Abstract
Objective: To compare the accuracy of postoperative mechanical alignment in computer-assisted total knee arthroplasties (CAS-TKA) related to various degrees of extra-articular tibial deformity.
Methods: We performed CAS-TKA on 30 knee models in which extra-articular proximal tibial deformities were preset to have malalignments ranging from 30° of varus to 30° of valgus. The knees were assigned to two groups, designated Group A (knees with ≤15° preoperative malalignment) and Group B (knees with >15° preoperative malalignment), and the postoperative mechanical alignment in the two groups was compared using a computer-assisted surgery (CAS) system. Resected bone pieces from the distal femurs and proximal tibias were measured with a digital Vernier caliper and the results compared with the CAS calculations to evaluate the execution accuracy of the bone resection.
Results: There was no outlier in either group when a ±3° deviation from neutral mechanical alignment was set as the acceptance criterion. Interestingly, Group B showed significantly more outliers when the acceptance criterion was a deviation of ±2° (26.67%, p = 0.0317) or ±1° (6.67%, p = 0.0007) from neutral alignment. There was no statistical difference between the groups in terms of the execution accuracy of the bone resection.
Discussion: The CAS-TKA approach provided significantly less alignment accuracy in tibia with greater preoperative frontal deformity, despite there being no outliers beyond ±3°.
Introduction
Correct limb alignment in total knee arthroplasty (TKA) is important for long-term survival [Citation1]. Postoperative limb alignment of more than 3° from the neutral mechanical axis increases the rate of early failure of the prosthesis [Citation2], and extra-articular deformity of the proximal tibia may lead to difficulties in correct implant alignment due to distorted anatomic alignment and landmarks [Citation3–5]. In severe varus deformities, manual use of instruments to make the tibia cut has been reported to increase the tibia cut in varus [Citation6]. Staged surgical procedures including tibial osteotomy can be performed, but this approach has been reported to have an increased risk of complications [Citation3–5, Citation7].
Computer-assisted TKA (CAS-TKA) is an alternative to the standard approaches to obtaining good component alignment in TKA in cases with extra-articular deformity. CAS-TKA has been shown to be effective in assisting the surgeon to obtain a more accurate overall alignment of the prosthesis in cases with extra-articular deformity of the tibia [Citation8–10]. However, the conclusions have also been controversial: many studies have reported that computer navigation did not improve the alignment in severely deformed knees [Citation11, Citation12]. From our clinical experiences in a small number of cases, we found that CAS-TKA in cases with severe extra-articular deformity of the tibia yielded good results for component alignment. We performed the present study to test the hypothesis that CAS-TKA would provide the same accuracy of postoperative limb alignment regardless of the degree of frontal knee deformity.
Materials and methods
We designed an experimental study in which 30 plastic knee models were preset with proximal tibial deformity in the frontal plane ranging from 30° of valgus to 30° of varus in relation to the lower limb mechanical axis. The knee insert bone models were prepared by making a wedge cut with an oscillating saw 1.5 cm below the most distal insertion of the patellar tendon to create proximal tibial deformities in the coronal plane, then re-joining the bone with epoxy glue (). The exact degree of deformity in each model was determined using a VectorVision CT-free navigation system (DePuy-BrainLAB, Heimstetten, Germany) after all anatomical registrations of the model were completed (). Only those models with a pure coronal deformity were included in the study. Fifteen knees were preset in varus alignment and the rest were set in valgus alignment relative to the neutral mechanical axis. The 30 knees were divided into two groups: Group A (14 knees which had mechanical valgus or varus ≤ 15°) and Group B (16 knees which had mechanical valgus or varus >15°). The angulation of the deformities is reported in . An additional knee model which had a neutral mechanical axis was used as a control.
Figure 2. Screenshot from the navigation system showed preoperative alignment of the mechanical axis (29° valgus).
