Abstract
Background: Within the domain of craniomaxillofacial surgery, orthognathic surgery is a special field dedicated to the correction of dentofacial anomalies resulting from skeletal malocclusion. Generally, in such cases, an interdisciplinary orthodontic and surgical treatment approach is required. After initial orthodontic alignment of the dental arches, skeletal discrepancies of the jaws can be corrected by distinct surgical strategies and procedures in order to achieve correct occlusal relations, as well as facial balance and harmony within individualized treatment concepts. To transfer the preoperative surgical planning and reposition the mobilized dental arches with optimal occlusal relations, surgical splints are typically used. For this purpose, different strategies have been described which use one or more splints. Traditionally, these splints are manufactured by a dental technician based on patient-specific dental casts; however, computer-assisted technologies have gained increasing importance with respect to preoperative planning and its subsequent surgical transfer.
Methods: In a pilot study of 10 patients undergoing orthognathic corrections by a one-splint strategy, two final occlusal splints were produced for each patient and compared with respect to their clinical usability. One splint was manufactured in the traditional way by a dental technician according to the preoperative surgical planning. After performing a CBCT scan of the patient’s dental casts, a second splint was designed virtually by an engineer and surgeon working together, according to the desired final occlusion. For this purpose, RapidSplint®, a custom-made software platform, was used. After post-processing and conversion of the datasets into .stl files, the splints were fabricated by the PolyJet procedure using photo polymerization. During surgery, both splints were inserted after mobilization of the dental arches then compared with respect to their clinical usability according to the occlusal fitting.
Results: Using the workflow described above, virtual splints could be designed and manufactured for all patients in this pilot study. Eight of 10 virtual splints could be used clinically to achieve and maintain final occlusion after orthognathic surgery. In two cases virtual splints were not usable due to insufficient occlusal fitting, and even two of the traditional splints were not clinically usable. In five patients where both types of splints were available, their occlusal fitting was assessed as being equivalent, and in one case the virtual splint showed even better occlusal fitting than the traditional splint. In one case where no traditional splint was available, the virtual splint proved to be helpful in achieving the final occlusion.
Conclusions: In this pilot study it was demonstrated that clinically usable splints for orthognathic surgery can be produced by computer-assisted technology. Virtual splint design was realized by RapidSplint®, an in-house software platform which might contribute in future to shorten preoperative workflows for the production of orthognathic surgical splints.
Introduction
Computer-assisted surgery has gained increasing importance in the field of craniomaxillofacial surgery, as the technologies offer improved possibilities for preoperative planning, surgical transfer and quality control of the procedures [Citation1, Citation2]. Within the special field of orthognathic surgery, preoperative model surgery and the subsequent manufacture of one or more surgical splints for the transfer of the surgical planning has been the gold standard until now [Citation3]. In 2003, Gateno and co-workers pointed out that precise surgical splints could be produced by computer-assisted technologies [Citation4], and in 2008 Metzger et al. described a detailed preoperative workflow for the manufacture of surgical splints for orthognathic corrections by 3D printing devices [Citation5]. In fact, there are already a variety of commercially available solutions which are basically capable of replacing the conventional manual production of surgical splints with computer-assisted procedures [Citation2]. However, apart from the additional time and financial cost involved, there are still some drawbacks and possible pitfalls with this approach. Three-dimensional imaging and corresponding DICOM datasets are generally required for subsequent computer aided processing by appropriate software. DICOM datasets can be acquired either by conventional high-resolution multi-slice computed tomography (MSCT) or by cone-beam CT (CBCT) scanners, which were introduced for maxillofacial imaging approximately 15 years ago [Citation6]. Furthermore, although devices for computer-assisted manufacturing (3D printers) are now available, their operation and maintenance requires special skills. These factors may continue to limit the wider distribution and application of computer-assisted technologies in orthognathic surgery, but a simplified workflow for the manufacture of surgical splints might improve this situation. Accordingly, a pilot study was conducted to evaluate whether usable splints for orthognathic corrections could be produced by a computer-assisted approach. For this purpose, surgical splints were virtually designed with respect to the desired final occlusion and subsequently produced using computer-assisted technology, then compared to conventional splints that had been manufactured by a dental technician using the same target settings.
