Abstract
Background: Various types of sternum defects are produced after the removal of thoracic tumors involving the sternum. The present study aims to elucidate the relationship between the defect patterns and their effects on thoracic respiration.
Methods: Ten sets of finite element models were produced simulating thoraces of 10 persons and termed normal models. With each of the 10 normal models, the sternum was removed in six different ways to produce new models termed defect models. Defect models were categorized into hemi-superior (H-S), hemi-inferior (H-I), hemi-whole length (H-W), bilateral-superior (B-S), bilateral-inferior (B-I), and bilateral-whole length (B-W) defect types, depending on the locations of the defects. Respiratory movement was dynamically simulated with these models. The volume change the thoraces present during respiration was measured to evaluate the effectiveness of thoracic respiration. This value – defined as ΔV – was calculated and was compared between normal and defect models.
Results: With H-W and B-W type models, ΔV dropped to around 20% of normal values. With H-S and B-S type models, ΔV dropped to around 50% of normal values. With H-I and B-I type models, ΔV presented values almost equivalent to those of normal models.
Conclusion: Effectiveness of thoracic respiration is seriously impaired when the whole length of the sternum is absent. Reconstruction of the defect is essential for these cases. However, since the upper part of the sternum is most important for effective thoracic respiration, priority should be placed on the upper part in performing reconstruction.
Introduction
The present study aims to elucidate the relationship between defect patterns of the sternum and their influences on thoracic respiration.Footnote1 The thoracic respiration is expansion and shrinkage of the thorax that results from the contracture and relaxation of respiratory muscles. This movement is an orchestrated action of the components of the thorax – the ribs, costal cartilages, and sternum. In this sense, the thoracic respiration is similar to ballet – where a team of dancers cooperate with each other, while each member plays a different role.
During respiration, the sternum plays the important role of connecting the right and left parts of the thorax. Hence, the sternum can be viewed as one of the main members of the “team”. However, there are situations, where the team has to play without this important member. In some clinical cases, partial or total resection of the sternum is needed to remove tumors invading the sternum,[Citation1–5] or to treat osteomyelitis of the sternum.[Citation6,Citation7]
In these situations, the thoracic respiration can be negatively affected because the connection of bilateral thoraces though the sternum is impaired. The impairment of the connection leads to asymmetric movement of the thorax. The asymmetric movement can hinder expansion and shrinkage of the thorax, lowering the effectiveness of the thoracic respiration.
We inquire if thoracic respiration is differently affected dependent on the locations of defects of the sternum. For instance, does the thorax present different respiratory movement between cases where the whole part of the sternum is lacking and cases, where part of the sternum remains. Furthermore, does the thoracic respiratory function differ in cases where the upper part is left and those where the lower part is left? The authors conduct the present study to clarify these questions.
Methods
Underlying principles
The present study employs computer simulation on three-dimensional dynamic models as its method. This methodology might be unfamiliar to practitioners of methods. Hence, the theoretical principles of the method are introduced first to help the readers’ understanding.
The thoracic respiration is caused by the interaction of the ribs, costal cartilages, and sternum. Although the interaction might appear complicated, it can be simplified by thinking in the following way.
First, we put our focus on a single rib. The rib has its ends constrained by the costo-vertebral and costo-sternal joints. Hence, a rib is modeled as a U-shaped object which has hinges on its bilateral ends (). In actual thoraces, multiple ribs are lined up, and the ribs are connected with each other by the fibers of the inter-costal muscles. This condition can be modeled by a column of U-shaped objects connected with each other by springs (). Columns of ribs exist on the right and left sides, and are connected with each other by the sternum. This structure is simulated by the model shown in . The connected ribs and sternum interact with each other as the springs shrink and loosen. The deviation each of the components presents with the movement can be easily evaluated by means of dynamic analysis with computer calculation. The asymmetric movement patterns that thoraces with sternum defects present can also be evaluated using this technique ().
Figure 1. Basic principles of the methods. (A) Each rib is modeled as a U-shaped object that has hinges on both ends. (B) To model bilateral sides of the thorax, the U-shaped object is placed in rows. (C) Bilateral rows are connected with a plate that simulates the sternum. (D) A model simulating a thorax that has a partial defect of the sternum.
