Abstract
Introduction: Anatomical implants enable minimally invasive osteosynthesis (MIO) and represent ideal complements of computer-assisted surgical workflows. This 3D morphometric study analyzes anatomical implant forms (AIF) for acetabular fracture osteosynthesis (AFO).
Materials and Methods: Three-dimensional pelvis models were created from clinical CT data of 99 European-Caucasian patients (50 females, 49 males). The mean age of the patients was 60.1 years (range: 20–89; SD 10.8). Definition of a referential region of interest (ROI) corresponding to an AIF for AFO was followed by automated ROI computation for each of the 198 hemipelvises. Three-dimensional statistical modeling and analysis of the resulting 198 homologous ROIs consisted of thin-plate spline transformation, generalized Procrustes fit, and principal component analysis.
Results: The mean ROI length was 18.2 cm (range: 16.1–20.1 cm; SD 0.76). The first principal component (PC1) mainly modeled the ROI length, which correlated well with body height (r = 0.325; p < 0.001). PC1 comprised 47.4% of the overall ROI form variation. PC2 primarily influenced the ROI curvature in the anterior-posterior (inlet) view. Curvatures were more pronounced in female patients compared to males (p < 0.001). There was no gender-specific ROI size variation. PC1-4 contained 80.2% of the total ROI form variation. Left and right ROI forms displayed symmetry.
Conclusion: This 3D morphometric study demonstrates the feasibility of anatomical implants for minimally invasive acetabular fracture osteosynthesis. Implant size/length is by far the most important variable of form variation. The necessity of gender-specific implant forms requires further investigation. The non-fractured, contralateral hemipelvis can be used for preoperative surgical planning. Ultimately, the plate design will depend on prospective implant fit tests based on the required fit as defined by the clinician.
Introduction
Acetabular fracture osteosynthesis (AFO) is still associated with a considerable rate of peri-operative complications Citation[1], Citation[2]. Demographic changes in industrial countries have resulted in a steady increase in the number of geriatric patients Citation[1], Citation[3], Citation[4], while the proportion of patients suffering from relevant co-existing morbidities and morbid obesity also continues to rise. Epidemiologic studies predict a substantial increment of acetabular fractures associated with osteoporosis, imposing particular demands on fracture fixation methods Citation[3], Citation[5].
Common peri-operative complications include thromboembolism, hemorrhage, nerve and vessel lesions, infection, fracture malreduction, and implant malplacement Citation[2], Citation[6]. Certain fracture patterns require extended surgical approaches, either to achieve fracture reduction or to obtain and control adequate implant fit. However, extensive surgical exposures involve an increased risk of soft tissue complications Citation[2]. Since anatomical implants (AIs) solve the issue of implant fit, they enable minimally invasive osteosynthesis when fracture reduction can be accomplished by closed or less invasive means Citation[7]. In the case of AFO, AIs could be inserted through selected anatomical windows of a limited ilioinguinal approach, a modified Stoppa approach, or an intra-pelvic approach Citation[8–10]. Thus, minimally invasive osteosynthesis (MIO) could significantly reduce operative morbidity and also lower the rate of intra- and postoperative complications. In addition, AIs ideally complement computer-assisted surgical workflows, which may comprise preoperative planning, computer-aided execution, and postoperative evaluation of the osteosynthesis Citation[11–13]. AIs are routinely and successfully used at various locations, e.g., the proximal and distal humerus, the femur, the proximal and distal tibia, and the orbital floor, but do not yet exist for AFO Citation[14], Citation[15]. One reason for this might be a certain reluctance on the part of healthcare companies in view of the extensive costs associated with development of such implants, while their economic profitability remains questionable. Regardless, the clinical demand will most likely continue to increase with the ongoing pursuit of minimally invasive and computer-assisted solutions for AFO, especially considering the steadily rising number of fractures in patients with a high-risk profile.
