Abstract
Introduction: Inflammation is a key pathological hallmark of several neurodegenerative disorders including Alzheimer’s disease, Parkinson’s disease and familial amyloidotic polyneuropathy (FAP). Among all inflammatory cytokines associated with FAP, IL-1β, in particular, has been implicated in playing a key pathogenic role. In the present study, we sought to investigate whether blocking IL-1β signaling provides disease-modifying benefits in an FAP mouse model. Methods: We assessed the effect of chronic administration of Anakinra, an IL-1 antagonist, on FAP pathogenesis in vivo, using real-time polymerase chain reaction (qPCR), semi-quantitative immunohistochemistry (SQ-IHC), western blot and nerve morphometric analyses.
Results: We found that treatment with Anakinra prevents transthyretin (TTR) extracellular deposition in sciatic nerve, protecting unmyelinated nerve fibers from aggregate-induced degeneration. Moreover, Anakinra administration significantly suppressed IL-1 signaling pathway and inhibited apoptosis and nitrative stress.
Conclusions: The present work highlights the relevance of the IL-1 signaling pathway in the pathophysiology of FAP. Our results bring to light the importance of non-amyloid targets in the therapeutic strategies for this disorder. Thus, we propose the use of Anakinra as a potential therapeutic agent for TTR-related amyloidosis.
Introduction
Inflammation is an essential protective response being a major cofactor involved in the pathogenesis of numerous conditions that are often considered non-inflammatory such as diabetes [Citation1], atherosclerosis, cancer or amyloid-related disorders [Citation2]. Different neurodegenerative diseases share an excessive activation of common pathogenic pathways, including overexpression of tumor necrosis factor α (TNF-α) and interleukin-1β (IL-1β) as in Alzheimer’s, Huntington’s or Parkinson’s disease [Citation3]. Inflammation is also observed in familial amyloidotic polyneuropathy (FAP) [Citation4], an autosomal dominant hereditary disorder characterized by the extracellular deposition of mutant transthyretin (TTR) aggregates and amyloid fibrils, particularly in the peripheral nervous system. Accumulation of TTR aggregates and fibrils in the peripheral nervous system leads to peripheral neuropathy, with reduced nerve fiber density and destruction of endoneurial blood vessels [Citation5].
TTR is mainly expressed by the liver and the choroid plexuses of brain [Citation6] and more than one hundred single point mutations have been described for TTR promoting amyloidogenesis [Citation7]. The most common TTR mutation in FAP results from an exchange of a methionine for a valine at position 30 – TTR V30M [Citation8]. The mechanism underlying TTR amyloid fibril formation is not fully understood. It has been proposed that fibrillogenesis requires dissociation of TTR homotetrameric structure into misfolded monomers which self-assemble forming non-fibrillar oligomers, protofibrils and finally mature amyloid fibers [Citation9,Citation10].
It has been shown that oligomeric and fibrillar TTR species bind to the receptor for advanced glycation end products (RAGE) leading to activation and nuclear translocation of nuclear factor κB (NF-κB) [Citation11], which is known to bind as dimers to promoters and enhancers of a variety of inflammation-associated molecules and oxidative stress intermediaries, regulating their transcription [Citation12,Citation13]. The pro-inflammatory cytokines will in turn promote NF-κB activation [Citation14] with consequent up-regulation of RAGE expression via the NF-κB binding elements in the RAGE promoter [Citation15] ending up in a circular regulation that prompts disease, with destructive cell responses. Pro-inflammatory mechanisms are likewise upregulated in FAP, especially in endoneurial axons, with increased expression of TNF-α, macrophage colony-stimulating factor and IL-1β since earlier stages of disease and increase with the ongoing neurodegenerative process [Citation4,Citation16]. IL-1 exists in two forms: IL-1α and IL-1β exerting its pleiotropic effects by binding to the IL-1 type 1 receptor. IL-1β is a primary regulator of inflammatory and immune responses being also involved in hypotension, fever, neutrophilia, thrombocytosis and in the production of acute-phase proteins [Citation17]. This cytokine can induce apoptosis of different types of cells and stimulate inducible nitric oxide synthase leading to stress responses [Citation18]. IL-1 receptor antagonist (IL-1Ra) is a member of the IL-1 family and is a natural endogenous inhibitor of both IL-1α and IL-1β, which competitively bind to the IL-1 type 1 receptor without activating it and thus prevents binding and intracellular IL-1 signal transduction. It is produced by hepatocytes, smooth muscle cells and macrophages as an anti-inflammatory acute-phase protein [Citation19].
A non-glycosylated recombinant human IL-1Ra (Anakinra), with similar properties to endogenous IL-1Ra, inhibits IL-1 by binding to the IL-1 type 1 receptor; it is a Food and Drug Administration approved drug with application in several human diseases [Citation20,Citation21] and successful results in clinical trials of human patients with rheumatoid arthritis [Citation22] or type 2 diabetes [Citation23].