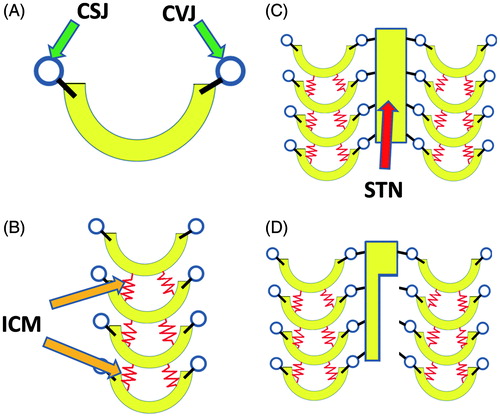
Based on the theoretical principles stated above, the authors produce three-dimensional computer models to evaluate the respiratory function of thoraces with various defects. Specific methods are explained in the following.
Production of finite element models of normal thoraces
After collecting CT data of the chests of 10 persons (6 males and 4 females) with unimpaired thoraces, the data were transformed to DICOM (Digital Imaging and Communication in Medicine) format. The thorax part of the data was extracted using graphic software (Rhinoceros 4.0, Robert McNeel & Associates, Seattle, WA).
The thoraces were transformed into dynamic models. The transformation was performed by simulating the bones and cartilages with beams and by simulating muscles with nonlinear springs (), using multi-purpose structural analysis software (ANSYS Workbench, ANSYS Co., Chicago, IL). In the process of element analysis, these components – beams and springs – are called elements. To reproduce interactions between elements of the original thorax appropriately, accurate material properties need to be allocated to each element. These material properties were decided in the following way.
Bones and cartilages: Young’s moduli (E) of bone correlate with CT density (QCT) in a relationship described by the equation E = −34.7 + 3230QCT.[Citation8] Based on this equation, Young’s modulus was calculated for each cartilage and bone of each thorax. Poisson’s ratio was set at 0.3 for both bone and cartilage.
Respiratory muscles: The external intercostal muscle and diaphragm were simulated with nonlinear springs (Mooney–Rivilin model) and were incorporated into the model. Material properties of these springs are given as a relationship between stress and strain. This relationship – shown in – was obtained from the literature.[Citation9]
Table I. Stress–strain relationship in nonlinear springs simulating respiratory muscles.
The models produced through these processes were categorized as normal type (, leftmost above).
Production of defect models
By modifying each of the 10 models belonging to the normal type, models with defects were produced. The modification was performed in the following way.
H-S (Hemi-Superior) type: Thorax models in which a hemi-sternum of the superior region is absent. These models were produced by removing the superior part of the left hemi-sternum.
H-I (hemi-inferior) type: Thorax models in which a hemi-sternum of the inferior region is absent. These models were produced by removing the inferior part of the left hemi-sternum.
H-W (hemi-whole length) type: Thorax models in which a hemi-sternum along its whole length is absent. These models were produced by removing the whole part of the left hemi-sternum.
B-S (bilateral-superior) type: Thorax models in which the bilateral-sternum of the superior region is absent. These models were produced by removing the superior part of the bilateral-sternum.
B-I (bilateral-inferior) type: Thorax models in which the bilateral-sternum of the inferior region is absent. These models were produced by removing the inferior part of the bilateral-sternum.
B-W (bilateral-whole length) type: Thorax models in which the bilateral-sternum along its whole length is absent. These models were produced by removing the whole part of the sternum.
Since all of these modifications were performed with each of the 10 normal type models, each of the above-stated six defect types has 10 models. Representative models for the six defect types are demonstrated in .
Simulation of respiration
After fixing the 1st and 12th vertebrae, loads were applied on each of the 70 thorax models (10 normal models and 60 defect models) by assuming 15% contracture of the nonlinear springs simulating respiratory muscles. Responding to these loadings, the thorax transforms. The inspiration phase was simulated as the condition, where the external inter-costal muscles and diaphragm shrank by 15%; expiration phase was simulated as the condition, where the internal inter-costal muscles shrank by 15%. Responding to these loading conditions, the thorax models transform. Representative transformation patterns of the normal and six defect types are demonstrated in .
Quantitative evaluation of respiratory function
The change in the thorax’s volume between its inspiration and expiration phases is considered to indicate the effectiveness of its thoracic respiration. The volume change was defined as ΔV. The values of ΔV were calculated from the defect and normal type models. The values of ΔV were evaluated in the following way.
Ranges and average of ΔV were calculated with the normal type models.
With the six defect type models, ranges and average of ΔV were calculated. Thereafter, the value of ΔV in a defect model was compared to the value of ΔV in the normal type model from which the defect model was produced, and the ratio was given in percentage. Thereby, the respiratory function of thoraces with defects was quantitatively accessed.