Design and development of AIs should rely on state-of-the-art 3D morphometric analysis Citation[16], Citation[17]. Anatomical implant forms (AIFs) represent indistinct (fuzzy) regions of interest (ROIs), which are located on 3D bone surfaces. Three-dimensional statistical modeling and analysis (SMA) represents a standard technique for analyzing forms within complex 3D environments. Previously, 3D SMA provided the morphometric fundamentals with regard to the design of AIs for orbital floor fracture treatment Citation[18].
This study investigated the morphometric fundamentals of AIFs for minimally invasive AFO. For this purpose, we analyzed inter-individual variation of ROI forms corresponding to their respective AIFs. Three-dimensional form (i.e., shape and size) analysis was accomplished by means of CT-based SMA. Furthermore, gender- and side-specific ROI form variations were examined with respect to their importance in implant design and preoperative surgical planning.
Materials and methods
The study included clinical CT (DICOM) data of 99 pelvises (50 females, 49 males). The data were obtained during routine or emergency diagnostic procedures (Somatom Sensation 64 CT scanner, Siemens Healthcare, Forchheim, Germany). A reconstruction kernel of B45f was used for optimal bone visualization. The slice distance and image resolution was 0.6–1.0 mm. For the purposes of the study, only adult European-Caucasian patients with no bony pathology of the pelvis were eligible. The mean age of the patients was 60.1 years (range: 20–89; SD 10.8). All patients agreed to the anonymous research use of their CT data and provided written informed consent prior to inclusion in the study. The local Ethics Committee approved the study, which met all ethical standards as defined in the 1964 Declaration of Helsinki.
An expert performed semi-automatic segmentation of the CT data using AMIRA® software (Version 5, Visage Imaging, Inc., San Diego, CA). Both ilia were segmented apart from the sacrum. Triangulated surfaces of the left and right ilium were produced, then the triangulated surfaces were smoothed and pre-processed to obtain regular surfaces without holes and triangle intersections. STL files were produced by an AMIRA® script, which processed the segmented LabelField with a 3D median filter. AMIRA® generated 3D hemipelvis surface models, and a batch post-processing script in Geomagic Studio® (Geomagic U.S. Corp., Research Triangle Park, NC) cultivated the surfaces of these models. Each surface was cleaned twice, spikes were removed, and holes were filled.
We introduced the instrument of a “smallest anatomically definable area” (SADA) containing the surgically relevant ROI. The expert defined ten distinct anatomical landmarks representing fixed corners of the SADA and delineated its contour by setting gliding segment landmarks (). The SADA consisted of ten segments, each defined by a pair of indices of the corresponding anatomical landmarks and a number of equidistant gliding segment landmarks. This segment structure was defined in a text file, which could be read by the AMIRA® script object. This script produced equidistant segment landmarks along the contour of the SADA without moving the distinct anatomical landmarks (). Automated computation resulted in 198 corresponding (homologous) SADAs.
Figure 1. (A) Ten distinct anatomical landmarks and intermediate gliding segment landmarks are manually placed and delineate the borders of the SADA. (B) Equidistant landmarks are computed while the 10 distinct anatomical landmarks remain fix.
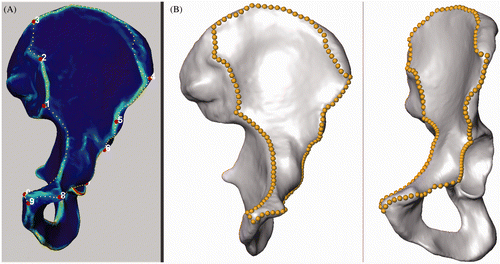
An experienced trauma surgeon (W.K.) defined the midline of an ideally positioned plate within an arbitrary SADA (the reference SADA). Further landmarks were manually placed parallel to this midline at distances of 0.25 mm and 0.5 mm (). Thus, a landmark set consisted of five landmarks in a row. An AMIRA® script object read the landmark files and produced a triangulated ROI surface structure. The ROI represented the 3D morphometric correspondence of an AIF with an approximate width of 10 mm (). Subsequently, the reference ROI was used to create corresponding (homologous) ROIs for each given SADA.
