Abstract
Object: The individualized Starfix® miniframe belongs to a new generation of stereotactic systems enabling high-precision electrode placement with considerably better time-efficiency in deep brain stimulation (DBS). We evaluated the usability and reliability of this novel technique in patients with idiopathic Parkinson’s disease (IPD) and compared surgical and clinical results with those obtained in a historical group in which a conventional stereotactic frame was employed. Methods: Sixty patients underwent surgery for implantation of DBS electrodes in the subthalamic nucleus. In 31 of them (group I) a conventional Zamorano-Dujovny frame was used and in 29 of them (group II) a Starfix® miniframe was used. Image fusion of preoperatively acquired 3D T1w and T2w 1.5 T MR-image series was used for the targeting procedure. Placement of the test electrodes and permanent electrodes corresponded to standard functional neurosurgery and included microelectrode recording and macrostimulation. Clinical (L-Dopa equivalent dose, United Parkinson's disease rating scale part III) and time for surgical electrode implantation were evaluated postoperatively in a 3-, 6- and 12-month follow-up. Results: Twelve months postoperatively, L-Dopa dose was significantly reduced from 685.19 to 205.88 mg/day and from 757.92 to 314.42 mg/day in groups I and II, respectively. A comparable reduction of the LED could be observed 1 year after surgery. Motor function has improved in a significant and identical manner with 59% (group I) and 61% (group II). Besides clinical effects by stimulation therapy there was a significantly reduced surgery time required for electrode implantation using the Starfix® miniframe (group I: 234.1 min, group II: 173.6 min; p < 0.001). Conclusions: Individualized miniframes such as the Starfix® miniframe allow implantation of DBS electrodes in IPD that is equally effective as conventional systems. The time efficiency achieved in surgery using of the Starfix® system helps to minimize patients' discomfort during DBS surgery.
Introduction
The region of the subthalamic nuclei (STN) is among the most favored target regions besides the globus pallidus in stimulation treatment of idiopathic Parkinson’s disease (IPD) by means of deep brain stimulation (DBS).[Citation1–3] The subject under discussion is, in addition to preoperative imaging (CT = computed tomography, MRI = magnetic resonance imaging, ventriculography), the preoperative planning of the intervention. Also, actual positioning of the electrodes is of major interest and the principle is key to success.[Citation4–7] The basis of conventional stereotactic systems and at the same its limitation is the rigid structure of the stereotactic frame. In terms of where it can be placed, the limits that are defined by the patient’s particularities (scull and neck configuration, spinal deformities as a result of camptocormia) and patient compliance, though such disadvantages can be partially compensated for through special patient positioning and an appropriate patient management. The majority of patients requiring treatment are old-aged patients suffering from multi-morbidity, who tend to have conditions such as Parkinson’s or severe dystonia that promote postural disorders, muscular dysfunctions or gibbus formations. Fixing the patient’s head into standard frame systems becomes considerably more difficult and at times even impossible.
In general, stereotactic CT or MRI scans are immediately followed by the surgical treatment in a medication-free interval, using a conventional frame system. It must be assumed here that the medication-OFF condition is responsible for the need to perform a brain imaging examination of the patient experiencing the full-blown symptoms of the disease.[Citation8] Additional movements (“motion artifacts”) that cannot willfully be suppressed by the patient are imaged in CT or MRI, making subsequent image processing and surgery planning more difficult as well. Statistics regarding this error are only of speculative value. Also, metal artifacts of the frame may appear in the field of view scans. The target region and risk structures as well as anatomical landmarks (anterior and posterior commissure, midcomissural point, red nucleus) may be at risk. Both expert practice and a correct and adequate frame assembly help to avoid such artifacts. One solution for the described conflict includes the use of individualized frames like a Starfix® miniframe. In this system, artificial landmarks (bone anchors) are placed in the tabula externa of the skull in outpatient treatment under ongoing Parkinson’s medication, which are used both as reference points for the subsequent electrode planning and as points to subsequently fix the patient-customized miniframe too. Image acquisition and processing are performed during a medication-ON interval and are therefore less stressful for the patient. Miniframes further implement all spatial coordinates and offer the chance to shorten the operative procedure using a bilateral approach.
