Abstract
Custom cutting guides based on pre-operative imaging have been introduced for total knee arthroplasty (TKA). The aim of this prospective cohort study was to assess the reliability of repeated placement of custom cutting guides by multiple surgeons in a group of patients undergoing TKA.
Custom cutting guides (ShapeMatch®, Stryker Orthopaedics) were designed from pre-operative MRI scans. The treating surgeon placed each guide on the femur and tibia of each patient three times without pinning the block. The three-dimensional position and orientation of the guide was measured for each repetition using a computer navigation system. The surgeon was blinded to the navigation system display.
Data from 24 patients and 6 surgeons were analyzed. Intraclass correlation coefficients for all measurement parameters were in the range 0.889–0.997 (excellent), and all comparisons were statistically significant (p < 0.001). The range for femoral varus/valgus was 0.0–1.5°, with 96% of patients being within 0.5°. For femoral flexion/extension the range was 0.0–3.5° (92% within 2.5°). On the tibia, varus/valgus had a range of 0.0–1.0° (92% within 0.5°), and for slope the range was 0.0–3.5° (92% within 2.5°).
The high degree of agreement indicated that intra-surgeon variation was minimal and that the technique is reliable.
Introduction
Surgical techniques for performing total knee arthroplasty (TKA) have traditionally used manual instrumentation to guide the bone resections necessary for implanting the prosthetic components. These approaches have relied heavily on the use of internal or external alignment jigs to set the cutting block position and orientation with respect to the hip, knee and ankle centers. Accurate identification of joint centers without direct visualization can influence the alignment of these blocks with respect to the individual femoral and tibial axes, creating opportunities for single and cumulative errors in jig position, cutting planes and resulting limb alignment Citation[1]. More recently, image-based and imageless computer-assisted surgery systems have been developed to enhance the intra-operative location of anatomical landmarks, resulting in more reliable and more consistent achievement of limb alignment goals Citation[2], Citation[3].
New techniques, involving pre-operative imaging modalities such as Computed Tomography (CT) and Magnetic Resonance Imaging (MRI), have recently become available for creating customized cutting guides for use in TKA. The motivations for developing such technology include making the procedure more efficient through a reduction in the number of surgical steps, maximizing the use of anatomical landmarks and joint surfaces to position the cutting guides, increasing the accuracy of implant placement, and implementing alternative alignment techniques Citation[4–8]. Surgical goals for many customized cutting guides include the traditional neutral mechanical axis alignment Citation[7]. However, alternative designs such as custom-fit cutting guides (CCGs) target an alternative alignment based on the anatomy of the individual patient. Early clinical experience with CCGs suggests that improved patient outcomes and function in the early post-operative phase may result from kinematic limb alignment Citation[4], Citation[5], Citation[9].
One factor influencing the outcome of TKA is the ability of the surgeon to place cutting blocks on the articular surfaces in a consistent and reliable manner. Stulberg Citation[1] examined the reproducibility of manual instrumentation, assessed using a computer-navigated surgery approach, and found a significant range of variation for individual prosthetic component position and limb alignment. Despite the improvements in achieving desired limb alignment with computer-assisted surgery systems, they still involve the (subjective) assessment of anatomical landmarks, and variability and potential errors resulting from intra- and inter-observer variation in landmark identification Citation[10] may help to explain why limb alignment is not always ideal. Understanding the magnitude of potential variation in cutting block position is particularly important when custom-fit cutting guides are used, due to the absence of additional guidance references beyond the knee joint. The design of the CCGs could assist in minimizing such variation. The guides feature a saw slot that helps to set varus/valgus, flexion/extension and proximal/distal positions for the femoral and tibial components. Two articular drill holes in the guides are used to set internal/external rotation and anterior/posterior and medial/lateral alignment for both the femoral and tibial components. Assessment of such parameters in a clinical setting on osteoarthritic TKA patients has not yet been reported.
An evaluation of intra- and inter-observer variability of cutting-guide placement in a cadaver study has previously been reported Citation[11]. A prospective cohort study was initiated to assess the clinical utility of CCGs. The first part of this study was designed to assess the ability of surgeons to repeatedly position CCGs on the distal femur and proximal tibia, and to quantify the intra-surgeon reliability of this technique. The accuracy of limb alignment and clinical outcomes in patients treated using CCGs forms the second part of this prospective study and is currently on-going.