Materials and methods
Ten patients were included in this pilot series and all were operated by the same team of surgeons. Orthognathic corrections were performed using a “single-splint strategy” wherein only one final occlusal splint was required to guarantee correct occlusal relations during osteosynthesis and consolidation of the repositioned jaws. In the case of single-jaw surgery, where the final position was already determined by the antagonistic occlusal situation, the surgical splint was used predominantly for correct midline positioning of the mobilized dental arch during osteosynthesis. In the case of two-jaw surgery, reorientation of the mandibulomaxillary complex was performed according to Reyneke’s concept of “rotation of the occlusal plane” [Citation7]. After mobilization, both jaws were assembled by wires using the final splint to achieve correct occlusal relations, and the whole complex was subsequently repositioned with respect to the planned position of the maxillary incisors. Control of vertical and sagittal dimensions was performed according to intra- and extraoral landmarks as described by Kretschmer et al. [Citation8] and Gil et al. [Citation9]. Retral condylar positioning was performed manually without positioning plates. For osteosynthesis, a semi-rigid internal fixation system (Modus Oss 2.0, Medartis, Basel, Switzerland), as described by Kleier, was used.
For preoperative surgical planning photographs, dental casts as well as 3D imaging were available. An ILUMA® CBCT Scanner (IMTEC Europe, Oberursel, Germany) was used for image acquisition of patients and the corresponding dental casts. Scans were performed at standard settings for adult patients (large field of view modality [LFOV], enhanced mode, 3.8 mA, 40 seconds scanning time). The corresponding software for treatment planning, ILUMAVisionDental® (V.2.1.2.8988), was used to render virtual models for further visualization, 3D cephalometry, and planning of skeletal movements. To maintain the final occlusion, a single splint should be used. For this purpose, two final splints per patient were produced. Splints were designed with respect to a coincident midline of the upper and lower dental arches. They were also intended to act as a triangular stabilizer between the incisal edges and bilateral molars in order to balance occlusal contacts.
One of the two splints was manufactured conventionally by a dental technician according to the preoperative planning [Citation3]. The other splint was produced simultaneously by computer-assisted technology. For this purpose, dental casts were scanned by the ILUMA® device to avoid occlusal interference by dental restorations. Subsequently, these datasets were exported in DICOM format to the RapidSplint® planning software (Berliner Zentrum für Mechatronische Medizintechnik, Berlin, Germany [Citation10]), which was developed in-house. In the software-based process the digitalized dental casts were segmented using a thresholding algorithm. In the virtual planning, the upper jaw was fixed and the lower jaw repositioned relative to it. This method represents an optimized strategy with reduced parameter numbers. The desired final virtual occlusion was determined jointly by the software engineer and the surgeon. On this basis, a virtual splint maintaining the occlusal relations was generated, with the datasets subsequently being piped through the implemented Marching Cubes algorithm to create the corresponding .stl (standard triangular language format/surface tessellation language) datasets which are required for the manufacture of a model by rapid prototyping technology. The Boolean operation (virtual stamping) of the jaws and splint was performed using an extern VTK-based helper software (our own development). After the finalized datasets were approved, they were transferred to a professional service provider specializing in rapid prototyping technologies by a web-based file transfer protocol. Splints were generated by a 3D printing device (FullCure™ 720, PolyJet™) using a photopolymer which is licensed to be left intraorally for 24 hours according to the German Medizinproduktegesetz (USP Plastic Class VI) [Citation11]. The splints were delivered by the manufacturer a few days later. The cost varied slightly depending on the individual size, but was around 100 Euros per splint. Based on the sequence described above, the additional time for generating a patient-specific splint must be calculated at 2–3 hours from the moment when the dental cast became available. This time included scanning of the casts, data export, thresholding, processing, cross-checking, upload of .stl files and administration. In this pilot series, this workflow was performed during normal clinical routine by the same team, including the software engineer and surgeon.
Evaluation of both splints was done in two steps. Initially, the fit of the splints to the dental casts which had been the basis for the fabrication of the virtual and real splints was checked, then the fit of both splints was assessed according to the intraoperative situation. Simple differentiation of “clinically usable” and “not clinically usable” splints was made according to the inter-occlusal fit of the splints. For splints rated “not usable”, the corresponding cause was evaluated. All patients were informed about the pilot series and had given their written consent prior to participating. As there was no alteration of routine medical care and surgical treatment, the approval of the committee of ethics was not required.