In a recently published study, we compared a smaller patient sample which STN-DBS by means of the Starfix® system or conventional stereotactic frames.[Citation9] We found a significant reduction of surgical time with the Starfix® miniframe and comparable clinical results using both approaches. In this study, we aim at determining whether these findings can be confirmed in a larger patient sample and longer follow-up times and to obtain more in-depth information on the advantages and disadvantages of both methods. The presented retrospective analysis compares conventional frame-based to customized stereotactic frames with regard to neurosurgically and clinically relevant data. The aim was to determine the impact this type of automated frame and surgical method has on the outcome of treated patients with IPD in a larger case series than published previously.[Citation9] The following hypotheses were addressed:
Hypothesis I. Individualized Starfix® miniframes are equally reliable and practicable in functional neurosurgery, as compared to conventional stereotactic frames.
Hypothesis II. Operation time can be shortened by using Starfix® miniframes, as compared to conventional stereotactic frames.
Materials and methods
Sixty patients with IPD were examined retrospectively in a timeframe between 2002 and 2011. The patients underwent STN-DBS surgery using different surgery methods: 31 patients of group I (15 women, 16 men) were treated using the conventional frame-based stereotactic frame (Zamorano-Dujovny, ZD), whereas the Starfix® miniframe was applied in another 29 patients of group II (10 women, 19 men). Diagnostic confirmation of IPD was done by the clinical symptoms and by supplementary diagnostic tests (SIDAM, MMST, BDI, L-Dopa-test, MRI, optional DAT-Scan, 123I-IBZM SPECT). Potential contraindications were excluded. Surgery was exclusively realized by one neurosurgeon (D.W.), specialized in functional stereotactic neurosurgery.
Group I: conventional stereotactic frame (ZD)
On the day of surgery, the patient’s head was fixed in a MR-compatible ceramic head holder (ZD) under local anesthesia. The positioning of the head ring was chosen in a manner to prevent pin and other metal artifacts from being in the expected STN region. For functional and surgical planning T1-and T2-weighted MRI modalities were acquired and fused slice identically. The T2-weighted images allow an excellent visualization of the STN and neighboring anatomical landmarks (red nucleus). The T1-weighted images were used for stereotactic planning and realization of surgery. Before T1-weighted image acquisition Gd-DTPA contrast media was given using the standard dose (0.1 mmol/kg bodyweight; Magnevist, Schering, Germany) to improve details and to identify passing vessels. Any field inhomogeneities were ruled out before starting the DBS program based on MR image modalities. Entry and target points were chosen and the space coordinates were calculated automatically by a “frame-based stereotactic” software, including the values for the target device of the stereotactic system. These coordinates were the basis for the intraoperative used target arc system, being connected with the stereotactic ZD head ring. A target point simulator was used for intraoperative control. Checking the coordinates verified that the electrode passed exactly to the previously defined target point. Electrode implantation was then done bilaterally, beginning with the more symptomatic side via microelectrode recording (MER) and macrostimulation.[Citation10] Under intraoperative fluoroscopy control, the final electrodes (quadripolar platinum/iridium electrode, model 3398, Medtronic, Minneapolis, MN) were placed and the first part of surgery was finished. Then, the impulse generator (Activa, Medtronic) was implanted and connected to the simulation electrodes under general anesthesia. In contrast to the majority of all operated patients in the first seven cases, an externalization of the electrodes and external test stimulation was done to test the stimulation effect intensively.
