Abstract
Background: Resection of bone tumors within the pelvis requires good cutting accuracy to achieve satisfactory safe margins. Manually controlled bone cutting can result in serious errors, especially due to the complex three-dimensional geometry, limited visibility, and restricted working space of the pelvic bone. This experimental study investigated cutting accuracy during navigated and non-navigated simulated bone tumor cutting in the pelvis.
Methods: A periacetabular tumor resection was simulated using a pelvic bone model. Twenty-three operators (10 senior and 13 junior surgeons) were asked to perform the tumor cutting, initially according to a freehand procedure and later with the aid of a navigation system. Before cutting, each operator used preoperative planning software to define four target planes around the tumor with a 10-mm desired safe margin. After cutting, the location and flatness of the cut planes were measured, as well as the achieved surgical margins and the time required for each cutting procedure.
Results: The location of the cut planes with respect to the target planes was significantly improved by using the navigated cutting procedure, averaging 2.8 mm as compared to 11.2 mm for the freehand cutting procedure (p < 0.001). There was no intralesional tumor cutting when using the navigation system. The maximum difference between the achieved margins and the 10-mm desired safe margin was 6.5 mm with the navigated cutting process (compared to 13 mm with the freehand cutting process).
Conclusions: Cutting accuracy during simulated bone cuts of the pelvis can be significantly improved by using a freehand process assisted by a navigation system. When fully validated with complementary in vivo studies, the planning and navigation-guided technologies that have been developed for the present study may improve bone cutting accuracy during pelvic tumor resection by providing clinically acceptable margins.
Introduction
Surgical procedures for pelvic bone tumors are challenging due to the geometrical complexity of the pelvic bone and the proximity of delicate organs and structures such as the bladder, rectum, sciatic nerve and numerous vessels Citation[1], Citation[2]. Tumor resection requires good cutting accuracy to achieve satisfactory margins Citation[3-8]. Local recurrence rates in the pelvis, as reported in the literature, can be very high, ranging from 30 to 90% Citation[9-11].
A preliminary study wherein four experienced surgeons each simulated three different tumor resections on a pelvic bone model emphasized a significant lack of accuracy with the conventional (freehand) bone-cutting procedure Citation[12]. Under ideal working conditions on an experimental test bed (complete visibility and accessibility of the bony surface), the errors in the 10-mm desired safe margin averaged 5.3 mm. In addition, two (17%) of the 12 resections were intralesional with a 5-mm negative margin. The complex three-dimensional (3D) geometry of the pelvic bone aggravated the manually controlled cutting errors.
Since the 2000s, computer-assisted technologies have been developed for pelvic bone tumor surgeries with the main objective of improving bone-cutting accuracy Citation[13-15]. Several planning and navigation systems are available, and surgeons are now benefiting from a real-time navigated process for the positioning of surgical cutting tools such as osteotomes Citation[16], chisels Citation[17], drills Citation[18], Citation[19], burrs Citation[20], Citation[21] and oscillating saws Citation[22-24]. These clinical studies have demonstrated the feasibility of achieving adequate (tumor-free) resection margins within the pelvis.
To date, however, few clinical studies have reported quantitative data on achieved margins during navigation-assisted bone tumor resections Citation[15], Citation[25], Citation[26]. Examples of data reported include maximum differences ranging from 4 to 10 mm between the preoperatively planned margins and those visualized on postoperative CT or excised histological actual margins.
An experimental study, designed to be independent of any surgical application, simulated bone-cutting on a geometrically simple (rectangular) bone model and assessed quantitatively the added value of the navigation technology Citation[27]. The results indicated that navigated bone-cutting errors were two times smaller when compared with a solely freehand process. However, no studies have yet compared the accuracy of navigation-assisted bone tumor cutting of the pelvis to that of a conventional (freehand) procedure in order to assess quantitatively the added value of the navigation technology.
This experimental study aimed at investigating cutting accuracy during navigated and non-navigated simulated bone tumor cutting in the pelvis.