Results
In eight of the patients two-jaw surgeries were performed, another patient underwent mandibular advancement after bilateral sagittal split osteotomy (BSSO), and LeFort I maxillary advancement was performed in the remaining patient.
Basically, in all 10 patients virtually designed splints could be generated according to the workflow presented above within a reasonable timeframe of approximately two hours per splint. In two cases, no conventional splints were available.
Clinical evaluation of the virtually designed splints was predominantly positive (). Eight of 10 virtually designed splints were assessed as being clinically usable and equivalent to conventional splints. In two cases with ideal occlusal support after repositioning of the dental arches, where splints would not be required during the postoperative consolidation period, virtual splints were produced which showed precise occlusal fitting intraoperatively. In one “non-usable” case, the design of the virtual splint was obviously erroneous. In the second case where the virtual splint was “non-usable”, the conventional splint did not fit intraoperatively either, so it is likely that dentoalveolar changes occurring between the taking of the dental casts and the surgery were responsible.
Table I. Evaluation of surgical splints with regard to clinical usability.
The preoperative workflow and the course of events during virtual splint generation and clinical evaluation will now be demonstrated by some representative patient cases.
Patient 1 ( and )
In the first patient of the series, affected by right-sided laterognathia, the surgical splint was designed for dental midline correction. An example of the operating sequence for virtual splint design is shown in . After scanning of the dental casts, DICOM datasets were exported to the RapidSplint® software, which enabled rendering and segmentation of the jaws, as well as positioning of the ideal virtual occlusion according to the desired treatment goal. This step of the workflow was performed jointly by the software engineer and surgeon. Using an implemented algorithm, the virtual splint could subsequently be designed, maintaining the position of the antagonistic occlusal surfaces in order to transfer the position of the repositioned dental arches to the real surgery. Further post-processing and conversion of the data into .stl format is required for computer-assisted splint production.
Figure 1. Virtual splint generation. The desired virtual occlusion can be determined after segmentation of scanned dental casts (upper row). A virtual surgical splint can subsequently be designed and datasets can be transformed to .stl files which are required for further computer-assisted manufacturing (lower right).
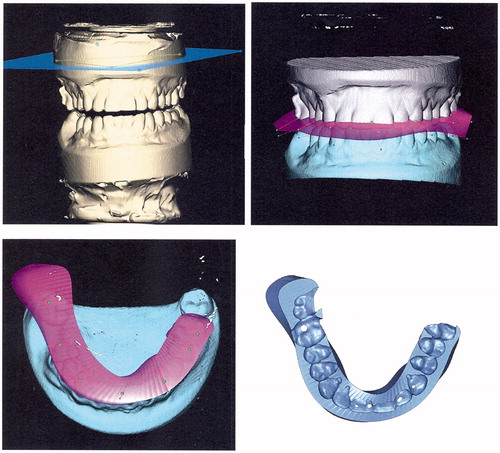
Figure 2. Top row: Prior to surgery, both splints were tested on dental casts with respect to occlusal fitting. In the first patient of the pilot series, the virtual splint (right) is still thicker than the conventional splint. Middle row: Occlusal impressions of both splints are comparable – the posterior extension of the transparent virtual splint (right) was intended to allow an assessment of the possibility of intraoperative grinding of the splint. Bottom two rows: Both splints were inserted during surgery and showed equivalent occlusal fitting with respect to the midline. Laterognathia to the right side (bottom left) was obviously improved after combined orthodontic and surgical treatment (bottom right).
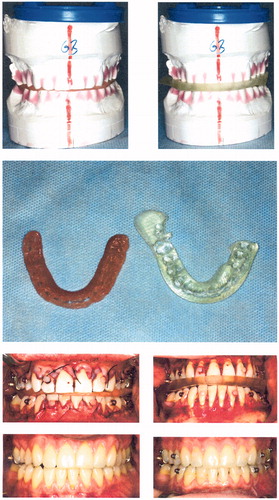
After materialization, both splints were initially checked on the dental casts. As seen in , the conventional splint (left) was obviously thinner than the virtual splint (right); however, occlusal fitting was assessed to be equal (top row). The posterior extension of the virtual splint (middle row, transparent material) was intended to enable an assessment of whether additional material could be easily reduced intraoperatively by grinding, which was not complicated. Both splints were inserted intraoperatively and showed equal occlusal fitting with respect to midline correction.