Group II: individualized Starfix® miniframe
When applying the patient-customized miniframes, at least four bone markers were placed in the area of the frontal and the parietal bones under local anesthesia. The potential trajectory entry points were planned to be located in the center of the working and activity field, formed by the bone markers, lying close to the coronal suture.[Citation5] Hence, the positions of the bone markers were in the vertex area, so that any potential metal artifacts could neither affect the target zone, nor orientation landmarks and structures at risk close to the target during surgery. Next, CT and MR (T2- and T1-weighted with and without contrast media) were acquired to detect anatomical landmarks (red nucleus) and structures at risk (blood vessels) as described for group I. The slice-identical fusion of the respective image data and 3D electrode planning then took place, using the Voxim®-software (JoCoMed, Chemnitz, Germany). The resulting data were used to produce a virtual stereotactic platform and then sent electronically for manufacturing an individual platform by means of laser sintering. Delivery of the frame took place within 2–3 days. The temporarily released patient was re-admitted for the surgical intervention. During the operation under local anesthesia, the previously positioned bone anchors were re-exposed and the customized platform was mounted onto these anchors. After burr-hole trepanation, simultaneous MER and macrostimulation were done bilaterally. Implantation of the impulse generator was routinely performed under general anesthesia after electrode placement.
Follow-up and statistics
To compare both groups, one neurologist blinded to the groups analyzed the preoperative clinical data and the data gained after 3, 6 and 12 months postoperatively. In normal distribution, the unpaired Student's t-test was used to compare United Parkinson's disease rating scale part III (UPDRS), L-Dopa dose and the L-Dopa equivalent dose (LED) of both groups. To compare the data of groups I and II before and after surgery, the paired Student's t-test was used. For the parametric data and the non-normally distributed values in group II, the Wilcoxon signed-rank test was used. To compare ordinal parameters of the two groups, we employed the Mann–Whitney U-test. All data were expressed as the mean values ± standard deviations.
Results
Patient age and gender were similar in both groups. The IPD duration of group II (12.9 years) was longer than in the group I (11.3 years) without being significantly different. The epidemiological data (gender, age and disease duration) can be found in .
Table 1. Baseline patient data.
Neurosurgical parameters
The inspection of the neurosurgical parameters revealed significantly differing operating times for the respective procedures (; p < 0.001). The mean duration of intraoperative electrode placement in the conventional group (group I) was 234 min (range 72–370 min), whereas electrode implantation averaged 174 min in group II (range 70–326 min). Generator placement took 65 min and 45 min in groups I and II, respectively. The given times did not include the preparation for surgery. The preparation times were similar in both groups and included the placement of the stereotactic frame under local anesthesia and light sedation in the conventional group, and the marker placement under local anesthesia in group 2.
Table 2. Surgical data of electrode and generator placement.
Group I (conventional stereotaxy)
Preoperative clinical data of group I showed a UPDRS ON of 24.6 (±12.8) and UPDRS OFF of 43.0 (±15.6; ). The postoperative improvement of the fine motor capability was statistically significant: under ongoing medication and after three months at 49.9% (UPDRS ON: 12.3 ± 7.6, p = 0.01), after 6 and 12 months at 28.9% [UPDRS ON: 17.5 ± 4.3 (p = 0.01) and 17.5 ± 6.2 (p = 0.06)]. Twelve months after STN-DBS, the UPDRS OFF was at 41.8 ± 15.6 and was thus comparable with the preoperative OFF-value (). The necessary L-Dopa medication was also similar throughout the entire period (). A reduction from preoperative: 685.2 ± 301.3 to 200.1 ± 186.9 mg/day after 3 months was apparent here, i.e. by 70.8% of the initial value. The dosage adjustment 6 and 12 months after implantation was significantly lower than the initial value with 265.9 ± 211.6 and 205.9 ± 141.3 mg/day, respectively. A similar dynamic could be detected in the LED. These were reduced significantly from a preoperative dose of 1158.9 ± 515.3 to 152.5 ± 184.0 mg/day 3 months postoperatively and to 325.3 ± 185.8 mg/day and 286.8 ± 170.6 mg/day 6 and 12 months postoperatively (; ).
Figure 1. Comparison of LED in both groups showing a significant decrease of dosage in all postoperative time intervals (p ≤ 0.01**).
