Abstract
Navigation in hand surgery is still in the process of development. Initial studies have demonstrated the feasibility of 2D and 3D navigation for the palmar approach in scaphoid fractures, but a comparison of the possibilities of 2D and 3D navigation for the dorsal approach is still lacking. The aim of the present work was to test navigation for the dorsal approach in the scaphoid using cadaver bones. After development of a special radiolucent resting splint for the dorsal approach, we performed 2D- and 3D-navigated scaphoid osteosynthesis in 12 fresh-frozen cadaver forearms using a headless compression screw (Synthes). The operation time, radiation time, number of trials for screw insertion, and screw positions were analyzed. In six 2D-navigated screw osteosyntheses, we found two false positions with an average radiation time of 5 ± 2 seconds. Using 3D navigation, we detected one false position. A false position indicates divergence from the ideal line of the axis of the scaphoid but without penetration of the cortex. The initial scan clearly increased overall radiation time in the 3D-navigated group, and for both navigation procedures operating time was longer than in our clinical experience without navigation. Nonetheless, 2D and 3D navigation for non-dislocated scaphoid fractures is feasible, and navigation might reduce the risk of choosing an incorrect screw length, thereby possibly avoiding injury to the subtending cortex. The 3D navigation is more difficult to interpret than 2D fluoroscopic navigation but shows greater precision. Overall, navigation is costly, and the moderate advantages it offers for osteosynthesis of scaphoid fractures must be considered critically in comparisons with conventional operating techniques.
Introduction
Conservative treatment of non-displaced fractures of the os scaphoideum is widely established and has long represented the treatment of choice. However, conservative treatment is also associated with a plaster cast immobilization of 12–16 weeks. Furthermore, especially with involvement of the distal part of the scaphoid, conservative treatment may result in higher rates of non-healing or delayed healing Citation[1]. To support fracture healing and shorten the period of immobilization, percutaneous screw osteosynthesis through an anterior approach has been introduced. Screw stabilization is also thought to enable early physiotherapy and improve functional outcomes; however, the operative procedure is technically demanding and requires considerable individual experience. The procedure is further associated with quite high levels of intraoperative radiation exposure and screw misplacement rates of up to 5–30% Citation[2–5]. Navigated procedures have recently been described as suitable tools for guiding percutaneous screw placement into the scaphoid. Liverneaux et al. Citation[6] found that a 2D technique based on conventional fluoroscopy diminished intraoperative radiation exposure; however, the screw misplacement rates were not decreased sufficiently, while procedure times were raised only slightly. The initial results of a feasibility study using a navigated 3D technique seemed to indicate some advantages compared to the conventional and 2D techniques Citation[4], but studies are not yet available comparing both techniques via a dorsal approach, which is especially advantageous for non-displaced fractures. Therefore, the aim of this study was to compare 2D and 3D computer-navigated procedures for the dorsal approach for percutaneous screw insertion into the scaphoid.
Materials and methods
Study design
Sixteen upper limbs of fresh-frozen anatomical subjects sectioned at the elbow were prepared. Eight pairs of adult human forearms were included (n = 16), 3 male and 5 female, with an average age of 64.9 ± 12.3 years. The limb specimens were no older than 6 months. For 2D and 3D navigation, the VectorVision Fluoro3D 2.0 navigation system (BrainLAB, Munich, Germany) was used in combination with an ARCADIS Orbic 3D C-arm (Siemens AG, Erlangen, Germany). We excluded the first four cases from our comparative study, considering them part of the learning curve. The 12 remaining limbs were then divided into two groups: the 2D fluoroscopic navigation group included six scaphoids, three right and three left, for a dorsal approach; and the 3D navigation group also included six scaphoids, three right and three left, again using a dorsal approach. For both groups, the scaphoids were percutaneously fixed under navigation control with a navigated guide pin, then a cannulated self-drilling, self-tapping, headless screw, the 3.0-mm Headless Compression Screw (Synthes, Switzerland), was inserted. In both the 2D and 3D groups, the distal limb from forearm to fingers was immobilized in the usual position for dorsal percutaneous scaphoid screw placement, i.e., in flexion and at an ulnar angle of the wrist. The necessary stable immobilization of the forearm was assured by a resting splint specially developed for dorsal interventions and made of radiolucent material (PE; Thum & Wilharm, Hamburg, Germany) (). The tracker for the 2D and 3D navigation could easily be positioned on this splint. In addition to the splint, a navigable drill guide and a corresponding guide wire were used for the intervention. A tracking marker was also attached to the accumulator drill (Colibri, Synthes, Switzerland) (). The same surgeon handled all procedures to exclude inaccuracies that might be introduced by differing levels of experience and manual skill.