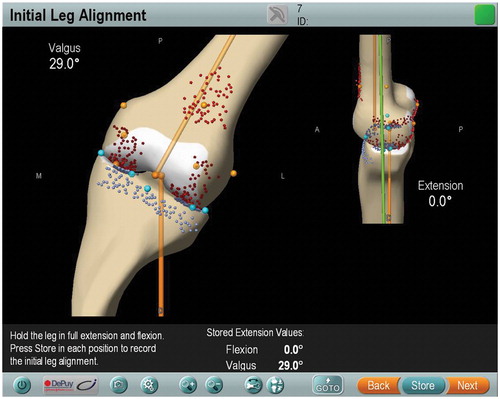
Table I. Angular deformities in all knee models.
All surgeries were performed by the senior surgeon (A.T.), who had prior experience of over 200 navigated TKAs. Before the experiment began, the preset knee insert bone models were fitted into a MITA Knee Trainer Leg workstation (Medical Models, Ltd., Bristol, UK). Using the MITA Knee workstation, the surgeon can recreate a full range of hip and knee motion, with a fixed acetabulum, which allows location of the hip center for CAS-TKA (). Every knee insert bone model had elastic medial and lateral collateral ligaments, enabling adjustment of the tension in the mediolateral plane to simulate ligament tension.
Figure 3. The knee insert bone model was fitted into a MITA Knee Trainer Leg workstation. The ball-and-socket joint of the hip in this workstation can pivot freely, allowing the surgeon to find the center of rotation of the hip. During the operation, the knee can move in the sagittal plane until full flexion or extension is attained.
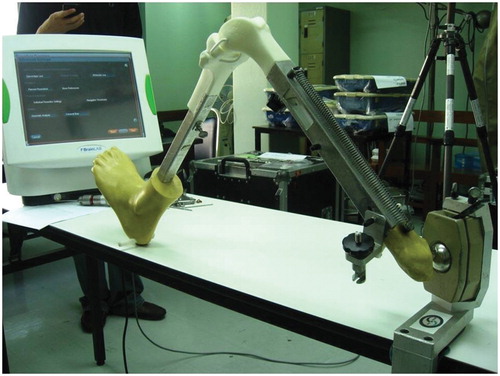
Reference arrays for the VectorVision navigation system were attached to the femur and tibia bone. The legs were pivoted around the hip to register the hip center, then surface registrations were performed by pointing or morphing the pointer according to the manufacturer’s guide. The navigation system was then used to register the anatomical data in order to adapt and digitize the 3D specific model for each sample. At this point, the navigation system determined the preoperative limb alignment (). The proximal-tibia-cut-first protocol was used in all knees. The navigation system determined the orientation of the extramedullary tibial cutting guide (in terms of varus, valgus, posterior slope and depth). We inserted the navigation system guidance array into the slot of the tibial cutting guide to enable the navigation system to trace the orientation of the guide. The position of the guide was adjusted until it was perfectly aligned with the suggested orientation in all directions, then it was fixed to the bone model with pins. The guide center was set at the middle of the tibial tubercle, and the proximal tibial bone cut was then performed using the 1.19-mm oscillating saw (DePuy, Warsaw, IN) through the tibial cutting slot. We used the same technique for the femoral bone cut. All remaining TKA steps were then performed until the steps of trial femoral, and tibial implant and polyethylene insertion were completed. The same TKA design was used in all samples (PFC Sigma Total Knee Replacement System, DePuy, Warsaw, IN). Finally, the postoperative limb alignment was determined by the computer navigation system with the knee in full extension.
All values of bone thickness according to the CAS planning for resection of the distal femur and proximal tibia were recorded. For all knees, the resected distal femur and resected proximal tibia were measured by an independent observer (S.T.) using a digital Vernier caliper. The measured values from knees operated on using the two methods were then compared.
Statistical analysis was performed using GraphPad Prism for Windows, version 5.01.336 (GraphPad Software, Inc., La Jolla, CA). The mean, standard deviation and range were used for descriptive statistics. The relationship between the degree of deformity and postoperative alignment was analyzed using the Pearson correlation coefficient. Categorical data were compared using Fisher’s exact test, with a p-value of less than 0.05 being considered significant.