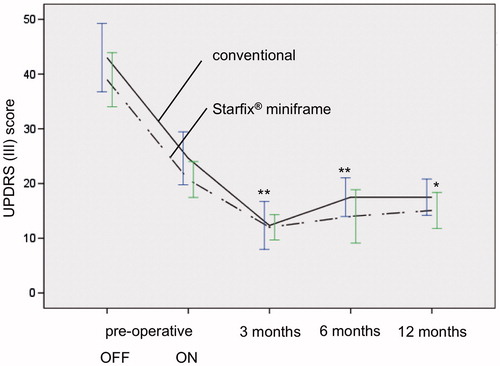
Figure 2. Pre- and postoperative UPDRS in both groups, depending on time course (preoperative, 3, 6 and 12 months after STN-DBS; p ≤ 0.01**; p ≤ 0.05*). Improvement of motor functionality is comparable in conventional stereotactic group (group I) and Starfix® miniframe group (group II).
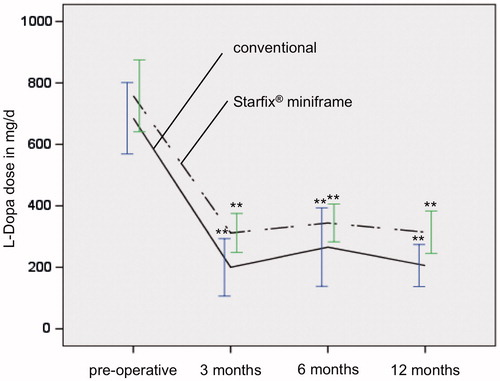
Figure 3. Reduction in L-Dopa dose in both groups is significant (p ≤ 0.01**) in the postoperative course 3, 6 and 12 months after DBS compared to initial preoperative L-Dopa dose. postop, postoperatively.
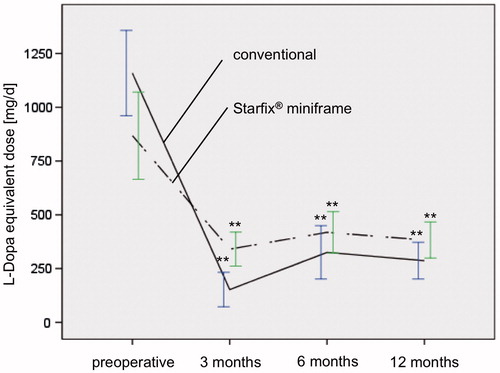
Table 3. Pre- and postoperative medication and UPDRS data showing the stimulation effect in conventional and Starfix® miniframe group during the 1 year follow-up.
Group II (Starfix® miniframe)
The results of group II were similar to group I with regard to all investigated parameters. The UPDRS ON improved from 20.7 ± 9.0 to 13.8 ± 3.9 (3 months) and 14.0 ± 6.0 (6 months; ). Clinical improvement was also significant one year (15.1 ± 8.4) after surgery (p =0.015). The pre- and postoperative UPDRS OFF were only marginally different (38.7 ± 13.3 versus 35.8 ± 9.7; ). As a result of the operation, there was a significant reduction in the L-Dopa dose from 757.9 ± 320.1 to 311.9 ± 145.5 mg/day and therefore to 46.6% of the initial value 3 months after start of the stimulation and to 344.4 ± 130.5 mg/day 6 months postoperatively, respectively, 314.4 ± 176.7 mg/day 12 months postoperatively (). The LED was significantly reduced by 39.2% (preoperative: 868.0 ± 554.9 mg/day, postoperative: 340.5 ± 180.5 mg/day) 3 months after stimulation started (). LED was reduced to 418.3 ± 203.5 mg/day 6 months after stimulation and to 383.0 ± 213.7 mg/day 1 year after surgery ().
