Abstract
Objective: The ROBODOC system offers the theoretical advantage of providing better fit and mechanical stability of the stem in total hip arthroplasty. However, there has been no previous study on short metaphyseal-fitting stem implantation using the ROBODOC system. The aim of the present study was to compare the implant position and primary stability of short metaphyseal-fitting stems implanted by robotic milling and manual rasping in a human cadaveric femoral model.
Methods: Eight matched pairs of human cadaveric femora were randomly assigned to a robotic milling group or manual rasping group. Operative time and intraoperative femoral fractures were monitored, and radiographic evaluation of stem alignment was performed by comparison of preoperative planning and postoperative CT data. Stability testing was performed on six matched pairs of femora, excluding two specimens in which intraoperative fractures occurred.
Results: The robotic milling procedures took an average of 27 minutes longer than the manual rasping procedures (p < 0.001). The robotic milling group exhibited significantly better anteroposterior alignment and vertical seating, and also showed a significantly reduced variability in both alignment and vertical seating. No intraoperative femoral fracture was detected in the robotic milling group, whereas two femoral fractures and one femoral stem tip perforation were detected in the manual rasping group. Stability testing showed no significant difference in translational and rotational migrations between the two groups, although the robotic milling group showed a trend towards reduced variability of stability.
Conclusions: Our cadaveric study suggests that the use of the ROBODOC system for short metaphyseal-fitting stem implantation may have advantages in improving implant fit and reducing the risk of intraoperative femoral fractures without compromising primary stability.
Introduction
Metaphyseal anchorage of short-stemmed hip prostheses is a well-known concept in younger patients because it preserves bone stock and reduces stress shielding in total hip arthroplasty (THA) Citation[1]. However, though a few recent studies have reported satisfactory early clinical results Citation[2–4], it is still questioned whether short-stemmed implants exhibit good stability and osseous integration without increasing complications in comparison to conventional stems. The small surface area available for anchorage raises concerns regarding the possibility of intraoperative femoral fracture and unstable fixation.
The ROBODOC system (Curexo Technology Corporation, Fremont, CA) was developed to provide a proper cementless femoral stem fit Citation[5]. The system consists of a preoperative planning computer workstation (ORTHODOC) and a robotic arm equipped with a high-speed milling device to prepare the femoral canal. Several studies of the use of this robotic-assisted system in THA have been conducted, and favorable short-term outcomes have been obtained Citation[6–8]. However, there has been no previous report of a comparison of robotic milling and manual rasping for short metaphyseal-fitting stem implantation.
We hypothesized that robotic milling would provide a better fit and mechanical stability for short metaphyseal-fitting stems than is obtained using the manual rasping technique. To test this hypothesis, we compared the implant position and primary stability of short metaphyseal-fitting stems implanted by robotic milling or manual rasping in a human cadaveric femoral model.
Materials and methods
Cadaveric specimens and prostheses
Following institutional review board approval, 8 matched pairs of fresh frozen human cadaveric femora were obtained and visually inspected to confirm the absence of any osseous abnormalities. These 16 cadaveric specimens contained all structures distal to the femoral head. Mean age of the eight donors at time of death was 76.8 years (range: 64 to 88 years), and four were male. One femur of each pair was randomly assigned to the robotic milling group and the other to the manual rasping group. The same surgeon performed all the surgeries.
The Metha short-stem prosthesis (B. Braun Aesculap, Tuttlingen, Germany) was used in all the cadaveric femora. This prosthesis has a proximal rough titanium micro-porous coating for cementless implantation. The Metha short cementless hip stem is anchored directly within the closed ring of the femoral neck and metaphysis. The prosthesis has a modular neck adapter available in neck-shaft angles of 130°, 135° or 140°, with 7.5° of antetorsion, 7.5° of retrotorsion, or neutral torsion. The implanted position allows approximately 20° of varus-valgus variability. The implant range also allows for balancing with respect to leg length (10 mm) and antetorsion or retrotorsion of ±7.5°. One of these nine different neck adapters, the 135° neck-shaft angle adapter with neutral torsion, was used in all the femora.
Operative procedures
For surgery, each specimen was bolted to a custom-built platform that prevented any movement during the operative procedures.