Materials and methods
Experimental set-up
Twenty-three operators, comprising 10 experienced surgeons (senior) and 13 residents in training (junior), performed simulated bone tumor cutting. The experiments were conducted using synthetic bones made from solid rigid polyurethane closed foam Citation[28] (Sawbones, Vashon, WA). The cuts were performed using a pneumatic oscillating saw (Compact Air Drive II, Synthes, Solothurn, Switzerland) equipped with a blade 60 mm long, 25 mm wide, and 0.9 mm thick (Synthes, Solothurn, Switzerland). The simulated bones were right hemipelvic bone models with an acetabulum 57 mm in diameter and a dimensional tolerance of 0.5 mm, according to the manufacturer. The test bed consisted of a clamping device and a reference block (40 × 40 × 40 mm, with a dimensional tolerance of 0.05 mm) (). The clamping device consisted of two template supports to rigidly fix the bones at the lower (ischium) and upper (iliac crest) extremities by means of screws. This device enabled clamping of each bone being cut at the same position relative to the reference block. Finally, a global reference frame (R0) fixed to the reference block was defined considering three faces of the block as the XY, YZ and ZX planes.
Figure 1. Bone cutting performed with an oscillating saw. The simulated bone is a polyurethane foam right hemipelvic model and is clamped by means of template supports and screw fixation. The reference block is a plastic POM-C resin block and is used to define the global reference frame R0.
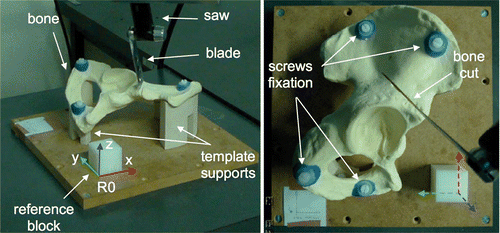
The test bed was scanned using a computed-tomography (CT) scanner (SOMATOM Definition AS, Siemens AG, Germany) with two-dimensional (2D) slices of 1.5-mm thickness and a 1.5-mm step in the frontal direction (the X axis of R0). A virtual 3D CT model of the test bed was reconstructed using in-house validated software for the planning of the bone tumor resection Citation[29]. In the 3D CT model, a geometrically simple bone tumor was simulated by a 75-mm sphere centered on the acetabulum, involving zone II of the Enneking classification Citation[30]. Finally, the frame R0 was defined in the 3D CT model by computationally identifying the XY, YZ and ZX planes of the reference block.
During the experiments, each operator accomplished sequentially the same three-step protocol: preoperative planning of the tumor cutting, freehand cutting, and navigation-assisted cutting. The details are presented in the following subsections.
Preoperative planning of the tumor cutting
Each operator completed the planning of the tumor cutting by using the planning software (). The software provided 2D and 3D visualization of the 3D CT model of the hemipelvic bone and enabled the cutting planes to be positioned close to the boundary of the tumor with a 10-mm safety margin Citation[29]. Each planning procedure consisted of four target planes defining the desired bone cutting, including a first plane in the ischium, a second plane in the pubis, and a third and fourth plane forming two angular cuts in the ilium. In practice, the planning of each operator was recorded by storing the plane coordinates expressed in the reference frame R0. Finally, each operator was free to define geometrical landmarks on the 3D CT model of the hemipelvic bone by using a distance measurement tool provided by the planning software.
Figure 2. Preoperative planning of tumor cutting on the 3D CT model of the hemipelvic bone. The tumor is simulated by a sphere centered on the acetabulum. The planning consists of four target planes defining the desired cutting of the simulated tumor, including a first plane in the ischium, a second plane in the pubis, and a third and fourth plane forming two angular cuts in the ilium. The plane coordinates are expressed in the reference frame R0.