Surgery associated complications
Neither group experienced any electrode malpositioning nor intracranial complications such as bleedings. One patient in the conventional group I had an infection in the area of the temporarily externalized electrode cable in the period under observation. Another patient experienced a ballooning of the generator pocket due to cerebral fluid collecting therein, and a third patient developed a retro-auricular wound healing disturbance in the area around the electrode extension, due to an atrophic skin condition. In group II, one patient had an infection at one of the bone anchors, another patient an infection near the generator pocket, both being treated conservatively. In a third patient, the extension cable broke and perforated.
Discussion
Both groups are similar in terms of their group size, gender distribution, patient age and disease duration. The preoperative findings regarding medication (L-Dopa dose and LED) and the UPDRS are similar, thus allowing subsequent group comparison. Both medical and clinical data gained in our present study are comparable to the data of others [Citation11–16] and to our own data in a much smaller number of cases.[Citation9] Comparability of both groups continued postoperatively: the L-Dopa dose and LED showed a significant reduction and the fine motor capability determined in UPDRS clearly improved postoperatively, confirming hypothesis I. Other groups report an UPDRS improvement of 22–43% after a 1-year lasting stimulation.[Citation11,Citation17,Citation18] With regard to medication required, Trittgemeyer et al. report a reduction in the L-Dopa dose to 71.1% and a LED reduction of 52.9% 1 year after stimulation began,[Citation17] Fehér et al. found a reduction in the L-Dopa dose to around 62% of the initial dose after an one year duration of stimulation [Citation15] and Krack et al. report a reduction of the LED to 41.4%, compared with the preoperative medication [Citation3]. Other existing studies have a large fluctuation in the clinical parameters observed in common.[Citation11–16] Similar fluctuations were observed in our study population in the postoperative course, which might be attributed to the continuous adaption of the medication and of the stimulation parameters.
The comparability of the two groups of our study was documented by both Balachandran et al. and Konrad et al. in comparing the method to conventional stereotaxy.[Citation19,Citation20] Further studies on methodological comparisons between conventional and Starfix® miniframe stereotaxy do not exist for the group dimensions stated. Independent of the clinical findings in both groups, the two methods have considerable logistical differences: for example, the imaging data in the conventional group are acquired immediately before the surgical intervention during a medication-OFF status and after the stereotactic ring has been attached. Limiting factors in this case are disease-based motion artifacts (medication-OFF) and the more challenging image acquisition in MRI because of the IPD related stiffness and the potential need of using a stereotactic frame and head coil.[Citation10,Citation21] Surgery start is delayed by the time required to perform image processing and target point calculation. The surgical procedure in the Starfix® miniframe group differs greatly: The outpatient presentation includes the preoperative acquisition of the required imaging after the bone anchors have been set. The ongoing medication of the patient makes it much easier to limit Parkinson-related motion artifacts. MRI imaging is eased, since no stereotactic frame is needed at this time. Image processing and target point calculation are completed with the dispatch of the planning coordinates for the production of the patient-customized Starfix® miniframe. The algorithm allows for a logistic decoupling of image acquisition, surgery planning and surgery execution [Citation22,Citation23]
The most striking result using the Starfix® miniframe system was the shortening of surgery time- required for electrode implantation. The reasons for the time and thus cost-saving electrode placement are primarily the use of a pre-manufactured stereotactic platform,[Citation1] which dispenses with the need for adjusting spatial dimensions and axes, and the option of carrying out simultaneous microrecordings and macrostimulation and the positioning of the final electrodes.[Citation2] This shortening in pre- and intraoperative time also results in a shortened medication-OFF interval for the patient, allowing a longer lasting cooperation during electrode placement or test stimulation. This advantage additionally contributes to minimizing surgery duration and optimizing surgical procedure, confirming hypothesis II.
Nevertheless, the accuracy of any “static” image guided devices decreases with the time of surgery secondary to the intraoperative brain shift as a result of (a) loss of cerebrospinal fluid (CSF), (b) gravity, (c) anesthesiology procedures and (d) brain-immanent characteristics. Shift studies proved that long lasting procedures can dispose progressive CSF loss via burr hole trepanation and brain shift associated inaccuracies even in basal parts of the brain.[Citation24] Consequently, all factors causing brain shift associated inaccuracies can be prevented effectively by minimizing surgical time.[Citation25,Citation26] Not having to perform image acquisition, image processing and surgery planning on the actual day of surgery has proven to be advantageous compared with conventional stereotactic intervention.