Robotic-assisted THA involves a computed tomography (CT) scan, preoperative planning using the workstation, robot diagnostics and preparation, exposure and registration, and robotic milling of the femur. Because a pinless version of the ROBODOC system was used, locator pins were not necessary. Instead, a MicroScribe 3D digitizer (Immersion Corporation, San Jose, CA) was used for femoral registration. First, a CT scan was performed according to the manufacturer's protocol (1.25-mm slice thickness; 3-mm scan interval; 200-mm field of view; fewer than 200 total slices). Three-dimensional (3D) preoperative planning was then performed using the CT image data of each femur on the workstation (), and a femoral stem of the maximum size compatible with not over-reaming the endosteal cortical bone was selected. After completing the planning process, the data were transferred to the ROBODOC control computer. Before each surgical procedure, the robot was calibrated according to the manufacturer's instructions. The robotic-assisted system computer performed data registration and verification automatically. A cutter bit was then installed and the robot arm was guided in front of the bone to begin milling. Finally, a bone-motion detector was attached to the femur. Reaming was performed under constant physiological saline irrigation. After completing the milling procedure, a stem was implanted in the femoral canal in the usual manner. The osteotomy level at the femoral neck was easily determined because the neck cut line was indicated by a notch in the medial cortex of the proximal femur.
In the manual rasping group, a CT scan was also performed preoperatively. Data were transferred to a workstation and used to select the position and size of the Metha stem using the same procedure as described for the robotic milling group. The surgeon then performed progressively larger manual raspings until sufficient axial and rotational stability of the appropriately sized rasp was felt in the femoral canal. The same preoperative plan and stem were used in both groups.
We recorded the total operative time and femoral canal preparation time, as well as the set-up time for the ROBODOC system.
Radiographic evaluation of stem alignment
Differences in the radiographic measurements from the preoperative planning and postoperative CT images were compared for the two groups. Radiographic evaluation was performed by measuring the alignment and vertical seating of the implanted prostheses (). Mediolateral stem alignment in the anteroposterior view was determined by comparing the angles between the medial margin of the prosthesis and the anatomical axis of the femur. Anteroposterior stem alignment in the lateral view was determined by comparing the angles between the anterior margin of the prosthesis and the anatomical axis of the femur. Vertical seating was defined as the distance from the apex of the greater trochanter to the distal tip of the stem. To investigate inter- and intra-observer variabilities, these parameters were measured by three independent observers twice on different occasions. In addition, the presence or absence of femoral fracture was determined using postoperative CT scans for all the cadaveric specimens.
Figure 2. (a) Mediolateral stem alignment in the anteroposterior view was defined as the angle between the medial margin of the stem and the anatomical axis of the femur. (b) Anteroposterior stem alignment in the lateral view was defined as the angle between the anterior margin of the stem and the anatomical axis of the femur. (c) Vertical seating of the stem was defined as the distance from the apex of the greater trochanter to the distal tip of the stem.
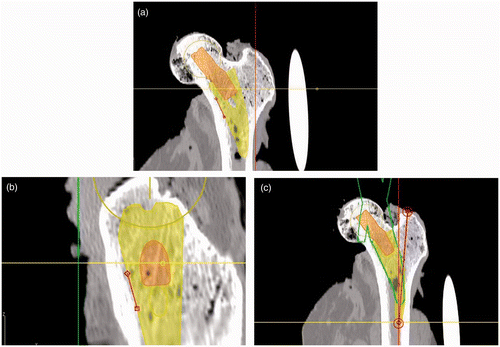
Loading procedures and stability measurements
Stability testing was performed on six matched pairs of femora, excluding two specimens that were found to have fractures during operative procedures. After implantation, all the femora were prepared as follows to apply physiological walking loads. The femora were cut 20 cm below the lesser trochanter and firmly fixed on the cylindrical mount jig of the test machine. Each specimen was positioned at an adduction angle of 16° in the frontal plane, with 9° posterior tilt in the sagittal plane, to create physiological loading, as described previously Citation[9–11]. The femora were fixed in place using a universal joint connected to the actuator of the machine, and were immobilized in the cylindrical jig by a plaster cast.
The electro-hydraulic universal test machine used (a) was manufactured specifically for stability testing at our institution, and the loading weight (maximum 5 tons) was regulated accurately by a controller. Linear variable differential transducer (LVDT) sensors (RDP Electronics, Wolverhampton, UK) were used to measure relative displacements between the implants and femora, and were connected to the implants and femora using LVDT jigs that generate bearing pressure using bolts (b). Signals recorded by the LVDT sensors were synchronized and analyzed using a National Instruments data acquisition system (DAQ system). Two LVDT sensors (LVDT1 and LVDT 2) were needed to record the displacement values, i.e., the migration of the implant in the proximal-distal direction (v) and in the mediolateral direction (h).