Materials and methods
The study design was conducted in accordance with the Helsinki Declaration and was approved by the local human research ethics committees at each participating institution. This study was registered in the Australian New Zealand Clinical Trials Registry (http://www.anzctr.org.au/) with registration number ACTRN12610000192088.
We identified patients scheduled for primary total knee arthroplasty using computer-assisted surgery for the treatment of osteoarthritis who were between 50 and 90 years old. Exclusion criteria included a history of reconstructive surgery or fusion of the affected joint; morbid obesity (BMI ≥ 40); the presence of metal hardware in the affected limb; and any known contra-indications for undergoing assessment by MRI. Suitable candidates who were willing to participate in the study gave their informed consent.
A total of 27 patients met the inclusion/exclusion criteria and protocol requirements for entry into the study and provided informed consent. Three completed patients were excluded from the final analysis; two because of consent violations and one due to a protocol deviation of BMI ≥ 40. A total of 24 patients were therefore included in the final data analysis, comprising 13 males and 11 females. The mean age at surgery was 65.5 years (SD 7.6; range: 55–83 years). The data from repeated trials in each subject were collated and analyzed, including calculation of the range for each parameter across the three trials.
Patients underwent a pre-operative MRI scan in accordance with a specific imaging protocol. This MRI protocol is made up of two scan sequences. First, a sagittal PD scan is acquired and used to generate a 3D model of the patient's bony knee anatomy. Following this scan, a series of T1 hip-knee-ankle scans are completed. These T1 scans are used to provide the patient's coronal limb alignment, which is then used to provide the patient's constitutional limb alignment values from the neutral limb axis. During the study, both GE and Siemens MRI scanners were used. The ShapeMatch imaging protocol is available for a number of MRI machines. Images were uploaded from the medical imaging facility to the manufacturing site (OtisMed Corporation, a subsidiary of Stryker Orthopedics, Alameda, CA) where they were used to manufacture CCGs for each patient (ShapeMatch® Cutting Guides, Stryker Orthopedics, Mahwah, NJ). A pre-operative plan including component sizing and limb alignment was created for each patient. This plan was reviewed and approved by the treating surgeon prior to manufacture of the cutting guide. The cutting guides are manufactured from Delrin® (ASTM F-1855 polyoxymethylene), with each block being intended for single use only.
Surgery and intra-operative measurements were performed by all six authors at six hospitals. Each surgeon performed between two and five procedures. Exposure was performed using either a medial parapatellar or midvastus approach, and dissection included removal of the meniscus, anterior cruciate ligament, and anterior fat pad. Any significant osteophytes deemed to affect the guide placement were also removed, as directed by the pre-operative plan. Intra-operative measurements were made using a computer navigation system (eNact Knee Navigation System and precisioN Navigation v4.0 software, Stryker Leibinger, Freiburg, Germany), which required insertion of threaded tracker pins into the femur and tibia and attachment of electronic trackers.
The navigation resection probe was placed in the cutting slot of the femoral CCG, and the treating surgeon placed the femoral CCG on the articular surface of the distal femur (). The femoral CCG was not pinned but rather held in place while the navigation system captured the positioning measurements. No measureable movement was recorded by the navigation system once the blocks were positioned. Measurement resolution as displayed by the graphical user interface of the navigation system was 0.5° and 1 mm. The surgeon was blinded to the measurements by turning off the computer screen display. After measurements were taken of the guide's position and orientation, it was removed and then repositioned on the articular surface until a total of three trials were completed. Following tibial exposure, the same procedure was then performed for repeated placement of the tibial CCG on the proximal tibial surface.
Figure 1. Technique used to measure femoral cutting guide position and orientation using the navigation system.