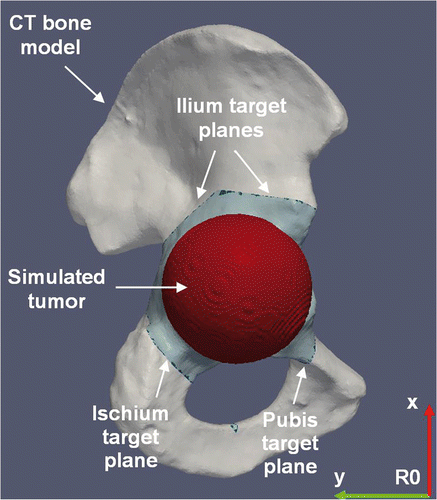
Freehand bone cuts
First, the 23 operators individually performed four cuts, one in each of their own four target planes. Before the cuts, each operator was free to mark the hemipelvic bone using a caliper and pencil to transfer their preoperative planning on the basis of the distance measurements previously defined on the 3D CT model. To simulate clinically relevant positioning of the patient on the operating table, the operators were allowed to fix the hemipelvic bone using a steel clamp with a 360° rotating base. Operators were instructed to respect the target planes as accurately as possible so as to avoid intralesional tumor cutting.
Navigated bone cuts
The 23 operators performed the bone cuts with the aid of a navigation system (Surgetics®, Praxim, Grenoble, France). The 3D CT model of the hemipelvic bone was loaded into the system. The spine module of the navigation system was adapted for direct navigation of the oscillating saw blade Citation[27].
Similarly, the operators were allowed to fix the hemipelvic bone by a base-rotating clamp to simulate the patient decubitus positioning. Before the navigated cuts, each operator was also allowed to fix a dynamic reference base (DRB) to the bone. This DRB was equipped with infrared-reflecting markers and was tracked by the optical localizer of the navigation system (Polaris®, NDI, Waterloo, Ontario, Canada; accuracy of 0.5 mm and 0.5° according to the manufacturer). The operator registered the bone with the 3D CT model using semi-automatic surface-based algorithms provided by the navigation system. Previous experiments had assessed a global surface-based registration accuracy of 1.3 mm Citation[31].
For each navigated cut, the oscillating saw was also equipped with infrared-reflecting markers and tracked by the Polaris localizer. Using the tracking information, the navigation system was programmed for real-time computing of the position of the oscillating saw relative to the hemipelvic bone. The operators performed the cuts with real-time visual feedback displayed on a monitor screen ().
Figure 3. Navigated freehand cutting process within the pelvis. (A) While the oscillating saw is tracked in the operating room by an optical localizer, the real-time visual feedback consists of continuously refreshing both the position and orientation of the saw blade relative to the 3D CT model of the simulated bone. The target plane is represented by a red line. The blade is represented by a green rectangle delimited by a yellow line (the distal extremity of the blade) and a blue line (the proximal extremity of the blade). (B) The distal extremity of the blade is positioned on the bone surface on the target plane since the yellow line overlaps the red line. (C) The blade is correctly aligned on the target plane since the red, yellow and blue lines overlap perfectly.
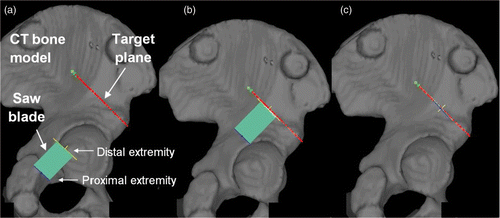
Evaluation of freehand and navigated bone cuts
A dataset of 184 cut planes was available for evaluating the cutting accuracy, including 92 cut planes performed using the solely freehand cutting process and 92 cut planes performed using the navigated process.
Three parameters were defined to evaluate the cutting accuracy (). The location (L) and flatness (F) parameters were used to evaluate the geometrical accuracy of the cut planes in accordance with the ISO1101 standard Citation[32]. L refers to the position of the cut plane with respect to the target plane and is defined as the maximum distance in millimeters between the cut plane and the target plane, as shown in previous experiments Citation[33]. F represents the form of the cut plane and is defined as the minimum distance in millimeters between two parallel planes that include the cut plane. Finally, the surgical margin (SM) evaluated the accuracy of the bone cut relative to the simulated bone tumor. SM is defined as the minimum distance in millimeters between the cut plane and the boundary of the simulated tumor. Consequently, the errors in the 10-mm desired safe margin were defined as the difference in millimeters between SM and 10 mm.