Figure 3. (a) The electro-hydraulic universal test machine with LVDT sensors. (b) LVDT jigs for connecting LVDT sensors to the implant and femur. (c) Trigonometric calculation of the rotating angle of the stem.
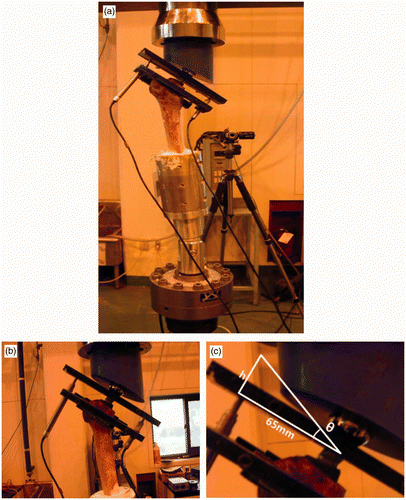
After setting up the test equipment, axial loads were applied vertically downward to standard medium (32-mm diameter) metal heads. Sinusoidal dynamic loads of frequency 1 Hz and amplitude between 58.8 N and 588 N were applied for 1200 cycles per measurement. The specimens were pre-conditioned by applying this load pattern until there was no change in the LVDT readings for at least 10 cycles. The data analysis methods used can be summarized as follows:
Initially, LVDT sensors were zeroed.
Displacement values were recorded using the two LVDT sensors.
These values were then used to calculate v and h.
Rotational angles (θ) were calculated trigonometrically (c).
The equations for these two parameters are as follows:
Statistical analysis
Non-parametric tests were used since the data were not normally distributed. The Wilcoxon signed rank test was used to detect a difference in the performance of the two different implantation techniques. Intraclass Correlation Coefficients (ICC) were used to identify degrees of inter- and intra-observer agreement. The test for equality of variances was used to compare variability between the two groups. Statistical significance was accepted for p-values of p < 0.05.
Results
Measurements of stem alignment and vertical seating in the preoperative planning and postoperative CT images are presented in . There was no significant difference in mediolateral alignment between the two groups (p = 0.382); however, the robotic milling group had a significantly better anteroposterior alignment (p = 0.046) and vertical seating (p = 0.023) than the manual rasping group. The robotic milling group also showed a significantly reduced degree of variability in all measurements, including mediolateral alignment (p = 0.001), anteroposterior alignment (p = 0.014), and vertical seating (p = 0.030), compared with the manual rasping group (). All radiological measurements showed excellent agreement for intra- and interobserver measurements (ICC > 0.8).
Figure 4. Box-and-whisker plots showing differences in implant position between the manual rasping and robotic milling groups. (a) Mediolateral alignment (ML); (b) anteroposterior alignment (AP); and (c) vertical seating (VS).
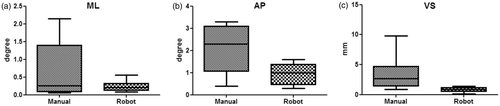
Table I. Differences in stem position between preoperative planning and postoperative CT images (median with interquartile range)
The mean operative time for the robotic milling group (63 ± 13 min) was 27 min longer (p < 0.001) than that for the manual rasping group (36 ± 10 min). The mean ROBODOC set-up time and femoral canal milling time were 9.3 min (range: 8–15 min) and 13.1 min (range: 9–22 min), respectively.
No intraoperative femoral fractures occurred in the robotic milling group, whereas in the manual rasping group two femoral neck fractures occurred during stem implantation. In addition, we also found a femoral cortical perforation at the distal stem tip by postoperative CT imaging of one specimen in the manual rasping group.
Dynamic loading procedures were performed without damaging any of the specimens (). There was no significant difference in migration in the proximal-distal direction (p = 0.437), migration in the mediolateral direction (p = 0.437), or rotational angle (p = 0.437) between the two groups, although the robotic milling group showed a trend towards reduced variability in the proximal-distal migration (p = 0.148), mediolateral migration (p = 0.096), and rotational angle (p = 0.096) compared to the manual rasping group (). All implants in both groups showed a biphasic pattern of migration with increasing load, which consisted of a relatively steep initial increase, followed by a relatively low displacement phase.
Figure 5. Box-and-whisker plots showing differences in implant motion between the manual rasping and robotic milling groups. (a) Migration in the proximal-distal direction (v); (b) migration in the mediolateral direction (h); and (c) rotational angle (θ).