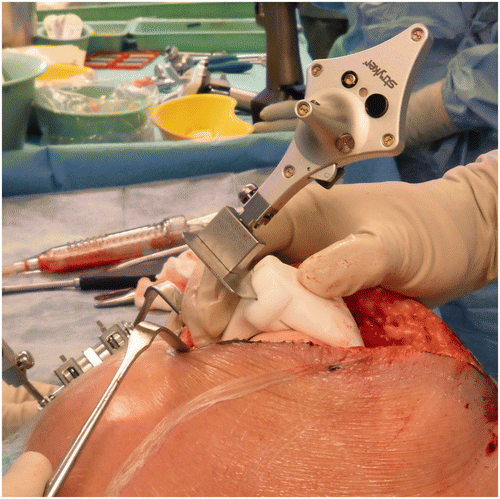
The CCGs were not used for the actual bone resection in these patients: Following completion of the study-specific measurement sequences, TKA surgery proceeded using the surgeon's preferred choice of implant and technique.
Statistical analysis was performed using SPSS for Windows version 18.0 (SPSS Inc., Chicago, IL). The intraclass correlation coefficient (ICC) describes how strongly units in the same group resemble each other. It is commonly used to assess the consistency and reproducibility of quantitative measurements made by different observers, or the repeatability of the same observers. In this study ICCs were calculated to investigate the repeatability of placement for each surgeon. ICC values greater than 0.75 represent excellent agreement, values between 0.75 and 0.4 represent good or fair agreement, and values of less than 0.4 represent poor agreement Citation[12], Citation[13].
Results
ICCs were computed for each position and orientation parameter on the femur and tibia (). All ICCs were greater than 0.9, apart from measurements for medial resection of the femur, for which the ICC was 0.889. The strongest correlation was seen in measurements for tibial rotation (0.997), closely followed by tibia varus/valgus and femoral rotation measurements with ICCs of 0.994 and 0.993, respectively. All ICCs were exceedingly high and show excellent reliability. All ICCs were statistically significant, with p-values of less than 0.001.
Table I. Intraclass correlation coefficients (ICC) for all measured variables on the femur and tibia.
The maximum and minimum change in absolute measurements between the three repetitions was calculated as the range for each parameter on both the femur and tibia ( and ). On the femur, the distribution of range for varus/valgus measurements was 0.0–1.5°, with 96% of patients being within a range of 0.5° (). The distribution of range in flexion/extension on the femur was 0.0° to 3.5°, with 92% of patients being within a range of 2.5°.
Figure 2. Frequency distribution of range for measurements for placement of the ShapeMatch cutting blocks on both the femur (a, c) and tibia (b, d). The cumulative percentage of distribution of ranges is also shown (dotted line).
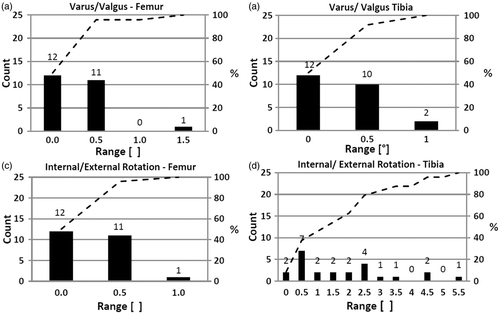
Table II. Range of absolute measurements for each variable on the femur and tibia.
Medial resection measurements on the femur showed a distribution of range of 0–3 mm, with 96% of patients being within a range of 1 mm. There was one outlier with a range of 3 mm. Similarly, the lateral resection distribution of range was 0–3 mm on the femur, with 96% of patients being within a range of 1 mm. There was one patient outlier with a range of 3 mm; this was the same patient outlier as seen in the medial resection measurements. The distribution of range for rotation on the femur was 0.0–1.0°, with 96% of patients being within a range of 0.5° ().
On the tibia, the distribution of range for varus/valgus was 0.0–1.0°, with 92% of patients being within a range of 0.5° (). The distribution of range for tibial slope was 0.0–3.5°, with 92% of patients being within a range of 2.5°. A larger variation of range was seen with this variable when compared to other variables on both the femur and tibia.
The distribution of range of medial resection measurements of the tibia was 0–3 mm, with 96% of patients being within a range of 1 mm. There was one outlier with a range of 3 mm. The distribution of range for lateral resection measurements was also 0–3 mm, with 96% of patients being within a range of 2 mm. There was one outlier with a range of 3 mm; this was the same patient outlier as seen in medial resection on the tibia.
Tibial rotation had a distribution of range of 0.0–5.5°, with 83% of patients being within a range of 3.0° (). The largest distribution of range was seen with this variable when viewing results across all the surgeons; however, this variable showed the strongest intra-surgeon correlation, representing excellent intra-surgeon reliability (ICC = 0.997).