Figure 4. Parameters for the geometrical and surgical evaluation of each cut plane of a tumor cutting. (A) Location (L) (mm) and surgical margin (SM) (mm); the target plane is the dashed line; the cut plane is the solid line. *The cut plane is represented here by a line instead of a curve for better clarity in the definition of L and SM. See main text for details. (B) Flatness (F) (mm); the cut plane is the solid curve.
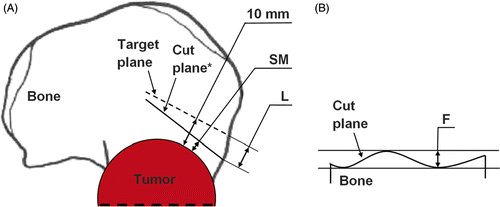
Each cut plane was first digitized with a resolution of 1 µm using a coordinate measuring machine (Signum® SL, Mycrona, Elgin, IL) with a 2-mm spherical sensor. The digitization of the cut planes was performed following the guidelines for an ISO-based assessment of location and flatness parameters Citation[34]. First, the 2-mm sensor was configured to ignore microscopic properties such as roughness, and second, the minimum cut-plane dataset comprised 20 measurement points. This coordinate set was then fitted to a least square plane, a common procedure in checking ISO parameters, and the parameters L, F and SM were calculated using numerical computation software (MATLAB®, The MathWorks, Natick, MA).
Finally, the time required for the freehand and navigated cuts was reported. The mean time for freehand cutting of the simulated bone tumor was defined as the sum of the mean times required for the preoperative planning, landmark measurement, marking of the simulated bone, and freehand cutting. The mean time for the navigated cutting of the simulated bone tumor was defined as the sum of the mean times required for the preoperative planning, bone registration, and navigated cutting.
Statistical analysis
Before the experiments, the minimum required sample size was defined to have a 95% chance of detecting a difference at the 5% level of significance, as described in reference Citation[35]. Assuming that the absolute-value error in the 10-mm desired safe margin would average approximately 5 mm (standard deviation: 5 mm) with a solely freehand cutting procedure Citation[12], and expecting that this error could decrease by a factor of two with use of the navigation technology Citation[25], the minimum required sample size was 45.
A mixed model Citation[36] was performed to evaluate the effect of the categorical variables “cutting process” (freehand or navigated cutting process) and “group of operator” (senior or junior surgeon). These categorical variables were considered fixed effects. Statistical differences between mean values of the response (numerical) variables of the mixed model, including the location and flatness of the cut planes and the surgical margin, were determined by Fisher's tests. p-values less than 0.05 were considered statistically significant. Results are presented as the mean and 95% confidence interval (CI).
In the mixed model, the variable “operator” (the 23 surgeons) was nested within the variable “group of operator”, and statistical differences among each group of operators were investigated. Finally, as the operators were required to perform the tumor cutting with a fixed number of four cuts, the categorical variable “cut plane” was taken into account as a fixed effect in the mixed model, but this variable was not highlighted in the statistical results.
Results
The location of the cut planes with respect to the target planes was significantly improved by the navigated cutting procedure. The location averaged 2.8 mm (95% CI, 2.4 to 3.2 mm) for the navigated cuts, compared to 11.2 mm (95% CI, 9.0 to 13.3 mm) for the freehand cuts (p < 0.001) for all surgeons. For senior surgeons, the location averaged 3.2 mm (95% CI, 2.7 to 3.8 mm) for the navigated cuts, compared to 10.8 mm for the freehand cuts (95% CI, 8.0 to 13.6 mm) (p < 0.001). For junior surgeons, the location averaged 2.4 mm (95% CI, 1.8 to 3.1 mm) for the navigated cuts, compared to 11.4 mm (95% CI, 7.9 to 15.0 mm) (p < 0.001).