Figure 1. Stable immobilization of the forearm was assured by a specially developed splint for the dorsal intervention made from radiolucent material. The tracker for the 2D and 3D navigation could easily be positioned on this splint.
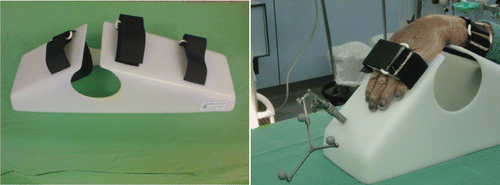
Figure 2. The instruments for the navigation. (A) Calibration device with the calibrated pointer. (B) Tracker for 2D and 3D navigation that can be attached to the splint. (C) Surgical motor, equipped with reflective marker spheres to enable its calibration and thereby its recognition by the optic localization system. (D) Drill guide, also equipped with marker spheres.
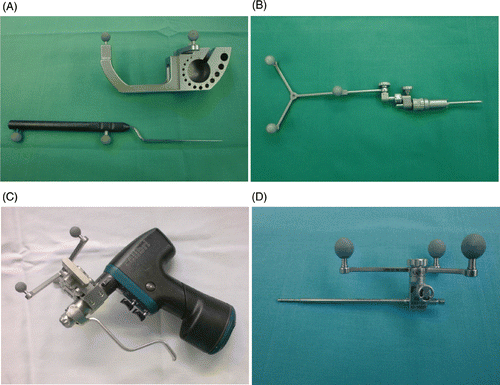
2D navigation: For the 2D navigation, we used the same method as Liverneaux et al. Citation[6] with a few changes, including using the use of a dorsal approach and navigating the drill guide for the K-wire rather than the screwdriver at the end. All surgical instruments, as well as the specially developed splint, were equipped with reflective marker spheres that were visible to a 3D optical localization system and detectable by the VectorVision computer aided surgery system. Three standard projections of the wrist (anteroposterior, lateral, and oblique) were obtained using a fluoroscope (ARCADIS Orbic 3D; Siemens AG), with the wrist immobilized in flexion.
The intensifier of the fluoroscope was equipped with a registration kit. Using computerized calculation, the registration kit enabled the correction of fluoroscopic image distortions. The images, which were digitally reformatted by specific algorithms, allowed instrument movements to be displayed in real time and simultaneously on the computer screen. Three views of the scaphoid were provided: anteroposterior, lateral, and oblique (). The degree of precision was within 1 mm, enabling simulation of distal pin insertion along the axis of the scaphoid (K-wire axis) using a precalibrated pointer (). The K-wire axis should yield the axis of the scaphoid. A K-wire was then fixed to a surgical motor that was equipped with reflective marker spheres enabling its calibration and thus its recognition by the optical localization system. Following a virtual determination of the scaphoid axis, the surgeon inserted a K-wire (length 100 mm, diameter 1.1 mm) from the proximal to the distal side with the help of a calibrated surgical motor. The drill guide was also equipped with reflective marker spheres (). The surgeon could track the wire with the help of the virtual images shown on the computer screen, and a fluoroscope was therefore not used for this purpose. Finally, the screw was measured, and a 22–28 mm cannulated screw (Headless Compression Screw; Synthes) was inserted over the pin using a normal screwdriver.