Discussion
The results of this study show excellent correlation for all parameters measured, defined as ICCs exceeding 0.75 according to the equivalent recommendation for values of kappa Citation[12], Citation[13]. The ICC values found in a clinical setting reinforce the reproducibility findings from a previous cadaveric study Citation[11].
Of all the parameters measured in other studies of TKA component positioning and orientation, several have been identified as being more subject to surgeon variation with potential implications for clinical outcomes Citation[13–15]. These include varus/valgus orientation on the femur and tibia, internal external rotation on the femur, and internal/external rotation of the tibia. Certainly, the introduction of computer-assisted surgery systems has resulted in demonstrated improvement in performing varus/valgus cuts and overall limb alignment compared to manual instrumentation Citation[2], Citation[3], Citation[16]. Tibial rotation has proven to be particularly challenging to define in a reliable fashion based on anatomical landmarks Citation[17], and was not found to be improved by computer-assisted surgery Citation[14], Citation[16]. Large variations in tibial component orientation have been reported, with ranges of up to 30–40°. The current study showed a much smaller range, with 83% of patients being within a range of 3.0°.
Similarly, the definition of femoral rotation is less well-defined in other techniques, including reference to a variety of landmarks such as the trans-epicondylar axis, posterior femoral condylar axis, Whiteside's line, etc. A cadaver study of component position set using these different axis systems showed high variability, with only 17.3% of axes being within 5° of a reference axis Citation[13]. Our study showed a tight variation with femoral rotation measurements varying by no more than 1°. Hence, use of CCGs seems to offer potential benefits in setting positional and rotational parameters compared to both manually instrumented and computer-assisted techniques.
All surgeons performing measurements in this study had previous exposure to the CCGs during a one-day cadaver laboratory, and all were provided with guidance consistent with the surgical technique. Consistency of the training element in the introduction of this new technology was designed to reduce this parameter as a confounding influence when assessing variability. Despite this training component, all the surgeons were still very early on in their learning curve, as only a few procedures were completed in the laboratory.
There was one outlier identified in this study with a 3-mm variation in medial and lateral resection levels of the femoral cutting block. The surgeon did not note any difference in block placement compared to his other cases. The frequency and clinical relevance of outliers of this magnitude requires further investigation.
There are several limitations to the study. First, it assessed cutting guide position and orientation, not the actual position of the prosthetic component, as the cutting guides were not used to complete the bone resections. Placement of the cutting guide and measurement of its position were performed in rapid sequence, approximately 1 minute apart, which may have introduced surgeon recall of placement position; however, this was necessary to minimize disruption to the surgery. Results for three patients from the original study cohort were not included in the final analysis, as previously described. Although intra-surgeon reliability of cutting guide placement was assessed, verification of the cutting plane position of the guide with the pre-operative plan was not performed. Lastly, patient outcomes related to the specific use of this instrument system were not assessed. A prospective cohort study is currently underway to assess the accuracy of alignment and clinical outcomes using CCGs.
This study illustrates a statistically significant level of reliability for CCGs and is the first such report of reliability of these guides in arthritic patients undergoing TKA. Strengths of the study include the fact that multiple surgeons and patients were involved in the assessment, indicating that the results can be applied to the broader patient population. Surgeons performing the repeated placements were blinded to the real-time measurements from the navigation system, thereby minimizing any self-correction bias due to comparison with previous placement trials.
In conclusion, this intra-operative assessment of customized cutting guides in TKA showed a high degree of reproducibility in position and orientation despite the lack of traditional alignment guides. The consistency in cutting block placement was shown across multiple surgeons and patients. Several parameters, including femoral internal/external rotation and tibial internal/external rotation, showed a smaller deviation range than has been demonstrated for manual and computer-assisted surgery techniques. Further studies to evaluate post-operative alignment and health economic outcomes are required to understand the broader outcomes of this new technology. Clinical and patient-reported outcomes of this technology are currently being assessed by the authors in a prospective study, and a separate randomized controlled trial comparing this technology to computer-assisted navigation is ongoing. This study is important because it has effectively evaluated reproducibility of custom cutting guides in a clinical setting. To the authors’ knowledge, no other studies have yet been reported for similar products from other manufacturers. It is imperative that new technology is adequately assessed in an appropriate manner when introduced to the market.