The temporal difference in placing the generator is independent of the stereotactic method chosen and is more likely to be a result of an external test stimulation carried out in the interim period in seven patients from the conventional group. Because of the increasing risk of avoidable infections during this test interval, no further patient was stimulated externally and this part of functional neurosurgery was stopped generally. The neurosurgical complications observed in both groups were similar to those cited by other authors.[Citation11–15] The time saving gained intraoperatively has to be weighed against the additional costs of the Starfix® miniframe system. These extra costs are accounted for in the German remuneration system. However, the question would be of interest as to how far the reduction of costs - including savings in personnel expenditure associated with the shortened surgery time may contribute to absorbing the extra costs connected with the use of miniframes.
Our study has several limitations that need to be addressed: First, potential effects of a learning curve of the procedure cannot be excluded in our study, especially since all patients underwent electrode implantation by a single neurosurgeon. Second, a temporary increase was observed for UPDRS-ON score, L-dopa dose and LED in both techniques especially 6 months postoperatively. Third, though UPDRS-ON scores decreased significantly in both groups in the postoperative course, the UPDRS-OFF scores 12 months postsurgically were hardly influenced by STN-DBS. These results underline the neuroplasticity related to IPD. It may also be argued that STN-DBS may have neuroprotective effects but not necessarily neuroregenerative effects, i.e. means of a partial recovery of cell populations in the substantia nigra. These findings will need to be subject of further investigations.
Conclusions
STN-DBS is a safe and effective method to treat or improve IPD. Over the course of the past decades, STN-DBS has drastically improved. In particular, this is owing to higher imaging resolutions, the possibility of secondary image processing, to integrating complementary information and to the advancements in hard- and software. Therefore, STN-DBS has become quite reliable. The method of individualized miniframe integration deliberately makes use of the possibilities of automation. The group comparison between conventional and individualized frames in stereotaxy confirms that the use of automation in DBS fulfills the requirements in terms of accuracy and feasibility when compared with other methods. The use of either method results in an almost identical effect of DBS with regard to medication reduction and improvement to fine motor performance capability. The significant time saving achieved through the use of the miniframe system helps to compensate for the additional system production costs incurred.
Declaration of interest
This manuscript has not been previously published in whole or in part or submitted elsewhere for review. There are no sources of financial or material support to declare. The authors report no declarations of interest.
References
- Kopper F, Volkmann J, Müller D, et al. High-frequency deep brain stimulation in the treatment for Parkinson's disease, tremor and dystonia. Der Nervenarzt 2003;74:709–725.
- Beric A, Kelly PJ, Rezai A, et al. Complications of deep brain stimulation surgery. Stereotact Funct Neurosurg 2001;77:73–78.
- Krack P, Pollak P, Limousin P, et al. Subthalamic nucleus or internal pallidal stimulation in young onset Parkinson's disease. Brain 1998;121:451–457.
- Yelnik J, Percheron G. Subthalamic neurons in primates: a quantitative and comparative analysis. Neuroscience 1979;4:1717–1743.
- Hutchison WD, Allan RJ, Opitz H, et al. Neurophysiological identification of the subthalamic nucleus in surgery for Parkinson's disease. Ann Neurol 1998;44:622–628.
- Raeva S, Lukashev A, Lashin A. Unit activity in human thalamic reticularis nucleus. I. Spontaneous activity. Electroencephalogr Clin Neurophysiol 1991;79:133–140.
- Minguez-Castellanos A, Escamilla-Sevilla F, Katati MJ, et al. Different patterns of medication change after subthalamic or pallidal stimulation for Parkinson's disease: target related effect or selection bias. J Neurol Neurosurg Psychiatr 2005;76:34–39.