The flatness of the cut planes did not vary significantly, averaging 1.0 mm (95% CI, 0.7 to 1.4 mm) for the freehand cuts and 0.8 mm (95% CI, 0.5 to 1.3 mm) for the navigated cuts (p = 0.5073) for all surgeons. The p-values for senior and junior surgeons were 0.5171 and 0.0858, respectively.
The achieved surgical margins were not subject to significant variations in terms of the mean and median values. The achieved margins averaged 9.8 mm (95% CI, 8.3 to 11.3 mm) for the freehand cuts and 10.5 mm (95% CI, 10.0 to 11.0 mm) for the navigated cuts (p = 0.4598) for all surgeons. The p-values for senior and junior surgeons were 0.9546 and 0.2382, respectively. The median value was 10.0 mm for the freehand cuts and 10.4 mm for the navigated cuts for all surgeons. However, the standard deviation of the achieved surgical margins was greatly decreased by the navigated cutting procedure (2.2 mm for the navigated cuts versus 5.5 mm for the freehand cuts). The maximum difference between the planned and achieved surgical margins was 6.5 mm with the navigated cutting process (compared to 13 mm with the freehand process). Finally, there was no intralesional tumor cutting with the navigated procedure, whereas for the freehand procedure five of the 23 tumor cuttings (22%) were intralesional.
The time required to perform the cutting of the simulated bone tumor averaged 49 min (range: 28 to 82 min) for the navigated procedure, compared to 58 min (range: 38 to 85 min) for the freehand procedure. The planning of the four desired cuts around the simulated bone tumor required an average time of 6 min (range: 1 to 16 min). Planning transfer before freehand bone cutting (distance measurements and bone landmarking with a pencil) averaged 19 min (range: 8 to 39 min) and planning transfer before navigated bone cutting (image-to-patient registration) averaged 16 min (range: 5 to 43 min). The time required to perform the bone cuts averaged 33 min (range: 15 to 57 min) for the freehand procedure and 27 min (range: 15 to 43 min) for the navigated procedure.
There was no difference between the senior and junior surgeons with respect to bone-cutting performances, including the location of the cut planes, their flatness, and the surgical margin, as well as the planning and operative times. Four of the five intralesional tumor cuttings observed during the freehand process were performed by junior surgeons and one by a senior surgeon.
Discussion
This experimental study found significant improvements in accuracy when using a navigation system during cutting of a simulated pelvic bone tumor with an oscillating saw. The results, in terms of the location parameter, demonstrate how a navigation system may replicate a preoperative bone-cutting planning on a pelvic structure with an accuracy significantly greater than that of a solely freehand process.
The flatness of the cut planes was not improved by the navigated cutting procedure, reflecting the fact that all bone cuts were performed with the same oscillating saw. Flatness errors could therefore be considered to be independent of the level of technological assistance integrated with the freehand cutting process.
The results, in terms of the mean and median values of the surgical margin parameter, reflect the fact that all the achieved margins were uniformly distributed around the 10-mm desired safe margin and were unbiased. The two cutting procedures that were designed for the present experimental study appear to be neither conservative nor non-conservative, by systematically inducing the surgeons to perform the bone cuts above or below the safety margins defined preoperatively around the simulated tumor.
The errors in the 10-mm desired safe margins of the simulated tumor were greatly decreased with the navigated cutting procedure. The results, in terms of the standard deviation of the achieved surgical margins, suggest that the manually controlled cutting errors can be much smaller when using a navigation system. The results in terms of the maximum difference between the planned and the achieved surgical margins are concurrent with recent clinical studies Citation[25], Citation[26] that reflect the fact that the navigation technology is capable of enabling tumor-free (extralesional) resections.