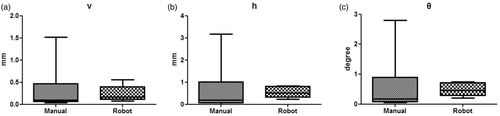
Table II. Magnitudes of migrations in the proximal-distal direction (v), migrations in the mediolateral direction (h), and rotations (θ) at the end of each load step
Discussion
The clinical performance of short metaphyseal-fitting stems remains controversial compared to that of other conventional cementless femoral components. One major concern regarding the short stem is whether it is possible to obtain proper alignment and stability of the femoral component without diaphyseal anchoring. The ROBODOC system has the theoretical advantage of providing a better fit and mechanical stability of the stem in total hip arthroplasty. However, to our knowledge, there has been no previous comparative study of robotic milling and manual rasping in short metaphyseal-fitting stem implantation. We therefore set out to address the hypothesis that use of the ROBODOC system would yield better fit and mechanical stability for short metaphyseal-fitting stems by comparing the implant position and primary stability of such stems implanted by robotic milling or manual rasping in a human cadaveric femoral model.
In the present study, the robotic milling procedures took an average of 27 min longer than the manual rasping procedures, but the robotic milling group showed significantly better anteroposterior alignment and vertical seating, and significantly reduced variability in stem alignment and vertical seating, compared with the manual rasping group. Although a large difference between the preoperative plan and postoperative CT images may not always result in poor clinical performance, the ROBODOC system does offer the possibility of an optimal preoperative planning and good execution according to the preoperative planning. Our results agree with those of studies performed with conventional cementless femoral components. Nishihara et al. Citation[7] reported that manual rasping, without preoperative planning, had disadvantages such as the potential for intraoperative femoral fracture, undersizing of the femoral stem, an unexpectedly higher vertical seating, and unexpected femoral anteversion, which in combination caused inferior implant fit and instability. Nakamura et al. Citation[12] reported that precise robotic milling enabled superior proximal stem fit and fill, which reduced proximal femur stress shielding for more than 5 years.
The reported rates of intraoperative femoral fracture following primary total hip arthroplasty using cementless femoral stems range from 2% to 20% Citation[7]. In the present study, no intraoperative femoral fracture was detected in the robotic milling group, whereas two femoral neck fractures and one femoral stem tip perforation (37.5%) were detected in the manual rasping group. This finding is consistent with those of studies performed with conventional cementless femoral components Citation[12–14]. With the use of a robotic system, the risk of intraoperative femoral fractures could be minimized. Zeh et al. Citation[15] indicated that, since the fixation of short-stemmed implants has to be achieved in the metaphysis and preparation and implantation are associated with significant peak loads, a proximal femoral fissure can be provoked intraoperatively. Other groups have reported that femoral fracture may result from poor-quality bone stock or suboptimal implantation, all of which can lead to medial cutout or cortical perforation Citation[4], Citation[16]. The comparatively high femoral fracture rate in our series might be attributable to the cadaveric specimens being from elderly donors that usually have compromised bone stock, although we did not perform bone quality assessments. Therefore, it should be emphasized that both surgical technique and careful patient selection are crucial for short-stemmed prosthesis implantation.
Westphal et al. Citation[16] indicated that the relatively higher migration magnitudes of short stem, as compared to conventional stems, implies that short-stemmed implants without additional distal stem fixation require good cancellous bone stock in the calcar region. In the present study, there was no significant difference in either the proximal-distal or mediolateral directions, or in the rotational angle, although the robotic milling group showed a trend toward reduced variability of stem stability compared to the manual rasping group. However, these results must be interpreted with caution when applied in vivo, because in vivo stability is provided by muscles and other soft tissues, and clinical loading never takes the form of a consistent loading pattern Citation[9], Citation[17], Citation[18].
Some limitations of this study should be mentioned. First, due to the nature of this cadaveric study, the number of samples available was small and, in particular, the sample size did not provide sufficient statistical power to analyze all variables. Second, the relative motion magnitudes reported cannot be directly compared to in vivo magnitudes, as explained above. Third, because only elastic motions were measured, failure loads could not be compared between the two groups and 3D motions were not measured.