Since neuroinflammation is associated with FAP peripheral nerve, the aim of the present study was to investigate the effect of blockage IL-1 signaling pathway, by Anakinra, on pathological cascades in the disease. To perform this work, we used a recent developed mouse model [Citation24], carrying the human TTR V30M gene, in a TTR null background and heterozygous for the heat shock factor-1 (Hsf-1), here designated as Hsf/V30M mice.
Methods
Ethics statement
All animal experiments were carried out in accordance with the European Community Council Directive (2010/63/EU) and were approved by the Institutional and National General Veterinarian Board ethical committees.
Experimental groups and treatment
Transgenic mice for human TTR V30M, in the 129/Sv and endogenous Ttr null background, heterozygous for Hsf-1 (labeled as Hsf/V30M), with 4.5 months (n = 10) were treated daily with subcutaneous injections of Anakinra at 25 mg/kg (Kineret®, Biovitrum, Stockholm, Sweden), over 6 weeks. Age-matched control Hsf/V30M untreated mice were injected with phosphate buffer saline (PBS, n = 10). Animals were housed in pathogen-free conditions, in a controlled temperature room, maintained under a 12-h light/dark period, with water and food ad libitum. At the end of treatment, mice were sacrificed with a lethal injection of a premixed solution containing ketamine (75 mg/kg) plus medetomidine (1 mg/kg). Unless otherwise mentioned, all reagents were obtained from Sigma-Aldrich, St. Louis, MO, and were of analytical grade.
RNA extraction, cDNA synthesis and real-time polymerase chain reaction
RNA from livers was isolated by phenol extraction (Invitrogen, Carlsbad, CA) (n = 10 controls and n = 10 treated mice). Dorsal root ganglia (DRG) were dissected free from the spinal cord and RNA extraction (n = 7 of each group) performed with RNeasy Mini columns (Qiagen, Gaithersburg, MD). cDNA was synthesized using the SuperScript double-stranded cDNA Kit (Invitrogen, Carlsbad, CA). The quality of extracted RNA was measured using Experion RNA StdSens Analysis Kit (Bio-Rad); real-time polymerase chain reaction (qPCR) was performed with duplicates using iQ Syber Green Super Mix (Bio-Rad, Hercules, CA), and reactions were run on an Bio-Rad iQ5 software.
Primer sequences were designed using Beacon Designer™ 8 (Premier Biosoft, Palo Alto, CA) () for the following genes: TTR, Il-1β, Tnf-α, interleukin-1 receptor-associated kinase 1 (Irak1), myeloid differentiation primary response gene 88 (Myd88), heat shock protein-27 (hsp-27) and glyceraldehyde 3-phosphate dehydrogenase (Gapdh). Differential expression was determined by the 2−ΔΔCT method using Gapdh as housekeeping gene.
Table 1. Primer sequences for forward and reverse probes used in qPCR.
Semi-quantitative immunohistochemistry
Sciatic nerve, DRG, stomach and colon (n = 10) were collected to 10% formalin, and 3-μm-thick paraffin sections were cut, deparaffinated in histoclear (National Diagnostics, Atlanta, GA) and hydrated in a descent alcohol series. Endogenous peroxidase activity was inhibited with 3% hydrogen peroxide solution in methanol, and the sections were blocked in 10% fetal bovine serum and 0.5% Triton X-100 in PBS. Primary antibodies used were: rabbit polyclonal anti-human TTR (1:600, DAKO, Glostrup, Denmark), goat polyclonal anti-IL-1β (1:25, Santa Cruz Biotechnology, Santa Cruz, CA), rabbit polyclonal anti-Fas death receptor (1:100, Santa Cruz Biotechnology, Santa Cruz, CA), rabbit polyclonal anti-cleaved caspase-3 (1:100, R&D Systems, Minneapolis, MN), rabbit polyclonal anti-3-nitrotyrosine (1:500, Chemicon, Temecula, CA) and rabbit polyclonal anti-NF-κB p65 (1:25, Santa Cruz Biotechnology, Santa Cruz, CA). Antigen visualization was performed with biotin-extravidin-peroxidase ABC kit (Vector, Burlingame, CA) using 3.3′-diaminobenzidine (Sigma-Aldrich, St. Louis, MO) as substrate. Semi-quantitative analysis of the immunostaining area was performed with the Image Pro-plus 5.1 software (Media Cybernetics, Rockville, MD). Results shown represent the area occupied by pixels, corresponding to the immunohistochemical substrate color, normalized relatively to the total area. Immunohistochemistry analysis was blind to treatment, performed by two independent researchers, and each animal tissue was evaluated in five different areas, with 20× magnification.
Total protein extracts and Western blot analysis
Protein extracts were obtained by homogenizing, in ice, DRG from control (n = 7) and Anakinra-treated mice (n = 7), in lysis buffer containing 5 mM ethylenediamine tetraacetic acid, 2 mM ethylene glycol tetraacetic acid, 20 mM 3-(N-morpholino)propanesulfonic acid, 0.5% Triton X-100, 30 mM sodium fluoride, 40 mM sodium pyrophosphate, 1 mM sodium orthovanadate, 1 mM phenylmethylsulphonyl fluoride and a protease inhibitor mix (GE Healthcare, Buckinghamshire). Total protein concentration was determined by the Bradford protein assay (Bio-Rad).