The operative times measured in the present study (for planning transfer and cutting of the simulated bone tumor) are consistent with the findings of recent clinical studies that reported planning transfer times ranging from 16 to 35 min Citation[25] and navigation cutting times ranging from 13 to 40 min Citation[21]. These clinical studies also reflect the fact that computer-assisted tumor resections usually require additional time-consuming stages, including preoperative mono- or multimodality imaging and planning, placement of skin-based markers prior to surgery for intraoperative registration purposes, etc. The present experimental study investigated navigated and freehand cutting procedures that were both associated with preoperative CT imaging and a computer-assisted planning process, and showed no significant variation in planning, transfer, and cutting times.
Based on the statistical results, the senior and junior surgeons performed similarly when following the same three-step experimental protocol including computer-assisted planning and freehand and navigated bone cutting. It appears that the bone-cutting experience of the senior surgeons was not necessarily an asset during the simulated pelvic bone cutting with the aid of a navigation system. Also, the new computer-assisted 3D planning and navigation technologies developed for the study did not appear to be more advantageous, in terms of the achieved accuracy, for the younger “digital age” surgeons. However, for the freehand cutting process, most of the intralesional tumor cuts were imputed to the junior surgeons, suggesting that the surgical background of the senior surgeons was more relevant in that respect.
The results of the study discussed here concur with those of previous laboratory experiments Citation[27] that assessed quantitatively the advantages of using navigation technology to perform bone cutting. The previous study was designed independently of any surgical application and used synthetic bones of simple rectangular geometry. Both the present and previous studies were designed according to the recent concept of orthopedic quantitative surgery Citation[37], Citation[38], and are the first studies to propose a quantitative assessment of bone-cutting accuracy by using two evaluation parameters – location and flatness – that are defined in the ISO1101 standard Citation[32]. However, the present study has certain benefits. First, the bone cuts were performed on pelvic bone models instead of rectangular models, thus the experimental test bed accounted for the complex 3D geometry of the pelvic bone. Secondly, the present study quantitatively evaluated clinically relevant aspects of bone tumor cutting, including achieved surgical margins and operative times. Thirdly, the present study included a much higher number of operators (23 operators versus six in the earlier study), resulting in much more accurate estimations of the statistical mean and variance values of the bone-cutting evaluation parameters. Fourthly, both the experimental test bed and evaluation methodology used in the present study could be re-visited to investigate the potential improvements in accuracy during simulated bone tumor cutting of the pelvis when using other available pre- and intraoperative assistance technologies, including mechanical navigation, robotics and prototyped cutting guides, as well as other cutting tools such as osteotomes, chisels, drills and burrs.
This study has some limitations. First, bone cutting was simulated on a pelvic model made of solid, homogenous polyurethane foam. Cutting of the synthetic bone was easier than cutting of actual bones presenting cortical and trabecular structures. Secondly, all tumor cuts were performed using the same 0.9-mm-thick saw blade. Using a thicker and less flexible blade or correcting for blade flexure (i.e., by measuring the forces applied to the blade) could potentially decrease the flatness errors observed. Thirdly, in each instance, the navigated procedure was performed after the freehand procedure, possibly inducing a confounder because the freehand cuts introduced the complex 3D geometry of the pelvic model to the operators. Finally, all the operators were working under ideal conditions (immobilization of the synthetic bone, complete visualization and accessibility of the bony surface, etc.). Consequently, the present experiments did not account for major clinical and surgical factors that can be time-consuming intraoperatively, as well as additional sources of inaccuracy (especially for the freehand cutting process), including patient anesthesia, patient positioning and draping, surgical exposure, presence of muscles and nerves, bleeding, movements of the patient, and so on.
Conclusion
This experimental study demonstrates that cutting accuracy during simulated bone cuts of the pelvis can be significantly improved by using a navigation system integrated into a freehand process with an oscillating saw. Complementary in vivo studies could be performed to fully validate the results observed here. Once completed, the planning and navigation-guided technologies that were developed for the present study may improve bone cutting accuracy during pelvic tumor resection by providing clinically acceptable margins.
Declaration of interest: No benefits in any form have been received or will be received from a commercial party related directly or indirectly to the subject of this article. Grants from the Fonds National de la Recherche Scientifique (F.R.S.-FNRS Télévie, Belgium) (grant 7.4570.06) and Fondation Belge contre le Cancer (grant SCIE 2006/20) were used to pay the salaries of laboratory personnel and for purchase of the bone models and surgical supplies.