Verification of methodological validity
The following in vivo measurements were performed to verify the validity of the present study’s simulation system.
Evaluation of thorax movement on actual persons
Transformation patterns of the thorax on inspiration were examined with three volunteers. First, CT data of the thorax were collected at the resting stage of respiration. Five numbered marking points were set on the thorax. Point 1, Point 2, and Point 3 were marked at the most lateral points of the 3rd, 6th, and 10th ribs, respectively; Point 4 and Point 5 were set at the sternal notch and xiphoid process, respectively. Three-dimensional coordinates of these points were measured using graphic software (Real Intage, K.G.T. Co., Tokyo, Japan). Thereafter, CT data were again collected at the maximum inspiration stage, and the three-dimensional coordinates of the marking points were measured in the same way. By comparing the three-dimensional coordinates of the marking points before and after inspiration, the deviations the marking points present on inspiration were measured.
Simulation of inspiration on models
With each of the three volunteers, a three-dimensional model was produced. Then, inspiration was simulated by applying a contracture ratio to the nonlinear fibers connecting the ribs. The contracture ratio – set at 15% in the section “Simulation of Inspiration”) – was adjusted in this verification experiment for each sample so that Point 3’s displacement in the actual measurement matched its counterpart in simulation. The displacements of the marking points in response to the thus-defined load were calculated.
Comparison of measured and calculated data
With the five marking points, the measured and calculated data were compared. The ratios of the calculated data were expressed in percentages. With all of the five marking points of the three volunteers, the calculated displacements were in the range of 95–105% of the actually measured displacements. Thus, the validity of the modeling system was secured.
Results
The relationship between defect types and ΔV is demonstrated in . These results can be summarized as follows.
With thoraces where connection of the left and right sides of the thorax is totally lost (i.e. H-W and B-W types), the effectiveness of thoracic respiration is seriously damaged, dropping to about 20% of the normal thoraces.
The effectiveness of thoracic respiration greatly differs between thoraces that have defects in the superior region (i.e. H-S and B-S types) and those that have defects in the inferior region (H-I and B-I types). With the former, the effectiveness drops to around 50% of normal; with the latter, the effectiveness remains almost normal.
Table II. Respiratory function: ranges and averages (in the parentheses).
Discussion
Methodological considerations
Computer simulation using three-dimensional finite element models was adopted as the main experimental method in the present study. Regarding this methodological choice, some readers might question whether computer techniques are a more complicated way to evaluate respiratory function than simply measuring actual respiration volumes. It is surely true that if we could collect a certain number of clinical cases, categorize the cases according to the patients’ defect patterns, measure the patients’ respiration volumes, and analyze the data, we could clinically elucidate how sternum defect type affects the effectiveness of thoracic respiration. Furthermore, because of its directness, this method would appear easier and more convincing than the three-dimensional computer simulation we employed in the present study.
However, in most actual cases, immediate repair of the defects is conducted using artificial meshes or metal plates. Hence, postoperative measurement of the patients’ respiratory functions does not provide answers to the question “When defects are left unrepaired, to what degree does the effectiveness of thoracic respiration drop?”
However, simulation using dynamic models enables us to evaluate the effectiveness of thoracic respiration for any type of sternum defects. Therefore, we employed simulation as the present study’s method. Simulation using finite element models is an established method in the field of biomechanics, and is used for evaluation of dynamic functions of various organs, such as bones,[Citation10–14] the thorax,[Citation15] and soft tissues.[Citation16,Citation17]
Despite its proven usefulness, we still need to examine the validity of finite element simulation when we use it as the main technique of a study. Hence, we conducted the verification examination and proved the validity of the present study’s methods.
Review of the findings
The present study has two main findings. First, with thoraces where the dynamic connection of the right and left ribs is totally lost (i.e. H-W and B-W type defects), the effectiveness of thoracic respiration seriously drops. Second, although defects of the superior part of the sternum (i.e. H-S and B-S type defects) lower the effectiveness of thoracic respiration by about one half, defects of the inferior part of the sternum (i.e. H-I and B-I type defects) scarcely affect thoracic respiration. Herein, dynamic etiology of these phenomena is hypothetically explained.
First, etiology of the first finding is discussed. To analyze the behavior of the thoraces, soft tissues were made invisible from an H-W type model (). Under this condition, the movement pattern the model presents during inspiration was observed. The observation revealed an abnormal movement pattern shown in , where bilateral ribs move inward during inspiration. Since this movement of the ribs looks like a person’s arms hugging another person, we termed the abnormal movement “hugging disorder”. Because of this disorder, the volume of the thorax decreases during respiration, negatively affecting the effectiveness of thoracic respiration.