The lower images in demonstrate the clinical situation before and after orthognathic correction, showing an improved dentoalveolar midline, although a slight deviation of the lower jaw to the right must be noted which occurred during postoperative orthodontic treatment.
Patient 2 ( and )
This was a 21-year-old male patient affected by amelogenesis imperfecta, causing multiple premature dental losses as well as a severe skeletal open bite. In a combined prosthodontic-surgical treatment concept, fixed prosthodontic bridgework was inserted preoperatively. Subsequently, two-jaw surgery was performed to close the open bite, taking into account the lateral crossbite on the left side, as the patient refused any orthodontic treatment or transversal maxillary expansion.
Figure 3. Top row: Preoperative clinical situation in patient 2, a young male affected by amelogenesis imperfecta causing a severe skeletal open bite, after insertion of fixed prosthodontic bridgework. Middle row: Virtual surgery and splint design by RapidSplint: both jaws were repositioned in order to close the open bite and correct dental midlines, and a virtual splint (pink) was designed accordingly. Bottom row: Virtual splint as an .stl-file (left) and after fabrication (right) by 3D printing technology (FullCure™ 720; PolyJet™).
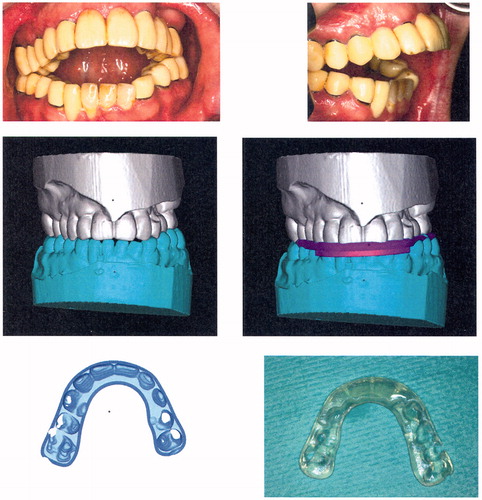
Figure 4. Upper row: The corresponding model situation for patient 2 before surgery (left) and after reorientation of the mandibulomaxillary complex. The desired occlusal relations are safeguarded by the virtually designed splint (right). Lower row: The corresponding situation during surgery. The occlusion of the mandibulomaxillary complex is safeguarded by the virtual splint (left). The final clinical situation (right) corresponds well to the virtually planned situation before surgery ().
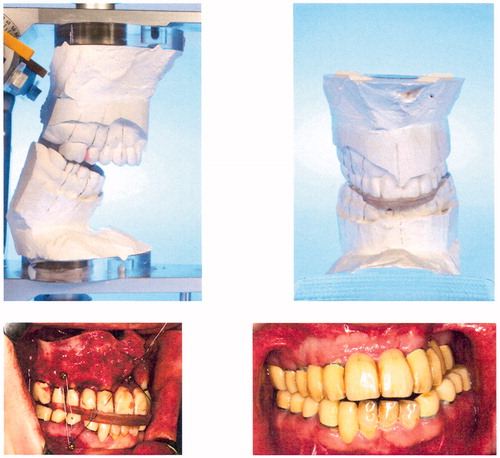
Virtual mandibulomaxillary repositioning was performed using RapidSplint®, demonstrating the desired final occlusion which is safeguarded by the virtual splint (shown in pink). The virtual splint was subsequently manufactured by 3D printing according to the virtual design and showed an excellent fit to the dental cast as well as during the intraoperative setting. The splint was used for intraoperative assembly of the mobilized jaws as a mandibulomaxillary complex, which was subsequently positioned according to the preoperative planning and stabilized by internal fixation. The postoperative clinical result is very comparable to the final virtual occlusion on which basis the virtual splint was generated. In this case, no conventional splint was fabricated. A combined prosthodontic-surgical approach and computer-assisted splint generation contributed to relevant functional and psychosocial improvement of the initial situation.