Figure 2. (A) The reference ROI featuring a triangulated surface structure is situated in a left hemipelvis. (B) The dark line represents the midline of an ideal anatomical implant as defined by the expert. Parallel lines are placed at distances of 2.5 and 5 mm, resulting in a virtual plate with an approximate width of 10 mm. The triangulated ROI surface is built from landmark sets, each consisting of the five landmarks of one row.
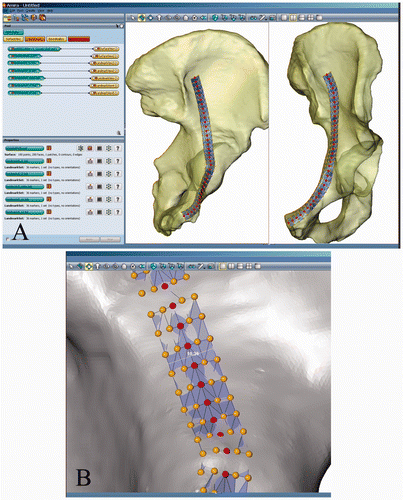
Thin-plate spline (TPS) deformation is a landmark-based elastic method routinely used for medical image registration Citation[19]. TPS functions as a 3D warp that transforms n points to exactly n corresponding points. Intermediate points are interpolated with use of minimal bending energy. An AMIRA® script object for automated TPS deformation was created. The script read the landmarks of the triangulated surface of the reference ROI and the reference SADA. TPS deformation elastically mapped the reference ROI onto each of the 197 remaining SADAs (target SADAs) by exactly matching their landmarks. Then, from each deformed triangle point of the reference ROI, the closest point on the surface of the target SADA was computed. Automated repetition of this process produced a set of 198 homologous ROIs.
Shape alignment in medical image analysis often needs to consider inter-individual size differences because of their particular relevance to certain clinical applications, as in the case of implant design Citation[20]. We chose 3D SMA as a standard method of form (shape and size) analysis because it preserves inter-individual size variation.
All right-side ROIs were mirrored to simplify the analysis. Procrustes alignment minimizes the sum of squared differences between homologous points, and can be calculated analytically Citation[21]. Procrustes alignment was run iteratively on the MATLAB® software (MathWorks®, Natick, MA). Generalized Procrustes alignment (GPA) produced 198 aligned, unscaled homologous ROIs. There were no obvious outliers.
In a subsequent step, the dimensionality of form variation was reduced by principal component analysis (PCA) Citation[22]. Following GPA, the principal components of form variation were computed. Computation and visualization of the results were realized using MATLAB® and AMIRA® software. The ROI size was specified by the Frobenius norm, a common size measure in multivariate statistics. We performed Pearson correlations (r = Pearson coefficient) to describe the relationship between the Frobenius norm and the respective PCs. The Wilcoxon rank-sum test compared groups of ROI forms. The alpha level was 0.05 and statistical significance was assumed at p-values < 0.05.
Results
illustrates the mean ROI form enveloped by a hull containing all 198 homologous ROI forms. The mean ROI length was 18.2 cm, the minimum ROI length was 16.1 cm, and the maximum ROI length was 20.1 cm (SD 0.8 cm). The Lilliefors test showed a Gaussian (normal) distribution of ROI lengths.
Figure 3. The mean ROI form (dark grey) is visualized within an envelope (light grey) containing all 198 homologous ROI forms. (A) Anterior-posterior (inlet) view. (B) Lateral view.
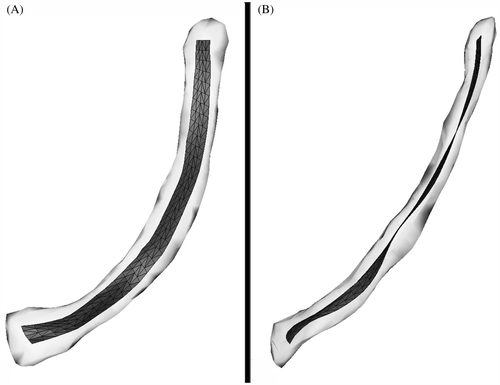
demonstrates the excellent fit of a synthetic rapid prototype model of the mean ROI form to its site of implantation using the example of an arbitrary, customary SYNBONE® hemipelvis model (SYNBONE AG, Malans, Switzerland).