Acknowledgements
The authors wish to thank Samantha Moulday, Ingrid Kiel-Monaghan, Regina Hansen, and Claire Leong for collection of data, and Sarah Whitehouse for statistical analysis. Timothy Barker and Natascha Millard from Stryker Australia assisted with manuscript preparation.
Declaration of interest: This study was sponsored and funded by Stryker Australia. The company assisted with study design, data verification, data analysis and manuscript preparation.
References
- Stulberg SD. How accurate is current TKR instrumentation?. Clin Orthop Relat Res 2003, 5–6: 177–184
- Sparmann M, Wolke B, Czupalla H, Banzer D, Zink A. Positioning of total knee arthroplasty with and without navigation support: A prospective, randomised study. J Bone Joint Surg (Br) 2003; 85: 830–835
- Ensini A, Catani F, Leardini A, Romagnoli M, Giannini S. Alignments and clinical results in conventional and navigated total knee arthroplasty. Clin Orthop Relat Res 2007; 457: 156–162
- Howell SM, Kuznik K, Hull ML, Siston RA. Results of an initial experience with custom-fit positioning total knee arthroplasty in a series of 48 participants. Orthopedics 2008; 31(9)857–863
- Spencer BA, Mont MA, McGrath MS, Boyd B, Mitrick MF. Initial experience with custom-fit total knee replacement: Intra-operative events and long-leg coronal alignment. Int Orthop 2009; 33(6)1571–1575
- Bonutti PM, Zywiel MG, Johnson AJ, Mont MA. The use of disposable cutting blocks and trials for primary total knee arthroplasty. Tech Knee Surg 2010; 9: 249–255
- Noble JW, Moore CA, Liu N. The value of patient-matched instrumentation in total knee arthroplasty. J Arthroplasty 2012; 27: 153–155
- Ast MP, Nam D, Haas SB. Patient-specific instrumentation for total knee arthroplasty: A review. Orthop Clin N Am 2012; 43: e17–e22
- Dossett HG, Swartz G, Estrada NA, LeFevre GW, Kwasman BG. Kinematically versus mechanically aligned total knee arthroplasty. Orthopedics 2012; 5(2)e160–e169
- Yau WP, Leung A, Liu KG, Yan CH, Wong LL, Chiu KY. Interobserver and intra-observer errors in obtaining visually selected anatomical landmarks during registration process in non-image-based navigation-assisted total knee arthroplasty. J Arthroplasty 2007; 22: 1150–1161
- Nogler M, Hozack W, Mayr E, Collopy D, Deirmengian G, Sekyra K, Intra- and inter-observer variability of jig placement in Shape Match technique of total knee arthroplasty – a cadaver study. Poster presentation at the 24th Annual Congress of the International Society of Technology in Arthroplasty (ISTA), Bruges, Belgium, September 2011
- Fleiss JL. Statistical Methods for Rates and Proportions2nd. Wiley, New York 1981
- Armitage P, Berry G. Statistical Methods in Medical Research3rd. Blackwell Science, Oxford 1994
- Siston RA, Patel JJ, Goodman SB, Delp SL, Giori NJ. The variability of femoral rotational alignment in total knee arthroplasty. J Bone Joint Surg (Am) 2005; 87(10)2276–2280
- Lützner J, Krummenauer F, Günther KP, Kirschner S. Rotational alignment of the tibial component in total knee arthroplasty is better at the medial third of tibial tuberosity than at the medial border. BMC Musculoskelet Disord 2010; 11: 57
- Nicoll D, Rowley DI. Internal rotational errors of the tibial components is a major cause of pain after total knee replacement. J Bone Joint Surg (Br) 2010; 92(9)1238–1244
- Lützner J, Krummenauer F, Wolf C, Günther KP, Kirschner S. Computer-assisted and conventional total knee replacement: A comparative, prospective, randomised study with radiological and CT evaluation. J Bone Joint Surg (Br) 2008; 90(8)1039–1044
- Page SR, Deakin AH, Payne AP, Picard F. Reliability of frames of reference used for tibial component rotation in total knee arthroplasty. Comput Aided Surg 2011; 16(2)86–92