Results
The control knee model had 0° of mechanical axis deviation in both pre- and postoperative measurements, with the same value for the resected distal femur and tibia being measured using both CAS and the caliper. For the 30 test knees, the mean preoperative mechanical axis was 16.63° (SD = 8.61°) and the mean postoperative mechanical axis was 1.12° (SD = 0.73°). The mean preoperative limb alignment was 9.27° (SD = 3.96°; range: 4–15°) for Group A and 24° (SD = 4.65°; range: 17–30°) for Group B. The mean postoperative limb alignment was 0.67° (SD = 0.45°, range: 0–1.5°) for Group A and 1.57° (SD = 0.68°; range: 0.5–3°) for Group B. There was a significant different in the postoperative limb alignment of the two groups (p = 0.0004).
There were positive correlations between the degree of severity of preoperative knee deformity and the degree of deviation from the target postoperative neutral mechanical axis regardless of preoperative valgus alignment (r = 0.65) or varus alignment (r = 0.59), as shown in .
Figure 4. Correlation between the preoperative mechanical axis and the postoperative mechanical axis deviation from neutral in both valgus alignment (a) and varus alignment (b).
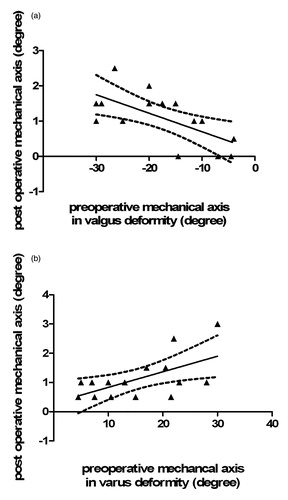
Regarding outliers, no knee in either group had a postoperative alignment beyond the range of ±3° deviation from the neutral mechanical axis. When the range of accepted outliers was narrowed to ±2°, there were four outliers in Group B (26.67%) but none in Group A ( p = 0.0317). When the acceptance range was further narrowed to ±1° deviation from the neutral mechanical axis, there was one outlier knee in Group A (6.7%) and 12 outliers in Group B (66.67%), which was a significant difference (p = 0.0007) (). For all the knees, there was no statistical difference between the CAS measurement and the digital Vernier caliper measurement of the resected bones from the distal femur and proximal tibia, as shown in .
Figure 5. Numbers of outliers were compared for Groups A and B with criteria ranging from ±1° deviation to ±3° deviation from the neutral mechanical axis.
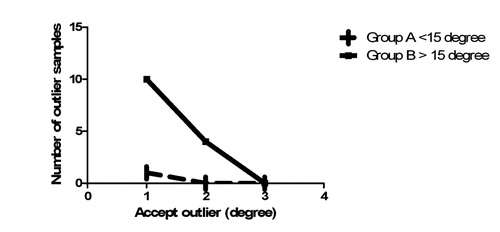
Table II. Mean difference and standard deviation of resected bone measurements using computer navigation and manual measurement with a digital Vernier caliper.
In the current study, we accepted the difference between the computer and manual measurement thickness at 1 mm; there was no significant correlation between the difference in bone cut thickness and the preoperative mechanical axis on either the femoral or tibial side ().
Figure 6. (a) The preoperative mechanical axis and differences in bone cut thickness on the femoral side show no significant correlation (r = 0.27, p = 0.14). (b) There is also no significant correlation on the tibial side at either the high or low side of the bone cuts (high side r = 0.19, p = 0.29; low side r = 0.1181, p = 0.53).