References
- Lewis VO. What's new in musculoskeletal oncology. J Bone Joint Surg Am 2009; 91: 1546–1556
- Donati D, El Ghoneimy A, Bertoni F, Di Bella C, Mercuri M. Surgical treatment and outcome of conventional pelvic chondrosarcoma. J Bone Joint Surg Br 2005; 87: 1527–1530
- Sherman CE, O’Connor MI, Sim FH. Survival, local recurrence, and function after pelvic limb salvage at 23 to 38 years of followup. Clin Orthop Relat Res 2012; 470(3)712–727
- Han I, Lee YM, Cho HS, Oh JH, Lee SH, Kim HS. Outcome after surgical treatment of pelvic sarcomas. Clin Orthop Surg 2010; 2(3)160–166
- Deloin X, Dumaine V, Biau D, Karoubi M, Babinet A, Tomeno B, Anract P. Pelvic chondrosarcomas: Surgical treatment options. Orthop Traumatol Surg Res 2009; 95(6)393–401
- Weber KL, Pring ME, Sim FH. Treatment and outcome of recurrent pelvic chondrosarcoma. Clin Orthop Relat Res 2002;, 397: 19–28
- Pring ME, Weber KL, Unni KK, Sim FH. Chondrosarcoma of the pelvis. A review of sixty-four cases. J Bone Joint Surg Am 2001; 83 A: 1630–1642
- Wirbel RJ, Schulte M, Maier B, Koschnik M, Mutschler WE. Chondrosarcoma of the pelvis: Oncologic and functional outcome. Sarcoma 2000; 4: 161–168
- Fuchs B, Hoekzema N, Larson DR, Inwards CY, Sim FH. Osteosarcoma of the pelvis: Outcome analysis of surgical treatment. Clin Orthop Relat Res 2009; 467: 510–518
- Delloye C, Banse X, Brichard B, Docquier PL, Cornu O. Pelvic reconstruction with a structural pelvic allograft after resection of a malignant bone tumor. J Bone Joint Surg Am 2007; 89: 579–587
- Ozaki T, Flege S, Kevric M, Lindner N, Maas R, Delling G, Schwarz R, von Hochstetter AR, Salzer-Kuntschik M, Berdel WE, et al. Osteosarcoma of the pelvis: Experience of the cooperative osteosarcoma study group. J Clin Oncol 2003; 21: 334–341
- Cartiaux O, Docquier PL, Paul L, Francq BG, Cornu OH, Delloye C, Raucent B, Dehez B, Banse X. Surgical inaccuracy of tumor resection and reconstruction within the pelvis: An experimental study. Acta Orthop 2008; 79: 695–702
- Abraham JA. Recent advances in navigation-assisted musculoskeletal tumor resection. Curr Orthop Pract 2011; 22(4)297–302
- Cartiaux O, Paul L, Docquier PL, Banse X. Computer- and robot-assisted resection and reconstruction of pelvic bone tumours - a review. Eur Musc Rev 2011; 6(2)125–130
- Fehlberg S, Eulenstein S, Lange T, Andreou D, Tunn PU. Computer-assisted pelvic tumor resection: Fields of application, limits, and perspectives. Recent Results Cancer Res 2009; 179: 169–182
- Cheong D, Letson GD. Computer-assisted navigation and musculoskeletal sarcoma surgery. Cancer Control 2011; 18(3)171–176
- Krettek C, Geerling J, Bastian L, Citak M, Rücker F, Kendoff D, Hüfner T. Computer aided tumor resection in the pelvis. Injury 2004; 35(Suppl 1)S-A79–S-A83
- Wong KC, Kumta SM, Chiu KH, Cheung KW, Leung KS, Unwin P, Wong MC. Computer assisted pelvic tumor resection and reconstruction with a custom-made prosthesis using an innovative adaptation and its validation. Comput Aided Surg 2007; 12: 225–232
- Kendoff D, Hüfner T, Citak M, Geerling J, Mössinger E, Bastian L, Krettek C. Navigated Iso-C3D-based percutaneous osteoid osteoma resection: A preliminary clinical report. Comput Aided Surg 2005; 10: 157–163