In summary, the results of our cadaveric study do not support the hypothesis that primary stability of a short metaphyseal-fitting stem is improved by robotic milling, but strongly suggest that use of the ROBODOC system may have advantages in improving implant fit and reducing the risk of intraoperative femoral fractures. A further long-term follow-up in vivo study is required to clarify whether the better radiographic results and reduced fracture rates achieved with robotic milling lead to significant long-term improvements.
Declaration of interest: The authors report no declarations of interest.
References
- Learmonth ID. Conservative stems in total hip replacement. Hip Int 2009; 19: 195–200
- Morrey BF, Adams RA, Kessler M. A conservative femoral replacement for total hip arthroplasty. A prospective study. J Bone Joint Surg Br 2000; 82: 952–958
- Santori FS, Santori N. Mid-term results of a custom-made short proximal loading femoral component. J Bone Joint Surg Br 2010; 92: 1231–1237
- Kim YH, Kim JS, Park JW, Joo JH. Total hip replacement with a short metaphyseal-fitting anatomical cementless femoral component in patients aged 70 years or older. J Bone Joint Surg Br 2011; 93: 587–592
- Cleary K, Nguyen C. State of the art in surgical robotics: clinical applications and technology challenges. Comput Aided Surg 2001; 6: 312–328
- Honl M, Dierk O, Gauck C, Carrero V, Lampe F, Dries S, Quante M, Schwieger K, Hille E, Morlock MM. Comparison of robotic-assisted and manual implantation of a primary total hip replacement. A prospective study. J Bone Joint Surg Am 2003; 85: 1470–1478
- Nishihara S, Sugano N, Nishii T, Miki H, Nakamura N, Yoshikawa H. Comparison between hand rasping and robotic milling for stem implantation in cementless total hip arthroplasty. J Arthroplasty 2006; 21: 957–966
- Schulz AP, Seide K, Queitsch C, von Haugwitz A, Meiners J, Kienast B, Tarabolsi M, Kammal M, Jürgens C. Results of total hip replacement using the Robodoc surgical assistant system: Clinical outcome and evaluation of complications for 97 procedures. Int J Med Robot 2007; 3: 301–306
- Bergmann G, Deuretzbacher G, Heller M, Graichen F, Rohlmann A, Strauss J, Duda GN. Hip contact forces and gait patterns from routine activities. J Biomech 2001; 34: 859–871
- Davy DT, Kotzar GM, Brown RH, Heiple KG, Goldberg VM, Heiple KG Jr, Berilla J, Burstein AH. Telemetric force measurements across the hip after total arthroplasty. J Bone Joint Surg Am 1988; 70: 45–50
- Heller MO, Bergmann G, Kassi JP, Claes L, Haas NP, Duda GN. Determination of muscle loading at the hip joint for use in pre-clinical testing. J Biomech 2005; 38: 1155–1163
- Nakamura N, Sugano N, Nishii T, Kakimoto A, Miki H. A comparison between robotic-assisted and manual implantation of cementless total hip arthroplasty. Clin Orthop Relat Res 2010; 468: 1072–1081
- Bargar WL. Shape the implant to the patient. A rationale for the use of custom-fit cementless total hip implants. Clin Orthop Relat Res 1989; 249: 73–78
- Prymka M, Wu L, Hahne HJ, Koebke J, Hassenpflug J. The dimensional accuracy for preparation of the femoral cavity in HIP arthroplasty. A comparison between manual- and robot-assisted implantation of hip endoprosthesis stems in cadaver femurs. Arch Orthop Trauma Surg 2006; 126: 36–44
- Zeh A, Radetzki F, Diers V, Bach D, Röllinghoff M, Delank KS. Is there an increased stem migration or compromised osteointegration of the Mayo™ short-stemmed prosthesis following cerclage wiring of an intrasurgical periprosthetic fracture?. Arch Orthop Trauma Surg 2011; 131: 1717–1722
- Westphal FM, Bishop N, Honl M, Hille E, Püschel K, Morlock MM. Migration and cyclic motion of a new short-stemmed hip prosthesis–a biomechanical in vitro study. Clin Biomech (Bristol, Avon) 2006; 21: 834–840
- Bergmann G, Graichen F, Rohlmann A. Hip joint loading during walking and running, measured in two patients. J Biomech 1993; 26: 969–990
- Fottner A, Schmid M, Birkenmaier C, Mazoochian F, Plitz W, Volkmar J. Biomechanical evaluation of two types of short-stemmed hip prostheses compared to the trust plate prosthesis by three-dimensional measurement of micromotions. Clin Biomech (Bristol, Avon) 2009; 24: 429–434