Figure 4. A synthetic rapid prototype model of the mean ROI, featuring an excellent fit to an arbitrary, customary SYNBONE® hemipelvis model. (A) Anterior-posterior (inlet) view. (B) Lateral view.
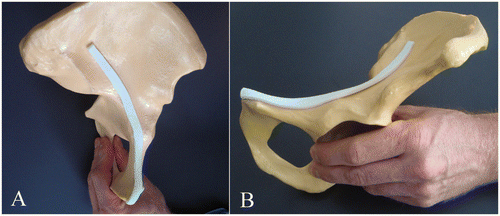
The first principal component (PC1) mainly modeled the ROI length. PC1 comprised 47.4% of the overall form variation. clearly shows the linear relationship between PC1 and the Frobenius norm as a quantitative measure of ROI size (r = 0.985; p < 0.01).
Figure 5. In this scatter plot, there is an almost linear relationship between PC1 and the Frobenius norm as a measure of ROI size. Females (cyan dots) and males (red dots) distribute equally along the abscissa.
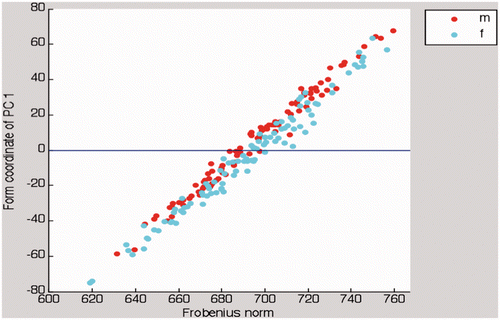
PC2 primarily modified the extent of the ROI curvature in the anterior-posterior (inlet) view. PC2 covered 18.8% of the total anatomical variance. There was no significant correlation between PC2 and the Frobenius norm. The Pearson correlation coefficient (r) was 0.170 (p = 0.017).
PC3 slightly altered the ROI flexion slightly at its anterior and posterior ends. The changes in flexion were most prominent in the lateral view. PC3 accounted for 8.7% of the total form variation. There was no significant correlation between PC3 and the Frobenius norm (r = 0.027; p = 0.702).
PC4 slightly altered the ROI torsion slightly at its anterior and posterior ends. These alterations of torsion could best be visualized in the lateral view. PC4 covered 5.3% of the total anatomical form variation. The Pearson correlation coefficient (r) between PC4 and the Frobenius norm was 0.008 (p = 0.906).
We calculated the proportions contributed by each single PC to the overall anatomical form variation. gives the numeric values as cumulative percentages for the first 15 PCs. PC1 already accounted for 47.4%, and PC1-4 for 80.2% of the total variance.
Table I. Cumulative percentages of total form variation (PC1-15)
Highly significant gender-specific ROI form variation was observed (p < 0.001). The ROI curvature was more pronounced in females than in males; males exhibited a rather straightened ROI shape. The scatter plot in illustrates clear clustering of sexes related to PC2; however, there was no gender-specific ROI size variation, as the Frobenius norms of female and male ROIs were not significantly different (p = 0.391).
Figure 6. In this scatter plot, there is clear clustering of sexes related to PC2. As in , females (cyan dots) and males (red dots) distribute equally along the abscissa.