- Cho HS, Kang HG, Kim HS, Han I. Computer-assisted sacral tumor resection. A case report. J Bone Joint Surg Am 2008; 90: 1561–1566
- Wong KC, Kumta SM, Antonio GE, Tse LF. Image fusion for computer-assisted bone tumor surgery. Clin Orthop Relat Res 2008; 466: 2533–2541
- Docquier PL, Cartiaux O, Paul L, Delloye C, Banse X. Computer navigated bone cutting in the resection of a pelvic bone tumor and reconstruction with a massive bone allograft. JBJS Essential Surgical Techniques 2011; 01: 1–13
- Docquier PL, Paul L, Cartiaux O, Delloye C, Banse X. Computer-assisted resection and reconstruction of pelvic tumor sarcoma. Sarcoma 2010; 2010: 125162
- So TY, Lam YL, Mak KL. Computer-assisted navigation in bone tumor surgery: Seamless workflow model and evolution of technique. Clin Orthop Relat Res 2010; 468(11)2985–2991
- Ieguchi M, Hoshi M, Takada J, Hidaka N, Nakamura H. Navigation-assisted surgery for bone and soft tissue tumors with bony extension. Clin Orthop Relat Res 2012; 470(1)275–283
- Cho HS, Oh JH, Han I, Kim HS. Joint-preserving limb salvage surgery under navigation guidance. J Surg Oncol 2009; 100(3)227–232
- Cartiaux O, Paul L, Docquier PL, Raucent B, Dombre E, Banse X. Computer-assisted and robot-assisted technologies to improve bone-cutting accuracy when integrated with a freehand process using an oscillating saw. J Bone Joint Surg Am 2010; 92(11)2076–2082
- ASTM International. Standard F1839-08e1. Standard specification for rigid polyurethane foam for use as a standard material for testing orthopedic devices and instruments. ASTM International, West Conshohocken, PA 2008
- Paul L, Cartiaux O, Docquier PL, Banse X. Ergonomic evaluation of 3D plane positioning using a mouse and a haptic device. Int J Med Robot 2009; 5: 435–443
- Enneking WF, Dunham WK. Resection and reconstruction for primary neoplasms involving the innominate bone. J Bone Joint Surg Am 1978; 60: 731–746
- Docquier PL, Paul L, Cartiaux O, Banse X. Registration accuracy in computer-assisted pelvic surgery. Comput Aided Surg 2009; 14: 37–44
- International Organization for Standardization. Standard 1101:2004. Geometrical Product Specifications (GPS) - geometrical tolerancing - tolerances of form, orientation, location and run-out2nd edition. International Organization for Standardization, Geneva, Switzerland 2004
- Cartiaux O, Paul L, Docquier PL, Francq BG, Raucent B, Dombre E, Banse X. Accuracy in planar cutting of bones: An ISO-based evaluation. Int J Med Robot 2009; 5: 77–84
- Anselmetti B. Tolérancement - Cotation de fabrication et métrologie. Lavoisier, Paris, France 2003; 3
- Machin D, Campbell MJ, Tan SB, Tan SH. Sample Size Tables for Clinical Studies3rd edition. John Wiley & Sons, Hoboken, NJ 2011
- Brown H, Prescott R. Applied Mixed Models in Medicine1st edition. John Wiley & Sons, New York 1999
- Rivkin G, Liebergall M. Challenges of technology integration and computer-assisted surgery. J Bone Joint Surg Am 2009; 91: 13–16
- Pearle AD, Kendoff D, Musahl V. Perspectives on computer-assisted orthopaedic surgery: Movement toward quantitative orthopaedic surgery. J Bone Joint Surg Am 2009; 91(Suppl.1)7–12