marsh JL, Warren JO. Three dimensional CT reconstruction images for craniofacial surgical planning and evaluation. Radiology 1984; 150: 179–184
- Savara BS, Miller SH, Demuth RJ, Kawamoto HK. Biostereometrics and computertomographics for patients with craniofacial malformations: diagnosis and treatment planning. Plast Reconstr Surg 1985; 4: 495–501
- Kärcher H. Three-dimensional craniofacial surgery: transfer from a three-dimensional model (Endoplan) to clinical surgery: a new technique (Graz). J Cran Max Fac Surg 1992; 20: 125–131
- Rose EH, Norris MS, Rosen JM. Application of high-tech three-dimensional imaging and computer-generated models in complex facial reconstructions with vascularized bone grafts. Plast Reconstr Surg 1993; 91: 252–264
- Heiland M, Habermann CR, Schmelzle R. Indications and limitations of intraoperative navigation in maxillofacial surgery. J Oral Maxillofac Surg 2004; 62: 1059–1063
- Hassfeld S, Mühling J. Comparative examination of the accuracy of a mechanical and an optical system in CT and MRT based instrument navigation. Int J Oral Maxillofac Surg 2000; 29: 400–407
- Gaggl A, Schultes G, Kärcher H. Precision of drilling tool navigation and prevention of mandibular channel damage. J Cran Max Fac Surg 2001; 29: 271–275
- Alp M-S, Dujovny M, Misra M, Charbel FT, Ausman JI. Head registration techniques for image-guided surgery. Neurol Res 1998; 20: 31–37
- Steinmeier R, Rachinger J, Kaus M, Ganslandt O, Huk W, Fahlbusch R. Factors influencing the application accuracy of neuronavigation systems. Stereotact Funct Neurosurg 2000; 75: 188–202
- Watzinger F, Birkfellner W, Wanschitz F, Ziya F, Wagner A, Kremser J, Kainberger F, Huber K, Bergmann H, Ewers R. Placement of endosteal implants in the zygoma after maxillectomy: a cadaver study using surgical navigation. Plast Reconstr Surg 2001; 107: 659–667
- Wagner A, Schicho K, Birkfellner W, Watzinger F, Schopper C, Patruta S, Kainberger F, Figl M, Kettenbach J, Bergmann H, Ewers R. Evaluation of accuracy of computer-aided intraoperative positioning of endosseous oral implants in the edentulous mandible. Clin Oral Implants Res 2002; 13: 59–64
- Herbert TJ, Fisher WE. Management of the fractured scaphoid using a new bone screw. J Bone Joint Surg 1984; 66: 114–123
- Gasser H. Delayed union and pseudarthrosis of the carpal navicular: treatment by compression screw osteosynthesis. J Bone Joint Surg 1965; 47: 249–266
- Cooney WP, Dobyns JH, Linscheid RL. Fractures of the scaphoid: a rational approach to management. Clin Orthop 1980; 149: 90–97
- Wu KK. Modified Mitchell's bunionectomy. Orthopedics 1997; 20: 253–257
- Kanellopoulos AD, Yiannakopoulos CK, Badras LS. Triplane fracture of the proximal tibia. Am J Orthop 2003; 32: 452–454
- Sano S, Rokkaku T, Saito S, Tokunaga S, Abe Y, Moriya H. Herbert screw fixation of capitellar fractures. J Shoulder Elbow Surg 2005; 14: 307–311
- Rozema FR, Otten E, Bos RR, Boering G, van Willigen JD. Computer-aided optimization of choice and positioning of bone plates and screws used for internal fixation of mandibular fractures. Int J Oral Maxillofac Surg 1992; 21: 373–377
- Vrionis FD, Foley K, Robertson J, Shea J, Lawton MT, Spetzler RF, Brackmann DE, Kelly PJ, Day JD, Maciunas RJ. Use of cranial surface anatomic fiducials for interactive image-guided navigation in the temporal bone: a cadaveric study. Neurosurgery 1997; 40: 755–764
- Marmulla R, Niederdellmann H. Computer assisted bone segment navigation. J Cran Max Fac Surg 1998; 26: 347–359
- Marmulla R, Wagener H, Hilbert M, Niederdellmann H. Precisions of computer-assisted systems in profile reconstructive interventions on the face. Mund Kiefer Gesichts Chir 1997; 1(Suppl 1)65–67
- Marmulla R, Hilbert M, Niederdellmann H. Inherent precision of mechanical, infrared and laser-guided navigation systems for computer-assisted surgery. J Cran Max Fac Surg 1997; 25: 192–197
- Husstedt H, Heermann R, Becker H. Contribution of low dose CT-scan protocols to the total positioning error in computer-assisted surgery. Comput Aided Surg 1999; 5: 275–280
- Ritter K, Giachino AA. The treatment of pseudoarthrosis of the scaphoid by bone grafting and three methods of internal fixation. Can J Surg 2000; 43: 118–124
- Krimmer H, Kremling E, van Schonhooven J, Prommersberger K-J, Hahn P. Proximal scaphoid pseudarthrosis–reconstruction by dorsal bone screw and spongiosa transplantation. Handchir Mikrochir Plast Chir 1999; 31: 174–177
- Krenkel C. Axial “anchor” screw (lag screw with biconcave washer) or “slanted-screw” plate for osteosynthesis of fractures of the mandibular condylar process. J Cran Max Fac Surg 1992; 20: 348–353
- Eckelt U. Zugschraubenosteosynthese bei Unterkiefergelenk-fortsatzfrakturen. Dtsch Z Mund Kiefer Gesichts Chir 1991; 15: 51–57
- Kitayama S. A new method of intraoral open reduction using a screw applied through the mandibular crest of condylar fractures. J Cran Max Fac Surg 1989; 17: 16–23