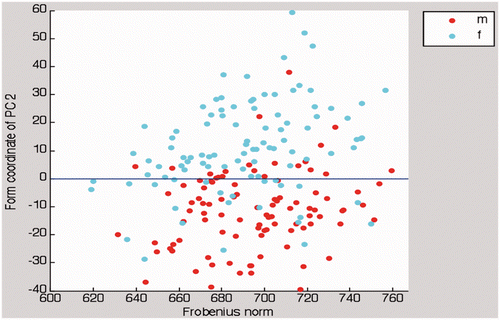
The ROI forms displayed symmetry: There were no differences in anatomical form variation between left and right ROIs. PCA did not reveal side-specific ROI shape variation (p = 0.151 for PC1, p = 0.084 for PC2). Also, the Frobenius norms of left and right ROIs were not statistically different (p = 0.508).
Pearson correlation of biometric data with ROI length yielded significance for body height (r = 0.325; p < 0.001). There was no significant correlation for body weight (r = 0.102; p = 0.185) or body mass index (r = −0.048; p = 0.533).
Discussion
This CT-based 3D form analysis of the human pelvis provides the morphometric fundamentals for the design of anatomical implants with regard to minimally invasive acetabular fracture osteosynthesis. For this purpose, we developed a novel application of 3D SMA which represents a state-of-the-art method for analysis of form (shape and size) variation. Morphometric analysis of an anatomically indistinct region within a complex 3D surface posed several technical and methodological challenges. Anatomical correspondence was created by the introduction of a ROI exhibiting a defined triangulated surface. The ROI was located within a segment-structured SADA, which in turn was confined by distinct anatomical landmarks. Thin-plate spine deformation automatically created a ROI for each given SADA. Since anatomical correspondence was given, all 198 ROIs were homologous and comparable to one another. Simultaneously, inter-individual size differences were maintained. Previously, similar methods were employed to analyze fuzzy ROIs of the human orbit Citation[14], Citation[18].
As in previous landmark-based morphometric studies, inter-individual size differences accounted for the highest proportion of total anatomical form variation Citation[23]. These results suggest the necessity of various implant lengths. A set of AIs for minimally invasive AFO should contain plates of different length categories. Alternatively, the surgeon could remove excess plate holes pre- or intraoperatively according to individual requirements. AIFs exhibited clear gender-related shape specificity, with more pronounced curvatures being observed in female implant shapes as compared to those of males. These findings are consistent with previous anatomical studies of sexual dimorphism of the human pelvis showing larger transverse diameters in female pelvises Citation[24]; however, the clinical requirement for a gender-specific implant design requires further investigation. The first four PCs accounted for 80.2% of the overall form variation. Anatomical studies of other skeletal regions yielded comparable variances of PCA. In a CT-based PCA of the clavicle, PC1-4 comprised approximately 87% of the overall anatomical variation Citation[23]; though, the sample size was rather small (n = 21). In our study, left and right ROI forms displayed symmetry. ROI form symmetry indicates that both preoperative planning and choice of the implant can be based on the non-fractured, contralateral hemipelvis in cases of unilateral acetabular fracture.
AIs can be inserted via less or minimally invasive approaches. The clinical feasibility and safety of limited approaches in osteosynthesis of acetabular and pelvic fractures has been shown previously Citation[8], Citation[25], Citation[26]. Percutaneous fracture reduction can be achieved employing Schanz screws as joysticks for fragment manipulation Citation[26]. Furthermore, a limited Stoppa approach potentially combined with the first window of a classical ilioinguinal approach is qualified for minimally invasive AFO Citation[8]. In orthopaedic and trauma surgery, the importance of preoperative planning is steadily increasing. This is particularly true for MIO of complex fractures, e.g., acetabular fractures, where access to the fracture site is anatomically demanding Citation[11], Citation[27]. Reliable tools already exist for virtual fracture reduction Citation[27], Citation[28]. AIs enable detailed virtual planning of all relevant parameters of the osteosynthesis, including the position and length of plates and screws Citation[11]. Computer-assisted osteosynthesis can be realized and controlled by 3D navigation tools Citation[26], Citation[29], Citation[30].
The study has some limitations. As it only included patients of European-Caucasian descent, we cannot comment on race-related anatomical form variation Citation[31]: An extended multi-center study design should include patients of different ethnicities. To a certain extent, the ideal implant position depends on personal preference. However, from a methodological point of view, the implant position may be regarded as arbitrary. The presented method can be applied to any ROI located within the SADA. It must be emphasized that the ROI forms corresponded to AIFs and did not represent the definitive AIs.
