Abstract
Segmented training cases for virtual simulation of bone-drilling interventions in middle ear surgery have proven to be helpful in learning about surgical anatomy of the temporal bone. The anatomy of the mastoid shows a high degree of variability, however, and the aim of this study was to evaluate whether individual virtual models could be created within an affordable timeframe, and to what extend they reflected natural individual anatomy during virtual mastoid surgery. Automatic segmentation schemes were used, and these reduced the time required to create individual models on the basis of DICOM CT scans to less than 5 minutes. Models based on CT data with a slice distance of 0.4 mm or better were found to provide excellent handling, an acceptable depiction of mastoidal organs, and a helpful impression of the individual surgical situation. Although landmarks are still more easily detected in real mastoids, virtual drilling of individual models makes the 3D estimation of specific anatomy more effective than estimations based on interpretation of CT scans alone.
Introduction
Surgical interventions in the mastoid require profound anatomic knowledge of the temporal bone, as well as a high degree of three-dimensional (3D) imagination, to avoid complications such as facial nerve palsy, hearing loss, or injuries to the dura mater. Due to its high degree of complexity and inter-individual variability, temporal bone anatomy is one of the most difficult topics to learn during the education of ENT surgeons.
Training in mastoid surgery is traditionally and most effectively done by performing cadaver dissections under professional supervision, supported by the study of anatomic illustrations. The latter, of course, can only give incomplete and two-dimensional views of the region of interest: To obtain an impression of spatial relations, the student or surgeon has to mentally combine several pictures and cross-sections into a 3D object, not knowing whether this imaginary construct matches the true situation until it is confirmed or disproved by surgical practice. In contrast, cadaver dissections show the complete anatomy and its spatial relations, but, as a result of the fixation process, cadaveric tissues do not reflect the real color and firmness of the corresponding tissues in a living patient. Additionally, each cadaver subject can only be used for dissection once, and therefore can only serve a limited number of trainees. In view of these limitations, and the increasing difficulty (for a variety of reasons) of acquiring donor human cadavers from year to year, compensating strategies are necessary.
In recent years, supported by rapidly developing data-processing technology, various virtual training systems for bone-drilling interventions in middle ear surgery have been developed Citation[1]. Attempts to simulate real interventions involve dealing with various aspects such as data acquisition Citation[2], development of virtual environments in general Citation[3],Citation[4], creation of the model itself Citation[5], and enabling interaction with it in a way that seems natural Citation[6]. Examples of approaches to this field are stereoscopic reproduction of photographically collected data Citation[7], stereolithographic reconstruction of CT data Citation[8], 3D model printing on the basis of CT scans Citation[9], and conversion of CT data into a 3D virtual voxel model Citation[10–14]. Some systems have attained a high degree of maturity, combining different aspects to create a realistic environment including visual, acoustic and haptic feedback, natural positioning of the surgeon, drill control by foot pedal, and stereoscopic view.
While cadaver dissections and mature virtual training systems are capable of delivering solid anatomic background as well as being validated training tools Citation[15–18], they still do not meet the special requirements that every mastoid surgeon has to deal with in certain patient cases, because they cannot represent all the anatomic and pathologic variations that are encountered in clinical routine. Estimating individual anatomy and individual pathology is done with the help of X-rays or CT scans, depending on the complexity of the case, again leaving the surgeon with the challenge of mentally reconstructing the information three-dimensionally.
Strategies aimed at the possibility of evaluating specific anatomy and interacting with it virtually have led to the creation of individual models Citation[19]. Unfortunately, the clinical use of patient-specific models is problematic, because complete segmentation of the data is too laborious and time-consuming. It is the purpose of this paper to evaluate the possibility of creating individual models of the mastoid on the basis of DICOM data acquired by CT, using a simple threshold-based segmentation scheme embedded in a user-friendly system for virtual bone drilling and navigation.
In detail, the following questions must be answered: (1) What level of spatial resolution of the CT images is needed?; (2) To what extent is the resulting model able to show anatomic structures of interest like the facial nerve or the sigmoid sinus?; and (3) Is the time expenditure affordable for potential use in clinical routine?