Figure 5. The abnormal transformation pattern thoraces with whole-length sternum defects present on inspiration. The ribs move inward like a person’s arms in hugging another person. This disorder pattern was named “hugging disorder”. The scale indicates the degree of movement in the posterior direction.
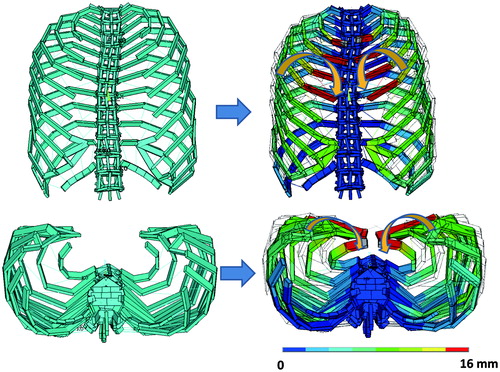
The second finding is also caused by the hugging disorder. demonstrates an inspiration pattern presented by a thorax model belonging to the B-S type. The upper part deviates inward, locally presenting with the hugging disorder. The local occurrence of hugging disorder negatively affects thoracic respiration – although the degree is less serious than for thoraces with H-W and B-W type defects. However, even if the lower part of the sternum is absent (H-I and B-I type defects), the thorax does not present hugging disorder. The absence of the hugging disorder enables the thoraces to maintain effective thoracic respiration with these types of defects.
Clinical meanings
The findings of the present study are useful in considering reconstruction plans for sternum defects.
For defects extending along the whole length of the sternum (i.e. H-W and B-W type defects), the effectiveness of thoracic respiration seriously drops. Hence, some measure should be taken to maintain the function. The functional loss is – as explained in the preceding section – caused by hugging disorder, the inward movement of the bilateral ribs. This disorder can be avoided by restoring kinetic unification of bilateral thoraces. The kinetic unification can be achieved by bridging the margins of defects using titanium plates,[Citation18,Citation19] prosthetic materials,[Citation20,Citation21] or autologeous bones.[Citation22]
In selecting the bridging site, in terms of thoracic respiration, priority should be placed on the superior part of the sternum. This is because if the kinetic unification is restored only for the inferior part of the sternum, the superior part of the thorax presents the hugging disorder like the thoraces with H-S or B-S type defects do in . Consequently, the thorax is expected to present impaired thoracic respiration. For the same reason, for thoraces with upper sternum defects, it is recommended that the defects are reconstructed to avoid local occurrence of the hugging disorder.
For thoraces with lower sternum defects, the thoracic respiration presents near-normal function. Hence, reconstruction of the defect does not bring about improvement in terms of the effectiveness of thoracic respiration. However, of course, reconstruction for mechanical protection of the heart and lung may be necessary, even if the reconstruction does not improve respiratory function.
As discussed above, the findings of the present study can be referred to in making restoration plans for sternum defects; by considering the meaning of reconstruction in terms of effectiveness of thoracic respiration, we can improve quality of life for patients in whom surgical removal of the sternum is needed.
Conclusion
By means of dynamic simulation on three-dimensional computer models of thoraces, the relationship between sternum defect patterns and their influences on the effectiveness of thoracic respiration was elucidated. The effectiveness of thoracic respiration was quantitatively evaluated referring to the volume change of the thorax between its inspiration and expiration phases. The effectiveness of thoracic respiration drops for cases with whole-length sternum defects and for cases with defects of the upper part of the sternum. For the former, the effectiveness drops to about 20% of the normal value; for the latter, the effectiveness drops to about 50%. These findings provide useful information in considering reconstruction methods for clinical cases, where removal of the sternum is needed.
Declaration of interest
The authors report no conflicts of interest. The authors alone are responsible for the content and writing of this article.
Notes
1Respiration is caused by two factors. The first factor is the volume change the thorax presents during respiration. The second factor is the positional change of the diaphragm. The present study evaluates only the former factor. Hence, all through the manuscript, we have used the term “thoracic respiration” rather than simple “respiration”.
References
- Bosc R, Lepage C, Hamou C, et al. Management of chest wall reconstruction after resection for cancer: a retrospective study of 22 consecutive patients. Ann Plast Surg 2011;67:263–268.
- Traibi A, Boulahya A, Ihrai H, et al. Giant cell tumors that originated in the sternum. Gen Thorac Cardiovasc Surg 2011;59:148–151.