Figure 3. Pin insertion using 2D fluoroscopic navigation. (A) The computer screen displays three views of the wrist: lateral, oblique, and anteroposterior. The surgeon first determines the ideal trajectory of the screw along the scaphoid axis in all 3 planes. The exact position of the screw can then be reproduced with the navigated drill guide. The view at lower right enables alignment parallel to the planned implant position to be maintained in all available projections. Pin insertion can now begin. In this case, the direction of the drill was first imitated by using a calibrated pointer applied to the scaphoid tubercle. (B) Application of the calibrated pointer to the scaphoid in the cadaver.
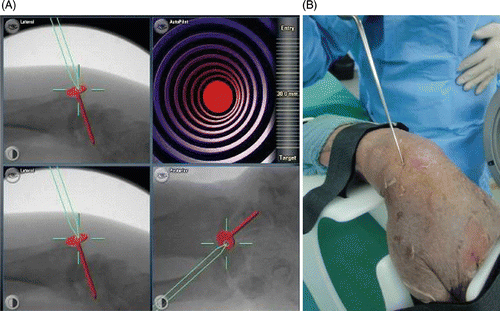
Figure 4. (A) Navigation screen for the 3D-navigated screw insertion. The presentation of multiplanar reconstructions allows exact positioning of the screw with respect to the drill. The head of the red screw corresponds to the end of the instrument to be navigated. The solid line indicates the extended virtual direction of the drill guide. (B) The navigated drill guide with the K-wire already inserted.
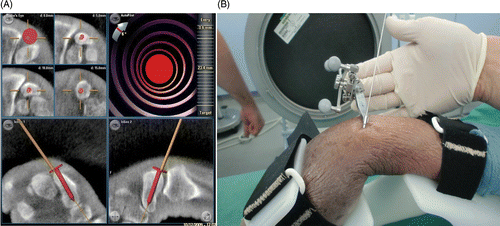
3D navigation: Following data acquisition with the 3D C-arm, we performed the operative planning with the navigation system, producing suitable lines for the drill guide and enabling planning of the screw placement at multiple levels of the CT reconstruction planes. In accordance with the conventional technology, an approximately 1-cm skin incision and a dull preparation to the proximal part of the scaphoid were performed. After calibration of the navigated drill, the drill guide was applied through the dorsal approach from the proximal side of the scaphoid under constant navigation control. During the placement of the K-wire, no other X-rays were acquired.
After introduction of the navigated K-wire, the screw was placed using a screwdriver. Following removal of the K-wire, we obtained X-rays to verify the position of the screw. The operation time and radiation time were documented intraoperatively. Furthermore, the scaphoid was explanted after the operation and the precision of the screw placement measured using contact radiography. A primary aim was to determine whether the preoperative planning for the navigation correlated with the postoperative result. To calculate the precision of screw placement in a manner similar to that of Liverneaux et al. Citation[6], we used contact radiography of the scaphoid. In addition, divergence from the ideal placement of the screw was measured in degrees in both views.
Statistical analysis
With respect to the learning curve, the first two cadavers in each group were used as trial specimens and the results of these trials were not included in the statistical assessment. The rest of the study data are presented as mean value ± standard deviation and range. Differences between the study groups were evaluated using a t-test. If the sample data were not normally distributed, the Mann-Whitney rank sum test was used instead. Frequency distributions including 95% confidence intervals were compared using the chi-square test. All tests were calculated with a desired power of 1 − ß = 0.8 and a significance level of α = 0.05. Statistical analysis was performed with SigmaStat (version 2.03) (SPSS, Inc., Chicago, IL).