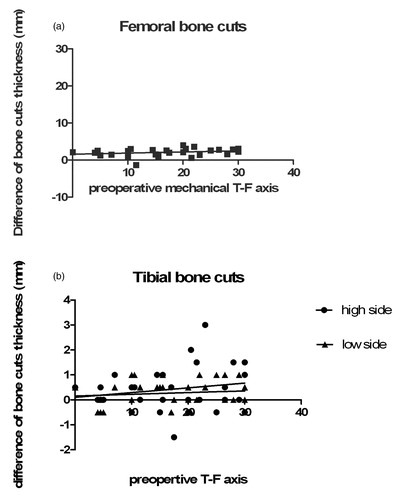
Discussion
Malalignment of greater than 3° after TKA results in higher failure rates [Citation2, Citation13, Citation14]. Computer-assisted TKA has been reported to decrease the range of outliers and improve the accuracy of the lower limb mechanical axis [Citation15, Citation16]. However, some authors have reported no relative superiority in the alignment achieved using computer navigation as compared to the conventional manual technique [Citation11, Citation12, Citation17, Citation18].
In our study, no knee had greater than 3° deviation from the neutral mechanical axis postoperatively, regardless of the severity of the preoperative deformity. This finding is in agreement with those of many other studies focusing on the accuracy of CAS-TKA [Citation3, Citation19, Citation20]. However, the variation of the postoperative mechanical axis increased significantly in Group B, which comprised knees which had more preoperative deformity. In addition, this correlation was similar for both valgus- and varus-deformed knees.
As no difference was found in the comparison of measurements of resected bones obtained using the CAS system and the digital caliper, we assumed that there was no bone cut error related to the cutting guide fixation as reported by Biant et al. [Citation21]. Thus, the correlation by which more variation of the postoperative limb axis occurred in knees which had more preoperative deformity may relate to the change in the mediolateral plane of the registered point for the center of the proximal tibia. This served to remind the surgeons that when knees were more deformed, there was a greater tendency for deviation of the postoperative limb axis away from the planned target.
A weakness of this study was that there was limited deformity only on the tibia, due to the inevitable damage to the simulated soft tissue tension construction on the preset deformed femur. Additionally, deformities were located only on the proximal tibia due to the limited length of the tibial bone model. However, our simulated knee deformity can be corrected intra-articularly more easily than femoral deformity [Citation22], and this may be of benefit in varus osteoarthritic knees, which are the most common type of deformity.
Our results showed that use of CAS-TKA in the presence of extra-articular tibial deformities can yield good results in an experimental setting with sawbones. However, application of these results to real patients requires caution. Bone resection in a real setting must take into account many factors, such as gap balancing, soft tissue tension, bone defects, and injury to the collateral ligament insertion. Wang and Wang [Citation23] stated that intra-articular resection without osteotomy was successful for the patient with an average of 20° coronal-plane deformity of the tibia. In addition, they reported that all cases require extensive medial soft tissue release and a posterior stabilized prosthesis. One-stage correction of extra-articular tibial deformity by intra-articular bone resection and TKA cannot be performed in all cases, and careful preoperative planning is mandatory for the success of the operation. Our study only points out that a computer navigation system which uses the center of the hip, knee and ankle as a reference to calculate the mechanical axis could provide high accuracy and reliability for bone resection, even in cases with very severe extra-articular deformity of the knee.
Conclusion
We concluded that computer-assisted TKA provided less alignment accuracy in cases with greater preoperative frontal tibial deformity, despite there being no outliers beyond ± 3°.
Declaration of interest
The authors report no declarations of interest.