- Koppert LB, van Geel AN, Lans TE, et al. Sternal resection for sarcoma, recurrent breast cancer, and radiation-induced necrosis. Ann Thorac Surg 2010;90:1102.e2–1108.e2.
- Gonfiotti A, Santini PF, Campanacci D, et al. Malignant primary chest-wall tumours: techniques of reconstruction and survival. Eur J Cardiothorac Surg 2010;38:39–45.
- Hirai S, Nobuto H, Yokota K, et al. Surgical resection and reconstruction for primary malignant sternal tumor. Ann Thorac Cardiovasc Surg 2009;15:182–185.
- Katayama Y, Minato N, Kawasaki H, Sakaguchi M. Surgical strategy for impending rupture of an infected anastomotic pseudoaneurysm of the aorta 9 years after a Bentall procedure: radical surgery involving en bloc resection of the infected sternum, pseudoaneurysm, and artificial vascular graft. Gen Thorac Cardiovasc Surg 2008;56:584–588.
- Meadows JA 3rd, Staats BA, Pairolero PC, et al. Effect of resection of the sternum and manubrium in conjunction with muscle transposition on pulmonary function. Mayo Clin Proc 1985;60:604–609.
- Kopperdahl DL, Pearlman JL, Keaveny TM. Biomechanical consequences of an isolated overload on the human vertebral body. J Orthop Res 2000;18:685–690.
- Yamada H. Muscle, skeletal muscle, fascia, tendons, tendinous tissue, calcaneal tendon, aponeuroses. In: Evans FG, ed. Strength of biological materials. Baltimore (MD): The Williams & Wilkins Company; 1970:93–105.
- Nagasao T, Miyamoto J, Hikosaka M, et al. Appropriate diameter for screws to fix the maxilla following Le Fort I osteotomy: an investigation utilizing finite element analysis. J Craniomaxillofac Surg 2007;35:227–233.
- Nagasao T, Tamada I, Miyamoto J, et al. Effectiveness of additional transmalar Kirschner wire fixation for a zygoma fracture. Plast Reconstr Surg 2007;119:1010–1019.
- Nagasao T, Miyamoto J, Nagasao M, et al. The effect of striking angle on the buckling mechanism in blowout fracture. Plast Reconstr Surg 2006;117:2373–2380.
- Nagasao T, Miyamoto J, Jin H, et al. The dynamics in implantation for patients with clefts. Cleft Palate Craniofac J 2006;43:84–91.
- Nagasao T, Nakajima T, Kimura A, et al. The dynamic role of buttress reconstruction after maxillectomy. Plast Reconstr Surg 2005;115:1328–1340.
- Nagasao T, Noguchi M, Miyamoto J, et al. Dynamic effect of the Nuss procedure on the spine in asymmetric pectus excavatum. J Thoracic Cardiovasc Surg 2010;140:1294–1299.
- Akaishi S, Akimoto M, Ogawa R, Hyakusoku H. The relationship between keloid growth pattern and stretching tension: visual analysis using the finite element method. Ann Plast Surg 2008;60:445–451.
- Mizunuma M, Yanai A, Tsutsumi S, et al. Can dog-ear formation be decreased when an S-shaped skin resection is used instead of a spindle skin resection? A three-dimensional analysis of skin surgery techniques using the finite element method. Plast Reconstr Surg 2000;106:845–848.
- Haddock NT, Weichman KE, Saadeh PB. Reconstruction of a massive thoracic defect: the use of anatomic rib-spanning plates. J Plast Reconstr Aesthet Surg 2012;65:e253–e256.
- Berthet JP, D'Annoville T, Canaud L, Marty-Ané CH. Use of the titanium vertical ribs osteosynthesis system for reconstruction of large posterolateral chest wall defect in lung cancer. Interact Cardiovasc Thorac Surg 2011;13:223–225.
- Berthet JP, Wihlm JM, Canaud L, et al. The combination of polytetrafluoroethylene mesh and titanium rib implants: an innovative process for reconstructing large full thickness chest wall defects. Eur J Cardiothorac Surg 2012;42:444–453.
- Thomas PA, Brouchet L. Prosthetic reconstruction of the chest wall. Thorac Surg Clin 2010;20:551–558.
- Chang SH, Tung KY, Hsiao HT, et al. Combined free vascularized iliac osteocutaneous flap and pedicled pectoralis major myocutaneous flap for reconstruction of anterior chest wall full-thickness defect. Ann Thorac Surg 2011;91:586–588.