Results
Based on the work of Liverneaux et al. Citation[6], we evaluated the results by measuring the number of guide wire placements required using 2D and 3D navigation, the total fluoroscopic time needed to perform each technique, the total operative time, and the accuracy of screw insertion (). During screw insertion, we counted the number of trial guide wire insertions performed before achieving a position considered to be satisfactory. The X-ray exposure time was measured in seconds of radiation for each group. In the 3D navigation group, a complete scan had to be performed at the beginning of the procedure. The radiation time for this scan amounted to 1.5 min, and this time was added to the 3D navigation time to allow comparison between the procedures. Finally, the total duration of the surgical procedure was measured. In both groups, this included the time for positioning the upper limb in the immobilization device, as well as the time for navigation-assisted pin insertion and screw placement, depending on the group. The time for calibration of the entire system, as well as the time for the 3D scan (for the 3D navigation) and the calibration of the surgical instruments, was also included in the total duration of the surgical procedure. shows these results.
Figure 5. Method for calculating precision of screw placement on the basis of anteroposterior (AP) and lateral X-ray images of the scaphoid following the calculation by Liverneaux et al. Citation[6]. The gray line represents the scaphoid axis and the white line the screw axis. (A) Angle α represents the angle between the scaphoid axis and the screw axis in an AP X-ray image; α is positive when the entry point of the screw is medial in relation to the axis, and negative when the entry point is lateral in relation to the axis. (B) Diagram of the scaphoid in lateral view. Angle β represents the angle between the scaphoid axis and the screw axis in a lateral X-ray image; β is positive when the entry point of the screw is posterior in relation to the axis, and negative when the entry point is anterior in relation to the axis.
![Figure 5. Method for calculating precision of screw placement on the basis of anteroposterior (AP) and lateral X-ray images of the scaphoid following the calculation by Liverneaux et al. Citation[6]. The gray line represents the scaphoid axis and the white line the screw axis. (A) Angle α represents the angle between the scaphoid axis and the screw axis in an AP X-ray image; α is positive when the entry point of the screw is medial in relation to the axis, and negative when the entry point is lateral in relation to the axis. (B) Diagram of the scaphoid in lateral view. Angle β represents the angle between the scaphoid axis and the screw axis in a lateral X-ray image; β is positive when the entry point of the screw is posterior in relation to the axis, and negative when the entry point is anterior in relation to the axis.](/cms/asset/27e587a7-c7c6-4223-937b-074bbc6d7611/icsu_a_621092_f0005_b.gif)
Table I. Overview of the different values for 2D and 3D navigation. We measured the operation time, the X-ray time, the number of attempts to place the K-wire properly, and the number of false positions. Malposition here means more than 15° divergence in one or both angles from the axis of the scaphoid. In no case was the cortex penetrated by the screw.
In the 2D-navigated group, the mean operation time was 27 ± 6 min – considerably shorter than the 45 ± 15 min in the 3D group (p = 0.01). If we look only at the fluoroscopy time and remove the time for the initial scan from the 3D group, the fluoroscopy time in the 2D group (15 ± 7 s) was three times higher than that in the 3D group (5 ± 2 s).
Another important aim was to determine the false positions of the K-wires. In the 3D group, at least one false position was identified, while two false positions were found in the 2D group. The cause of this false positioning was probably movement between the splint and the cadaver in the 3D group or a minimal motion between the radius and the carpal bones in the 2D group. A false position does not invariably imply clinical relevance, but could mean, for instance, that the screw has broken through the distal cortex. Specifically, we identified as false any screw position that deviated by more than 15° from the axis of the scaphoid (). For quantification of the screw positions following the procedures, the bones were removed from the wrist and contact radiography was performed at standardized levels. To facilitate comparability, based on the work of Liverneaux et al. Citation[6], we calculated the divergences of the ideal line in the same manner. In each case, the axis of the scaphoid was marked and the angle to the screw measured. The average divergence from the ideal line in the scaphoid for the 2D-navigated screws was 13 ± 5.2° for α and 7 ± 3.6° for β. In the 3D-navigated group, the corresponding values were 10 ± 4.1° for α and 4 ± 2.3° for β. There was a tendency towards greater precision in the 3D group, but this was not statistically significant (p = 0.182; p = 0.009).