References
- Jeffery RS, Morris RW, Denham RA. 1991. Coronal alignment after total knee replacement. J Bone Joint Surg Br 73:709–14
- Ritter MA, Faris PM, Keating EM, Meding JB. 1994. Postoperative alignment of total knee replacement: its effect on survival. Clin Orthop Relat Res 299:153–6
- Bottros J, Klika AK, Lee HH, Polousky J, Barsoum WK. 2008. The use of navigation in total knee arthroplasty for patients with extra-articular deformity. J Arthroplasty 23:74–8
- Chauhan SK, Scott RG, Breidahl W, Beaver RJ. 2004. Computer-assisted knee arthroplasty versus a conventional jig-based technique: a randomized prospective trial. J Bone Joint Surg Br 86:372–7
- Lonner JH, Siliski JM, Lotke PA. 2000. Simultaneous femoral osteotomy and total knee arthroplasty for treatment of osteoarthritis associated with severe extra-articular deformity. J Bone Joint Surg Am 82:342–8
- Teeny SM, Krackow KA, Hungerford DS, Jones M. 1991. Primary total knee arthroplasty in patients with severe varus deformity. A comparative study. Clin Orthop Relat Res 273:19–31
- Papadopoulos EC, Pavizi J, Lai CH, Lewallen DG. 2002. Total knee arthroplasty following prior distal femoral fracture. Knee 4:267–74
- Jenny JY, Clemens U, Kohler S, Kiefer H, Konermann W, Miehlke RK. 2005. Consistency of implantation of total knee arthroplasty with a non-image based navigation system: a case control study of 235 cases compared with 235 conventionally implanted prostheses. J Arthroplasty 20:832–9
- Martin A, Wohlhanannt O, Prenn M, Oelsch C, Strempel AV. 2007. Imageless navigation for TKA increases implantation accuracy. Clin Orthop Relat Res 460:178–84
- Tigani D, Masetti G, Sabbioni G, Ayad RB, Filanti M, Fosco M. 2012. Computer-assisted surgery as indication of choice: total knee arthroplasty in case of retained hardware or extra-articular deformity. Int Orthop 36:1379–85
- Yau WP, Chiu KY, Zuo JL, Tang WM, Ng TP. 2008. Computer navigation did not improve alignment in a lower volume total knee practice. Clin Orthop Relat Res 66:935–45
- Mullaji A, Kanna R, Marawar S, Kohli A, Sharma A. 2007. Comparison of limb and component alignment using computer assisted navigation versus image intensifier guide conventional total knee arthroplasty: a prospective randomized, single surgeon study of 476 knees. J Arthroplasty 22:953–9
- Anderson KC, Buehler KC, Markel DC. 2005. Computer assisted navigation in total knee arthroplasty: comparison with conventional methods. J Arthroplasty 20:132–8
- Wallace AL, Harris ML, Walsh WR, Bruce WJ. 1998. Intraoperative assessment of tibiofemoral contact stresses in total knee arthroplasty. J Arthroplasty 13:923–7
- Mason JB, Fehring TK, Estok R, Banel D, Fahrbach K. 2007. Meta-analysis of alignment outcomes in computer-assisted total knee arthroplasty surgery. J Arthroplasty 22:1097–1106
- Victor J, Hoste D. 2004. Image-based computer-assisted total knee arthroplasty leads to lower variability in coronal alignment. Clin Orthop Relat Res 428:131–9
- Takasaki M, Matsuda S, Fugakawa S, Mitsuyasu H, Miura H, Iwamoto Y. 2010. Accuracy of image free navigation for severely deformed knees. Knee Surg Sports Traumatol Arthrosc 18:763–8
- Kim YH, Kim JS, Choi Y, Kwon OR. 2009. Computer-assisted surgical navigation does not improve the alignment and orientation of the components in total knee arthroplasty. J Bone Joint Surg Br 91:14–9
- Daniel HV, Abelardo SV, Manuel ASG, Alfonso NF. 2010. Computer assistance increases precision of component placement in total knee arthroplasty with articular deformity. Clin Orthop Relat Res 468:1237–41
- Klein GR, Austin MS, Smith EB, Hozack WJ. 2006. Total knee arthroplasty using computer-assisted navigation in patients with deformities of the femur and tibia. J Arthroplasty 21:284–8
- Biant LC, Yeoh K, Walker PM, Bruce WJM, Walsh WR. 2008. The accuracy of bone resections made during computer navigated total knee replacement. Do we resect what the computer plans we resect? The Knee 15:238–41
- Wolff AM, Hungerford DS, Pepe CL. 1991. The effect of extraarticular varus and valgus deformity on total knee arthroplasty. Clin Orthop Relat Res 271:35–51
- Wang JW, Wang CJ. 2002. Total knee arthroplasty for arthritis of the knee with extra-articular deformity. J Bone Joint Surg Am 84:1769–74