Materials and methods
For the study, the VOXEL-MAN TempoSurg simulator (Spiggle & Theis Medizintechnik GmbH, Overath, Germany) was used. It was developed by the VOXEL-MAN group at the University Medical Center Hamburg-Eppendorf to provide a setting for virtual bone drilling with high (“subvoxel”) resolution, as well as visual, acoustic and haptic feedback. It contains several completely segmented training cases ( and ), and was recently complemented by new features for processing individual data.
Figure 2. Training case “pneumatized”: Mastoidectomy is complete, with the middle (1) and posterior (2) cranial fossae, the incus (3), the facial nerve (4), the semicircular ducts (5) and the sigmoid sinus (6) clearly visible.
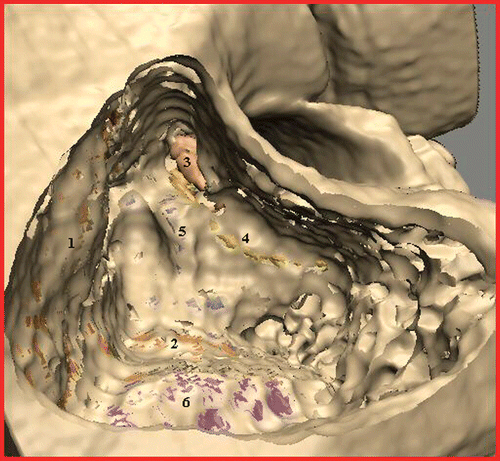
Creation of individual bone-drilling models involves the following steps. The data source is a DICOM CT which is brought into the system by a data import module. To avoid overloading the processing capacity, the raw data are released from the unnecessary parts. This is done by discarding slices beyond the mastoid and cropping the remaining parts to the region of interest using one representative slice to determine the width of the picture (). Definition of all the bony structures to be incorporated into the resulting model is interactively done by the surgeon using a threshold-based segmentation scheme (). Thus defined, parts of the DICOM CT are then converted into a 3D voxel model () similar to the VOXEL-MAN training cases but without segmented soft tissue. To enable its position to be changed in real time, the resulting model can temporarily be converted into a surface model whenever necessary (). The surgical site is presented with the help of a mirror which allows the surgeon to work in a natural surgical position. Virtual drills of different shape and size can be used, optionally under the control of real-time CT navigation in all planes, in which removed parts of the bone are automatically marked in color ().
Figure 3. Representative individual CT slice with cropping frame for limitation of the data volume. The incus can be clearly identified as a distinct object.
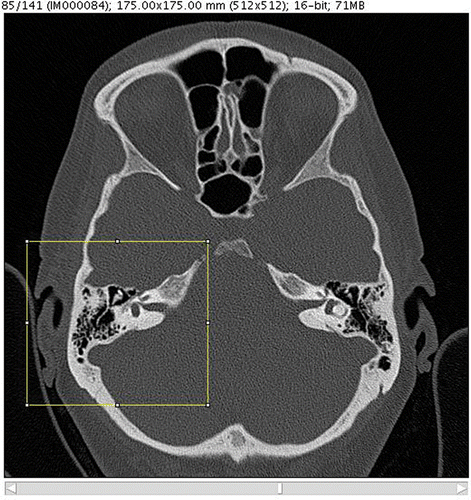
Figure 4. Threshold-based segmentation applied to the cropped image. The lateral semicircular duct is visible as a void in its bony surrounding.
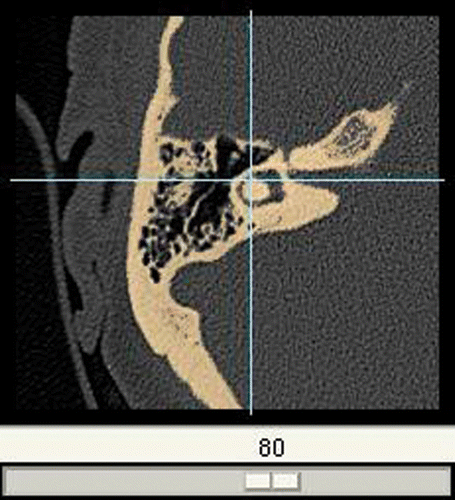
Figure 7. Additional real-time CT navigation in separate window with removed parts of the bone marked in green.