Table II. Comparison of the precision of 2D and 3D screw insertion navigation techniques.
Discussion
The relevance of computer-assisted surgery (CAS) has clearly increased in recent years. In orthopedic surgery, it is used in spine and pelvic surgery in particular, and has grown considerably in popularity Citation[7–9]. Therapy for fractures of the scaphoid is an interesting indication for CAS, although it also involves some potential difficulties. The introduction of the initial wire can pose a problem even for the experienced hand surgeon, and repeated placement of the K-wires, as well as an increased number of imaging processes, may be necessary before the wire is placed in the required central position in the scaphoid. For this procedure, both a palmar approach and a dorsal approach are available, and the best access for different fracture patterns has been the subject of controversy for many years Citation[10–11]. The dorsal approach seems to be advantageous for fractures of the proximal pole, non-displaced fractures, and wave aligned non-unions. Placement of the screw in the central axis is biomechanically advantageous and easier when attempted dorsally. The palmar approach is more advantageous for fixation of fractures of the distal pole and unstable fractures, as the risk of fracture displacement is lower when dorsiflexing the wrist Citation[10–13]. Because we use the dorsal access most often, and because data are lacking regarding the combination of this approach with additional navigation, we chose the dorsal access for our experiments.
In hand surgery, the first attempts to use CAS trace back to the work of Liverneaux et al. Citation[6], who demonstrated the feasibility of 2D navigation in 2005. The aim of the present study was to ascertain whether 3D navigation was also applicable and to compare it with 2D navigation. For this purpose, we included 12 cadaver scaphoids in the evaluation, operated through a dorsal approach, which represents a divergence from the previous work in which the screws were introduced through a palmar approach Citation[6]. The recognized problem of minimal shifts between specimens and the splint was solved by firm placement on a special splint as demonstrated by others [4, 14]. For the navigation to be precise, it is important that stable, motion-free reference markers be placed near the anatomic region of interest and registered by the navigation system Citation[15], Citation[16].
The first important parameter to be addressed was the total operation time. If we compare times from the cadaver studies with the average operation times known from our clinical experience without navigation, the time with navigation is unquestionably lengthened. An evaluation in our clinic of 15 conventionally treated non-dislocated fractures of the scaphoid with percutaneous screw insertion showed that operative time never exceeded 21 min; thus, the time for a navigated operation on the scaphoid was definitively extended in the current work. The operation time was particularly extended for the 3D navigation with its initial scan, as compared to the 2D navigation. Here, however, our experience does not completely match that of other authors. We found that the time expenditure was considerable for navigation in the wrist, and, indeed, the times reported by Liverneaux et al. Citation[6] for the navigated procedure hardly differ from ours. However, they also included in the navigation the time required for set-up, image acquisition, and instrument calibration, which slowed down the procedure, even after an initial period of familiarization with the system. That noted, in the first-described clinical applications of navigation, the times given are up to 3 hours Citation[14].
Diminished radiation exposure is always raised as an advantage of navigation. However, in this study we identified a clearly extended exposure time, especially with 3D navigation. The average X-ray time given for conventional screw osteosynthesis is well under 22 s Citation[6]. In our study, the total X-ray time was much longer in the 3D group than in the 2D group: The initial scan for 3D navigation alone lasted 1.5 min; thus, only 2D navigation reduced radiation time.
As a widely used implant, we chose the Synthes Headless Compression Screw that allows sufficient compression on a fracture gap. Studies have shown a clear decrease in pseudarthrosis following screw osteosynthesis, even though pseudarthrosis was still present at relatively high rates after non-dislocated fractures Citation[1], Citation[10], Citation[17]. One problem with the insertion of these screws is the choice of screw length. The standard measuring procedures, even when used correctly, do not fully protect against selection of an incorrect screw length. A screw that is too short will not fulfill its purpose, while one that is too long can lead to articular damage. Navigation may offer some advantages in this context because screw length can be calculated quite precisely. Some purely anatomical studies impressively emphasize the need for correct positioning of the screw Citation[11], Citation[14], Citation[18].