Patient 3 ( and )
This was a 20-year-old female patient with skeletal class III malocclusion due to maxillary hypoplasia and a unilateral cleft palate. Transpalatal distraction and preoperative orthodontic treatment had already been performed, and the same preoperative workflow for virtual splint generation was followed. In this case, to reduce the time required for calculation and software processing, datasets of the dental casts were reduced to occlusal surfaces which were sufficient for the positioning of the desired final occlusion. The virtual splint could also be designed with reduced datasets – a time-saving trick for possible use in future planning. Despite the minimization of the datasets, the occlusal surfaces were well reproduced and the splint was clinically usable during the two-jaw surgery. Although there is a small occlusal midline deviation, the dentofacial situation of the patient has obviously improved compared to the preoperative situation. In this case, the modification of the surgical procedure to a one-splint technique in combination with computer-assisted splint generation contributed to the realization of a contemporary patient management.
Figure 5. Top row: Preoperative situation in patient 3, a young female with skeletal class III malocclusion due to maxillary hypoplasia. Middle row: Intraoral situation before surgery and after orthodontic treatment. Bottom row: Intended virtual occlusal situation after two-jaw surgery.
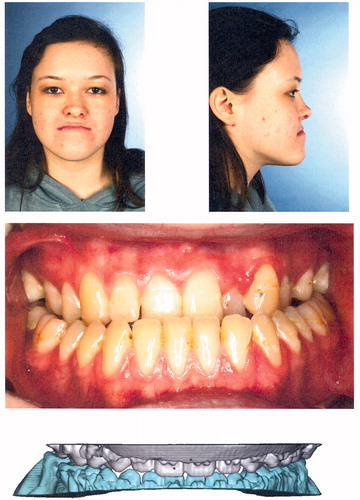
Figure 6. Top row: Virtually designed splint for patient 3 generated using reduced datasets containing only occlusal information for faster data processing. Second row: Detailed reproduction of occlusal impressions of both dental arches. Third and fourth rows: Intraoperative situation with a virtually designed splint safeguarding occlusal relations of the mandibulomaxillary complex (third row, left); final occlusion and final clinical result after termination of combined orthodontic-surgical treatment: class I occlusion, with improved facial balance and harmony.
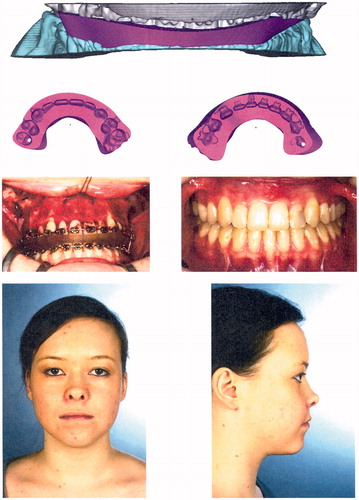
Discussion
In this pilot series, it was investigated whether surgical splints for orthognathic corrections could be produced by computer-assisted technology. In 2003 Gateno et al. were already emphasizing the possibility of surgical splint generation based on DICOM datasets, and predicted that conventional splint manufacturing by dental casts could become dispensable and be replaced by computer-assisted workflows [Citation4]. Until now, however, manual splint generation based on individual dental casts has remained the gold standard for the surgical transfer of the preoperative orthognathic planning [Citation3], probably due to the various obstacles which have inhibited the wider use of computer-assisted procedures for that purpose. Nevertheless, the number of publications about computer-assisted strategies for facilitating an exact transfer of the surgical planning has increased recently. Solutions that are already commercially available, as well as individual approaches, have been advocated for this purpose by various authors [Citation2, Citation5, Citation12–14].