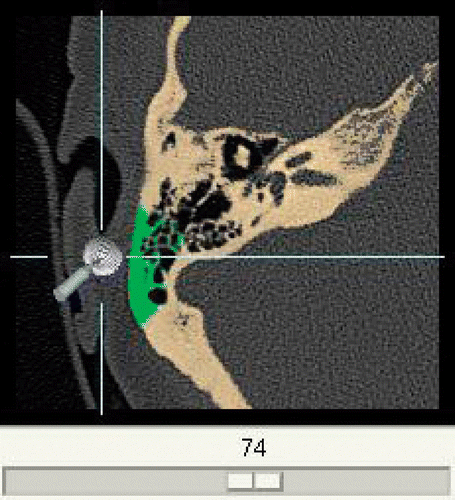
To answer the initial questions concerning the original data requirements, the visibility of organs at risk, and the time expenditure, a set of 20 DICOM CTs of the petrous bone acquired in clinical routine work was processed. Antrotomy, mastoidectomy, posterior hypotympanotomy, cochleostomy and radical mastoidectomy were simulated in each created model by an expert ENT surgeon in chronological order. The accuracy of the surgeon's identification of temporal bone structures was assessed with the help of the navigation tool.
Results
Image quality
It was found that the quality of the individual model is best when the CT images meet the following quality criteria: on the transverse cross-sections, the auditory ossicles must be visible as distinct objects (), and the lateral semicircular duct, the cochlea and the facial nerve must be visible as voids in the bone structure (). The latter, along with a precise optical contrast between bone and soft tissue in the CT scan, is prerequisite for reliable functioning of the segmentation process and thus for acceptable quality of the resulting model. To meet these requirements, the slice distance and slice thickness of the CT scan should be 0.4 mm or better.
Anatomy
Provided that the quality of the CT scan meets the above-mentioned requirements, it can be shown that all landmarks such as the sinus sigmoideus, the skull base, or the incus can be clearly identified during virtual surgery. While the ossicles themselves are segmentable, organs at risk like the facial nerve, semicircular ducts, cochlea and sinus sigmoideus have to be derived from their bony shell (), similar to the process in a real surgical intervention. Preparation must therefore be done more carefully than when using the VOXEL-MAN training cases. The individual virtual model is limited by the absence of the eardrum and the intratympanal part of the chorda tympani, since those are not coated by a threshold-based segmentable bony structure.
Time consumption
The mean time expenditure for the preparation of the data () was 5 minutes for a surgeon who had spent two hours acquainting himself with the system. The time for the simulation of the intervention is comparable to that for a real procedure, and depends on the severity of the mastoid, the type of operation, the surgeon's experience, and the amount of detail he needs to simulate.
Table I. Time consumption for the different steps.
Discussion
The main conclusion from this study of the use of individual bone-drilling models is that the simulation mimics the real preparation situation at several decisive points (understanding of individual anatomy, extension of malformations, haptic feel of the drilling process). The auditory ossicles are clearly visible, and the location of other sensible organs such as the facial nerve, semicircular ducts or sigmoid sinus can be inferred from the surrounding bone anatomy. The absence of soft tissue landmarks like the eardrum or chorda tympani, as present in completely segmented models or in the vital mastoid, is counterbalanced by the additional navigation tool. These findings bring the quality of simulation close to that of practicing on cadaveric specimens, with the decisive advantage that the simulation reflects individual patient anatomy.
In summary, we can state that the anticipation quality of individual bone-drilling interventions in mastoid surgery is substantially improved by the above-described simulation. In future, by using patient-specific models, virtual mastoid surgery could become a part of clinical routine, besides being an excellent education and training tool. Of course, radiation exposure concerns and time available will limit the virtual procedures to selected cases, such as extensive cholesteatoma or skull-base interventions.