Considering the high vulnerability of the scaphoid with the exceptionally high rate of pseudarthrosis, it is important to keep surgical therapy brief and with as little soft tissue damage as possible. Therefore, multiple wire and screw changes should be avoided. In this context, we also saw moderate advantages with the navigated procedure: In the conventional technique, if the pin position is insufficiently accurate, the surgeon repeats the procedure via a different pathway, leaving behind the trauma of the previous insertions, whereas with the use of a navigation system, multiple pin positions can be avoided.
The complication rate for the dorsal percutaneous method reaches as high as 29% Citation[10], Citation[18], Citation[19], often because of a non-ideal positioning of the screw. Discussion of the ideal screw positioning continues, with the anatomical scaphoid axis being considered too complex and essentially too variable for use in clinical practice. The anatomical axis can be defined by a curve traversing all slice barycenters. With this method, the axis would thus be roughly concave inside and at the bottom in an anteroposterior view, form an italic S in an oblique view, and be concave at the front and at the top in a lateral view. Currently, there is no osteosynthetic material that can be fixed along this winding anatomical axis. The “surgical” axis is actually more intuitive and corresponds to the straight form of the material used to perform fixation. From our viewpoint, this axis corresponds to the line passing through the middle of the tangent at the scaphoid tubercle and through the middle of the tangent of the proximal scaphoid pole. We thus decided to use this “surgical” axis in our study to compare the two techniques. Looking at the divergences of the screws from this surgical axis given by the angles calculated, we detected no significant differences between the methods, although there was a tendency towards greater precision in the 3D group.
Overall, then, we identified only minor differences with navigation. The 3D navigation seemed to allow a little more precise screw insertion, as demonstrated by the smaller divergences from the anatomical axis of the scaphoid. From our experiences with these investigations, however, we also note that the images and different views from the 3D navigation were sometimes difficult to interpret, and that proper evaluation of the images requires considerable experience. The learning curve for this procedure was steeper for the 3D navigation; 2D navigation was already well known to us from our clinical experience. From that perspective, it is critical to ask whether navigation really offers advantages for experienced hand surgeons. For dislocated fractures of the scaphoid, navigated procedures are even less of an option, because there the correct reduction of the bone is paramount. For non-dislocated fractures, 2D as well as 3D navigation might have some advantages, but in those cases screw length can be estimated presurgically based on the CT scan.
Thus, 2D and 3D navigation in the scaphoid is feasible, with advantages in planning the correct positioning of the wire and determining the correct screw length, which might reduce implant costs and possibly avoid injury to the subtending cortex. However, 3D navigation is more difficult to interpret, although it may enable greater precision. In our opinion, the obvious increase in costs for the apparatus and in time would be justified only for less-experienced hand surgeons who can simplify osteosynthesis with the help of navigation.
Acknowledgments
The authors would like to thank PD Dr. Pohlenz from the Department of Oral and Maxillofacial Surgery for kindly providing us with the BrainLAB navigation system and Thum & Wilharm KG for the production of the resting splint for fixation of the forearm.
Declaration of interest: The authors report no conflicts of interest.