- Pinsker MO, Herzog J, Falk D, et al. (Accuracy and distortion of deep brain stimulation electrodes on postoperative MRI and CT. Zentralbl Neurochir 2008;69:144–147.
- Winkler D, Hammer N, Oehlwein C, et al. Implementing Conventional Zamorano Dujovny Frames versus Individually Manufactured microTargeting™ Platforms – a comparative study on deep brain stimulation in parkinson patients. Stereotact Funct Neurosurg 2013;91:392–398.
- Kondziolka D, Dempsey PK, Lunsford LD, et al. A comparison between magnetic resonance imaging and computed tomography for stereotactic coordinate determination. Neurosurgery 1992;30:402–406; discussion 406–407.
- Tir M, Devos D, Blond S, et al. Exhaustive, one-year follow-up of subthalamic nucleus deep brain stimulation in a large, single-center cohort of parkinsonian patients. Neurosurgery 2007;61:297–304; discussion 304–305.
- Slowinski JL, Putzke JD, Uitti RJ, et al. Unilateral deep brain stimulation of the subthalamic nucleus for Parkinson disease. J Neurosurg 2007;106:626–632.
- Derost P, Ouchchane L, Morand D, et al. Is DBS-STN appropriate to treat severe Parkinson disease in an elderly population. Neurology 2007;68:1345–1355.
- Moro E, Lozano AM, Pollak P, et al. Long-term results of a multicenter study on subthalamic and pallidal stimulation in Parkinson's disease. Mov Disord 2010;25:578–586.
- Fehér G, Balás I, Komoly S, et al. A kétoldali subthalamicus stimuláció hatékonysága az antiparkinson gyógyszereles változtatásának tükrében. Ideggyogy Sz 2010;63:314–319.
- Germano IM, Gracies J, Weisz DJ, et al. Unilateral stimulation of the subthalamic nucleus in Parkinson disease: a double-blind 12-month evaluation study. J Neurosurg 2004;101:36–42.
- Trittgemeyer M, Wollny G, Kruggel F. Visualising deformation fields computed by non-linear image registration. Comput Vis Sci 2002;45:45–51.
- Paek SH, Lee J, Kim H, et al. Electrode position and the clinical outcome after bilateral subthalamic nucleus stimulation. J Korean Med Sci 2011;26:1344–1355.
- Konrad PE, Neimat JS, Yu H, et al. Customized, miniature rapid-prototype stereotactic frames for use in deep brain stimulator surgery: initial clinical methodology and experience from 263 patients from 2002 to 2008. Stereotact Funct Neurosurg 2011;89:34–41.
- Balachandran R, Mitchell JE, Dawant BM, Fitzpatrick JM. Accuracy evaluation of microTargeting Platforms for deep-brain stimulation using virtual targets. IEEE Trans Biomed Eng 2009;56:37–44.
- Dötterl A, Trappe AE, Al-Hami S. Optimized visualization of contrast medium dependent differences in CT/MRI scans by RGB transformation. Minim Invasive Neurosurg 1999;42:194–197.
- Fitzpatrick JM, Konrad PE, Nickele C, et al. Accuracy of customized miniature stereotactic platforms. Stereotact Funct Neurosurg 2005;83:25–31.
- Erola T, Heikkinen ER, Haapaniemi T, et al. Efficacy of bilateral subthalamic nucleus (STN) stimulation in Parkinson's disease. Acta Neurochir (Wien) 2006;148:389–394.
- DeLong MR, Wichmann T. Deep brain stimulation for Parkinson's disease. Ann Neurol 2001;49:142–143.
- Winkler D, Tittgemeyer M, Schwarz J, et al. The first evaluation of brain shift during functional neurosurgery by deformation field analysis. J Neurol Neurosurg Psychiatr 2005;76:1161–1163.
- Nimsky C, Ganslandt O, Cerny S, et al. Quantification of, visualization of, and compensation for brain shift using intraoperative magnetic resonance imaging. Neurosurgery 2000;47:1070–1079; discussion 1079–1080.