Ultimately, the design of anatomical implants depends on the results of implant fit tests which consider the required implant fit as defined by the clinician. Implant fit tests often rely on synthetic rapid prototype models or cadaver bones. Virtual tests address the practicability, accuracy and long-term cost-effectiveness, particularly when performed semi- or fully automatically Citation[32], Citation[33]. Such tests should resort to large databases covering the anatomical variation of a representative population. In the more distant future, controlled clinical studies should evaluate whether the use of anatomical implants reduces operative morbidity and complications, shortens periods of hospitalization and rehabilitation, and improves clinical and radiological outcomes.
Acknowledgments
The authors thank all the assistants from the Department of Diagnostic Radiology and Medical Physics. This work is dedicated to our friend and colleague Klaus Bräun, who lost his life in a tragic avalanche accident.
Declaration of interest: The project was supported by the AO Research Fund of the AO Foundation, Davos, Switzerland (AO Research Grant F-07-71-S).
References
- Porter SE, Russell GV, Dews RC, Qin Z, Woodall Jr J, Graves ML. Complications of acetabular fracture surgery in morbidly obese patients. J Orthop Trauma 2008; 22(9)589–594
- Russell GV Jr, Nork SE, Chip Routt ML Jr. Perioperative complications associated with operative treatment of acetabular fractures. J Trauma 2001; 51(6)1098–1103
- Guggenbuhl P, Meadeb J, Chalès G. Osteoporotic fractures of the proximal humerus, pelvis, and ankle: epidemiology and diagnosis. Joint Bone Spine 2005; 72(5)372–375
- Melton LJ, 3rd, Sampson JM, Morrey BF, Ilstrup DM. Epidemiologic features of pelvic fractures. Clin Orthop Relat Res 1981;, 155: 43–47
- Vanderschot P. Treatment options of pelvic and acetabular fractures in patients with osteoporotic bone. Injury 2007; 38(4)497–508
- Mayo KA, Letournel E, Matta JM, Mast JW, Johnson EE, Martimbeau CL. Surgical revision of malreduced acetabular fractures. Clin Orthop Relat Res 1994;, 305: 47–52
- Weise K. [30 years of osteosynthesis: developments in surgical fracture treatment over the last three decades] [In German]. Orthopade 2010; 39(2)122–131
- Andersen RC, O’Toole RV, Nascone JW, Sciadini MF, Frisch HM, Turen CW. Modified stoppa approach for acetabular fractures with anterior and posterior column displacement: quantification of radiographic reduction and analysis of interobserver variability. J Orthop Trauma 2010; 24(5)271–278
- Starr AJ, Jones AL, Reinert CM, Borer DS. Preliminary results and complications following limited open reduction and percutaneous screw fixation of displaced fractures of the acetabulum. Injury 2001; 32(Suppl 1)SA45–SA50
- Wolf H, Wieland T, Pajenda G, Vecsei V, Mousavi M. Minimally invasive ilioinguinal approach to the acetabulum. Injury 2007; 38(10)1170–1176
- Cimerman M, Kristan A. Preoperative planning in pelvic and acetabular surgery: the value of advanced computerised planning modules. Injury 2007; 38(4)442–449
- Ochs BG, Gonser C, Shiozawa T, Badke A, Weise K, Rolauffs B, Stuby FM. Computer-assisted periacetabular screw placement: Comparison of different fluoroscopy-based navigation procedures with conventional technique. Injury 2010; 41(12)1297–1305
- Koestler W, Sidler R, González Ballester MA, Nolte LP, Suedkamp NP, Maier D. A feasibility study of computer-assisted bone graft implantation for tissue-engineered replacement of the human ankle joint. Comput Aided Surg 2008; 13(4)207–217