References
- McQueen MM, Gelbke MK, Wakefield A, Will EM, Gaebler C. Percutaneous screw fixation versus conservative treatment for fractures of the waist of the scaphoid: A prospective randomised study. J Bone Joint Surg Br 2008; 90: 66–71
- Adams BD, Blair WF, Reagan DS, Grundberg AB. Technical factors related to Herbert screw fixation. J Hand Surg Am 1988; 13: 893–999
- Moran R, Curtin J. Scaphoid fractures treated by Herbert screw fixation. J Hand Surg Br 1988; 13: 453–455
- Kendoff D, Citak M, Gaulke R, Gardner MJ, Geerling J, Krettek C, Hüfner T. [Navigation for placement of scaphoid screws: A new indication for intraoperative 3D navigation – a cadaver study] [in German]. Unfallchirurg 2007; 110: 745–750
- Müller M, Chen ZB, Al Morshidy A, Germann G, Sauerbier M. [Postoperative CT-controlled results of renewed osteosynthesis using screw fixation for acute scaphoid fractures] [in German]. Unfallchirurg 2006; 109: 185–192
- Liverneaux PA, Gherissi A, Stefanelli MB. Kirschner wire placement in scaphoid bones using fluoroscopic navigation: A cadaver study comparing conventional techniques with navigation. Int J Med Robot 2008; 4: 165–173
- Grossterlinden L, Rueger J, Catala-Lehnen P, Rupprecht M, Lehmann W, Rücker A, Briem D. Factors influencing the accuracy of iliosacral screw placement in trauma patients. Int Orthop 2011; 35(9)1391–1396
- Briem D, Rueger JM, Begemann PG, Halata Z, Bock T, Linhart W, Windolf J. [Computer-assisted screw placement into the posterior pelvic ring: Assessment of different navigated procedures in a cadaver trial] [in German]. Unfallchirurg 2006; 109: 640–646
- Briem D, Linhart W, Lehmann W, Begemann PG, Adam G, Schumacher U, Cullinane DM, Rueger JM, Windolf J. Computer-assisted screw insertion into the first sacral vertebra using a three-dimensional image intensifier: Results of a controlled experimental investigation. Eur Spine J 2006; 15: 757–763
- Weinberg AM, Pichler W, Grechenig S, Tesch NP, Heidari N, Grechenig W. The percutaneous antegrade scaphoid fracture fixation – a safe method?. Injury 2009; 40: 642–644
- Adamany DC, Mikola EA, Fraser BJ. Percutaneous fixation of the scaphoid through a dorsal approach: An anatomic study. J Hand Surg Am 2008; 33: 327–331
- Bedi A, Jebson PJ, Hayden RJ, Jacobson JA, Martus JE. Internal fixation of acute, nondisplaced scaphoid waist fractures via a limited dorsal approach: An assessment of radiographic and functional outcomes. J Hand Surg Am 2007; 32: 326–333
- Polsky MB, Kozin SH, Porter ST, Thoder JJ. Scaphoid fractures: Dorsal versus volar approach. Orthopedics 2002; 25: 817–819
- Citak M, O’Loughlin PF, Kendoff D, Suero EM, Gaulke R, Olivier LC, Krettek C, Hüfner T. Navigated scaphoid screw placement using customized scaphoid splint: An anatomical study. Arch Orthop Trauma Surg 2010; 130: 889–895
- Citak M, Board TN, Sun Y, Look V, Krettek C, Hüfner T, Kendoff D. Reference marker stability in computer aided orthopedic surgery: A biomechanical study in artificial bone and cadavers. Technol Health Care 2007; 15: 407–414
- Kendoff D, Bogojevic A, Citak M, Citak M, Maier C, Maier G, Krettek C, Hüfner T. Experimental validation of noninvasive referencing in navigated procedures on long bones. J Orthop Res 2007; 25: 201–207
- Bond CD, Shin AY, McBride MT, Dao KD. Percutaneous screw fixation or cast immobilization for nondisplaced scaphoid fractures. J Bone Joint Surg Am 2001; 83-A: 483–488
- Slade JF III, Gillon T. Retrospective review of 234 scaphoid fractures and nonunions treated with arthroscopy for union and complications. Scand J Surg 2008; 97: 280–289
- Slade JF III, Jaskwhich D. Percutaneous fixation of scaphoid fractures. Hand Clin 2001; 17: 553–574