References
- Fried MP, Uribe JI, Sadoughi B. The role of virtual reality in surgical training in otorhinolaryngology. Curr Opin Otolaryngol Head Neck Surg 2007; 15: 163–169
- Wiet GJ, Schmalbrock P, Powell K, Stredney D. Use of ultra-high-resolution data for temporal bone dissection simulation. Otolaryngol Head Neck Surg 2005; 133: 911–915
- Edmond CV, Jr, Wiet GJ, Bolger B. Virtual environments. Surgical simulation in otolaryngology. Otolaryngol Clin North Am 1998; 31: 369–381
- Jackson A, John NW, Thacker NA, Ramsden RT, Gillespie JE, Gobbetti E, Zanetti G, Stone R, Linney AD, Alusi GH, et al. Developing a virtual reality environment in petrous bone surgery: A state-of-the-art review. Otol Neurotol 2002; 23: 111–121
- Pommert A, Höhne KH, Burmester E, Gehrmann S, Leuwer R, Petersik A, Pflesser B, Tiede U. Computer-based anatomy: a prerequisite for computer-assisted radiology and surgery. Acad Radiol 2006; 13: 104–112
- Pflesser B, Petersik A, Tiede U, Höhne KH, Leuwer R. Volume cutting for virtual petrous bone surgery. Comput Aided Surg 2002; 7: 74–83
- Bernardo A, Preul MC, Zabramski JM, Spetzler RF. A three-dimensional interactive virtual dissection model to simulate transpetrous surgical avenues. Neurosurgery 2003; 52: 499–505
- Vorwerk U, Begall K. Practice surgery on the artificial temporal bone. Development of temporal bone facsimiles with stereolithography. HNO 1998; 46: 246–251
- Schneider G, Müller A. Multi-center study of the Jenaer model of the temporal bone. Laryngorhinootologie 2004; 83: 363–366
- Wiet GJ, Bryan J, Dodson E, Sessanna D, Stredney D, Schmalbrock P, Welling B. Virtual temporal bone dissection simulation. Stud Health Technol Inform 2000; 70: 378–384
- Leuwer R, Pflesser B, Urban M. The stereoscopic simulation of otosurgical approaches by means of a novel 3D-computer model. Laryngorhinootologie 2001; 80: 298–302
- Wiet GJ, Stredney D, Sessanna D, Bryan JA, Welling DB, Schmalbrock P. Virtual temporal bone dissection: An interactive surgical simulator. Otolaryngol Head Neck Surg 2002; 127: 79–83
- Agus M, Giachetti A, Gobbetti E, Zanetti G, Zorcolo A, John NW, Stone RJ. Mastoidectomy simulation with combined visual and haptic feedback. Stud Health Technol Inform 2002; 85: 17–23
- Neri E, Sellari Franceschini S, Berrettini S, Caramella D, Bartolozzi C. IERAPSI Project: Simulation of a canal wall-up mastoidectomy. Comput Aided Surg 2006; 11: 99–102
- Agus M, Giachetti A, Gobbetti E, Zanetti G, Zorcolo A, Picasso B, Sellari Franceschini S. A haptic model of a bone-cutting burr. Stud Health Technol Inform 2003; 94: 4–10
- Morris D, Sewell C, Barbagli F, Salisbury K, Blevins NH, Girod S. Visuohaptic simulation of bone surgery for training and evaluation. IEEE Comput Graph Appl 2006; 26: 48–57
- Sewell C, Morris D, Blevins NH, Agrawal S, Dutta S, Barbagli F, Salisbury K. Validating metrics for a mastoidectomy simulator. Stud Health Technol Inform 2007; 125: 421–426
- Zirkle M, Roberson DW, Leuwer R, Dubrowski A. Using a virtual reality temporal bone simulator to assess otolaryngology trainees. Laryngoscope 2007; 117: 258–263
- Tolsdorff B, Petersik A, Pflesser B, Pommert A, Tiede U, Leuwer R, Höhne KH. Preoperative simulation of bone drilling in temporal bone surgery. Int J CARS 2007; 2(Suppl 1)S160–S161