Our results contribute to that context as, in this pilot series, it was demonstrated that clinically usable splints can be successfully produced for routine orthognathic surgeries using a modified workflow. Virtually designed splints were assessed as being equivalent to conventional splints and could be generated within a reasonable timeframe that would not exceed the time and effort that is normally required for the manufacture of conventional splints by a specialized technician. In a comparable study by Song and Baek, the authors also concluded that surgical splints generated by computer-assisted technologies were equivalent to conventional manufactured splints [Citation15]. The workflow described above can be further improved when 3D printing is available [Citation5, Citation14]. In that case, however, the operation and maintenance of the device can become an additional issue, which in this study was avoided by using an external provider. The economic aspects of computer-assisted splint generation were not part of this pilot series; however, Xia et al. stated in 2006 that computer-assisted technologies are cost-effective and are at least as good as established methods for craniomaxillofacial treatment planning [Citation16].
A drawback of the presented workflow is certainly the fact that scanning of dental casts is still required in order to guarantee adequate occlusal reproduction, as dental restorations still present an unsolved problem in CBCT image acquisition. Eventually, there will be a software algorithm to solve this drawback, as metal dental restorations and fixed orthodontic appliances will remain widespread for the foreseeable future. Nevertheless, this method, as well as other imaging techniques for correct occlusal reproduction (laser scanning, intraoral cameras), seems to be appropriate for providing precise occlusal datasets which can be used for surgical planning. For routine clinical application, however, these additional imaging procedures still seem to be too time-consuming [Citation17, Citation18]. The additional time required for the CBCT scanning of dental casts was around 15 minutes in this pilot series, which was considered to be acceptable.
The single-splint strategy used in this pilot series should certainly be discussed, as it seems to be a justifiable simplification compared to other surgical transfer options using two or more splints. So far, however, there are no evidence-based data indicating that one surgical approach is really superior to other approaches, as long as the outcome represents the preoperative planning and complies with the expectations of the patient and surgeon. Multiple additional factors (skeletal stability, postoperative orthodontic treatment) may further influence the final result. In single-jaw corrections where the final position of the mobilized dental arch was determined by the fixed antagonistic jaw, the surgical splint was used predominantly for midline correction. In two-jaw surgeries, the splint was used for correct assembly of the mandibulomaxillary complex according to correct occlusal relations in the first instance. Reorientation of this complex was then controlled with regard to the desired final position of the maxillary incisors by intraoperative landmark measurement, which has proved to be reliable for vertical and sagittal movements [Citation8, Citation9]. A computer-assisted two-splint strategy as described by Choi et al. [Citation14], where the first splint is used for the positioning of the mobilized maxilla with respect to the mandible, would, in fact, require the use of positioning devices. According to Costa et al. [Citation19], their significance remains open to question. In this pilot series, the complexity of the surgical procedure was further reduced by the use of a semi-rigid system for mandibular osteosynthesis which allows for condylar settling postoperatively. Consequently, there was no need for mandibular positioning devices during surgery. The modified workflow which was used in this pilot series is an attempt to reduce the time and effort required for planning and transfer of orthognathic surgical corrections. Interdisciplinary planning and computer-assisted splint generation by an engineer and surgeon, as well as an adapted surgical approach, might contribute to reducing the well-known disadvantages of classic model surgeries and the related transfer of the planning [Citation20, Citation21]. For further evidence-based statements, prospective investigations as well as long-term results are required.
Conclusion
According to the results of this pilot study, splints for orthognathic surgical corrections which were produced by computer-assisted technology proved to be equivalent for routine clinical use to conventional splints manufactured on dental casts. The presented technique seems basically appropriate for reducing the time and effort required for routine planning and realization of orthognathic corrections.
Declaration of interest
The authors report no declarations of interest.
Acknowledgements
Special thanks to Franz Hafner for his organisational skills in arranging photo documentation for this article.