- Kamer L, Noser H, Schramm A, Hammer B. Orbital form analysis: problems with design and positioning of precontoured orbital implants: a serial study using post-processed clinical CT data in unaffected orbits. Int J Oral Maxillofac Surg 2010; 39(7)666–672
- Südkamp N, Bayer J, Hepp P, Voigt C, Oestern H, Kääb M, Luo C, Plecko M, Wendt K, Köstler W, Konrad G. Open reduction and internal fixation of proximal humeral fractures with use of the locking proximal humerus plate. Results of a prospective, multicenter, observational study. J Bone Joint Surg Am 2009; 91(6)1320–1328
- Bookstein FL. Landmark methods for forms without landmarks: morphometrics of group differences in outline shape. Med Image Anal 1997; 1(3)225–243
- Heimann T, Meinzer HP. Statistical shape models for 3D medical image segmentation: a review. Med Image Anal 2009; 13(4)543–563
- Noser H, Hammer B, Kamer L. A method for assessing 3D shape variations of fuzzy regions and its application on human bony orbits. J Digit Imaging 2010; 23(4)422–429
- Paulsen RR, Hilger KB. Shape modelling using Markov random field restoration of point correspondences. Inf Process Med Imaging 2003; 18: 1–12
- Neumann A, Lorenz C. Statistical shape model based segmentation of medical images. Comput Med Imaging Graph 1998; 22(2)133–143
- Golland P, Grimson WE, Shenton ME, Kikinis R. Detection and analysis of statistical differences in anatomical shape. Med Image Anal 2005; 9(1)69–86
- Jolicoeur P, Mosimann JE. Size and shape variation in the painted turtle. A principal component analysis. Growth 1960; 24: 339–354
- Daruwalla ZJ, Courtis P, Fitzpatrick C, Fitzpatrick D, Mullett H. An application of principal component analysis to the clavicle and clavicle fixation devices. J Orthop Surg Res 2010; 5: 21
- Correia H, Balseiro S, De Areia M. Sexual dimorphism in the human pelvis: testing a new hypothesis. Homo 2005; 56(2)153–160
- Cole JD, Bolhofner BR. Acetabular fracture fixation via a modified Stoppa limited intrapelvic approach. Description of operative technique and preliminary treatment results. Clin Orthop Relat Res 1994;, 305: 112–123
- Crowl AC, Kahler DM. Closed reduction and percutaneous fixation of anterior column acetabular fractures. Comput Aided Surg 2002; 7(3)169–178
- Fornaro J, Keel M, Harders M, Marincek B, Székely G, Frauenfelder T. An interactive surgical planning tool for acetabular fractures: initial results. J Orthop Surg Res 2010; 5: 50
- Hüfner T, Pohlemann T, Tarte S, Gänsslen A, Citak MSM, Bazak N, Culemann U, Nolte LP, Krettek C. Computer-assisted fracture reduction: novel method for analysis of accuracy. Comput Aided Surg 2001; 6(3)153–159
- Stöckle U, Schäffler A, König B, Haas NP. [Integrated navigation. Preclinical evaluation and initial clinical experience]. Unfallchirurg 2006; 109(11)925–931
- Kendoff D, Gardner MJ, Citak M, Kfuri M Jr, Thumes B, Krettek C, Hüfner T. Value of 3D fluoroscopic imaging of acetabular fractures comparison to 2D fluoroscopy and CT imaging. Arch Orthop Trauma Surg 2008; 128(6)599–605
- Patriquin ML, Steyn M, Loth SR. Metric assessment of race from the pelvis in South Africans. Forensic Sci Int 2002; 127(1–2)104–113
- Schmutz B, Wullschleger ME, Kim H, Noser H, Schütz MA. Fit assessment of anatomic plates for the distal medial tibia. J Orthop Trauma 2008; 22(4)258–263
- Kozic N, Weber S, Büchler P, Lutz C, Reimers N, González Ballester MA, Reyes M. Optimisation of orthopaedic implant design using statistical shape space analysis based on level sets. Med Image Anal 2010; 14(3)265–275