References
- Bell RB. 2010. Computer planning and intraoperative navigation in cranio-maxillofacial surgery. Oral Maxillofac Surg Clin North Am 22(1):135–56
- Markiewicz MR, Bell RB. 2011. Modern concepts in computer-assisted craniomaxillofacial reconstruction. Curr Opin Otolaryngol Head Neck Surg 19(4):295–301
- Ruvo AT, Scully JR, Vandersea BA. 2008. Model surgery. In: Fonseca RJ, Marciani RD, Turvey TA, editors. Oral and Maxillofacial Surgery, Vol III: Orthognathic Surgery, Esthetic Surgery, Cleft and Craniofacial Surgery. 2nd edition. Saint Louis, MO: Saunders (Elsevier). pp 364–71
- Gateno J, Xia J, Teichgraeber JF, et al. 2003. The precision of computer-generated surgical splints. J Oral Maxillofac Surg 61(7):814–17
- Metzger MC, Hohlweg-Majert B, Schwarz U, et al. 2008. Manufacturing splints for orthognathic surgery using a three-dimensional printer. Oral Surg Oral Med Oral Pathol Oral Radiol Endod 105(2):e1–7
- Arai Y, Tammisalo E, Iwai K, et al. 1999. Development of a compact computed tomographic apparatus for dental use. Dentomaxillofac Radiol 28(4):245–8
- Reyneke JP. 2009. Rotation of the occlusal plane. In: Fonseca RJ, Marciani RD, Turvey TA, editors. Oral and Maxillofacial Surgery, Vol III: Orthognathic Surgery, Esthetic Surgery, Cleft and Craniofacial Surgery. 2nd edition. Saint Louis, MO: Saunders (Elsevier). pp 248–71
- Kretschmer WB, Zoder W, Baciut G, et al. 2009. Accuracy of maxillary positioning in bimaxillary surgery. Br J Oral Maxillofac Surg 47(6):446–9
- Gil JN, Campos FE, Claus JD, et al. 2011. Medial canthal region as an external reference point in orthognathic surgery. J Oral Maxillofac Surg 69(2):352–5
- Liu W, Holzwarth F, Adolphs N, et al. 2013. A novel concept of intelligent parametric modeling of surgical splint based on clinic empirical assessment in orthognathic surgery. Presentation at the 27th International Congress and Exhibition on Computer Assisted Radiology and Surgery (CARS 2013), Heidelberg, Germany, June 2013
- Medical Device Testing GmbH m, Bioservice B. 2003. Test report 03b072. Test item (FullCure™ 720; PolyJet™). Ochsenhausen, Germany
- Olszewski R, Villamil MB, Trevisan DG, et al. 2008. Towards an integrated system for planning and assisting maxillofacial orthognathic surgery. Comput Methods Programs Biomed 91(1):13–21
- Zinser MJ, Mischkowski RA, Sailer HF, Zoller JE. 2012. Computer-assisted orthognathic surgery: feasibility study using multiple CAD/CAM surgical splints. Oral Surg Oral Med Oral Pathol Oral Radiol 113(5):673–687
- Choi JY, Song KG, Baek SH. 2009. Virtual model surgery and wafer fabrication for orthognathic surgery. Int J Oral Maxillofac Surg 38(12):1306–10
- Song KG, Baek SH. 2009. Comparison of the accuracy of the three-dimensional virtual method and the conventional manual method for model surgery and intermediate wafer fabrication. Oral Surg Oral Med Oral Pathol Oral Radiol Endod 107(1):13–21
- Xia JJ, Phillips CV, Gateno J, et al. 2006. Cost-effectiveness analysis for computer-aided surgical simulation in complex cranio-maxillofacial surgery. J Oral Maxillofac Surg 64(12):1780–4
- Swennen GR, Mommaerts MY, Abeloos J, et al. 2009. A cone-beam CT based technique to augment the 3D virtual skull model with a detailed dental surface. Int J Oral Maxillofac Surg 38(1):48–57
- Rangel FA, Maal TJ, Berge SJ, Kuijpers-Jagtman AM. 2012. Integration of digital dental casts in cone-beam computed tomography scans. ISRN Dent 2012:949086
- Costa F, Robiony M, Toro C, et al. 2008. Condylar positioning devices for orthognathic surgery: a literature review. Oral Surg Oral Med Oral Pathol Oral Radiol Endod 106(2):179–90
- Olszewski R, Reychler H. 2004. [Limitations of orthognathic model surgery: theoretical and practical implications] [In German]. Rev Stomatol Chir Maxillofac 105(3):165–9
- Baek S-H, Kang S-J, Bell WH, Chu S, Kim H-K. 2007. Fabricating a surgical wafer splint by three-dimensional virtual model surgery. In: Bell WH, Guerruero CA, editors. Distraction Osteogenesis of the Facial Skeleton, Vol I. Hamilton, Ontario: BC Decker, Inc. pp 115–30