Total protein from each extract (50 µg per lane) was separated in 12% SDS-PAGE gels and transferred onto a nitrocellulose Whatman™ membrane (GE Healthcare, Buckinghamshire) using a Mini Trans-Blot Cell system (Bio-Rad). After blocking in 5% bovine serum albumin in PBS, the membrane was incubated overnight at 4 °C with rabbit polyclonal anti-binding immunoglobulin protein (BiP; 1:1000, Abcam, Cambridge) and mouse monoclonal anti-α-Tubulin (1:10,000, Sigma-Aldrich, St. Louis, MO), followed by incubation with anti-rabbit or anti-mouse antibodies conjugated to horsederadish peroxidase (1:5,000, 1:2,500 respectively, The Binding Site, Birmingham). Detection was performed by the Luminata™ Crescendo (enhanced chemiluminescence, Millipore, Billerica, MA). Quantitative analysis was carried out by densitometry using Quantity One software (Bio-Rad). Density values were normalized to α-Tubulin levels.
Morphometric analysis
Sciatic nerves (3 mm length) from control (n = 6) and Anakinra-treated (n = 6) animals were removed and fixed overnight in a 0.1-M sodium cacodylate solution containing 1.25% glutaraldehyde and 4% paraformaldehyde. Tissues were washed 3 × 30 min in 0.1 M sodium cacodylate and post-fixed with 2% osmium tetroxide in the cacodylate solution, overnight at 4 °C. After a new wash of 3 × 30 min, the tissue was dehydrated using a series of graded acetone and finally embedded in spurr. Transverse sections were cut (0.5 µm thick) with a SuperNova Reichert Leica ultramicrotome (Wetzlar, Germany) and stained with 1% toluidine blue, for 15 s, in an 80 °C heating plate, before being examined on a light microscope. For each animal, the total number of myelinated fibers present in one semithin section was determined by counting 40× magnified photographs covering the whole nerve area. Regarding unmyelinated fibers, ultrathin transverse sections were cut and stained with lead uranyl acetate. Sections were then mounted on 400 mesh copper grids and examined by transmission electron microscopy (Jeol JEM-1400, Tokyo, Japan). For unmyelinated fiber density analysis, 40 non-overlapping photographs (12,000 amplification) per sciatic nerve were selected and fibers counted, normalized to the area of each ultrathin section.
Statistical analysis
All data are expressed as mean values ± standard error of the mean (SEM). Comparison between Anakinra treated and control group was performed using the Student’s t test. p values of less than 0.05 were considered to be significant (*p < 0.05; **p < 0.01; ***p < 0.001).
Results
Anakinra prevents non-fibrillar TTR deposition in sciatic nerve and does not alter TTR expression by the liver
We decided to test the effect of Anakinra in preventing TTR aggregation using the Hsf/V30M FAP mouse model. In this model, when animals have 6 months of age, non-fibrillar deposits are observed, not only in the gastrointestinal tract but also in the autonomic and peripheral nervous system [Citation24]. We treated 4.5-month-old Hsf/V30M mice, thus before TTR starts to deposit in the peripheral nervous system. Therefore, we were able to evaluate the effect of Anakinra on preventing non-fibrillar TTR aggregates deposition in the peripheral nervous system and to investigate the contribution of IL-1 signaling in the pathological mechanisms of disease. Some reports show that mice respond to Anakinra in a dose range of 1 mg/kg to 100 mg/kg [Citation25,Citation26] with no signals of toxicity. Based on this and to be as closest as possible to the clinical human dose, in our study we chose the dose of 25 mg/kg. Since Anakinra has a short half-life, daily subcutaneous injections were selected. Accordingly with previous literature [Citation27], no major secondary effects besides the topic reaction in the local of the injection were detected.
At the end of the treatment period, animals were 6 months old and displayed widespread TTR non-fibrillar deposition along the gastrointestinal tract and the peripheral nervous system. Treated mice presented a markedly reduction on TTR deposition in sciatic nerve (43%) as compared to non-treated animals, and a trend, although not statistically significant, was observed in DRG (). In the gastrointestinal tract, no difference regarding TTR deposition was observed between treated and control mice (data not shown).
Figure 1. Anakinra prevents TTR deposition in the sciatic nerve and does not alter TTR expression by the liver. (A) Representative pictures of TTR load in sciatic nerve and DRG of Hsf/V30M mice treated with Anakinra (right panels, n = 10) and age-matched controls (left panels, n = 10). Scale bar 50 µm. Charts represent the quantification of immunohistochemical images, and data represent mean ± SEM (*p < 0.05). (B). Histogram represents TTR mRNA levels in the liver of Anakinra-treated mice (n = 10), as compared with controls (n = 10). Gapdh was the housekeeping gene for normalization. Data represent mean ± SEM.
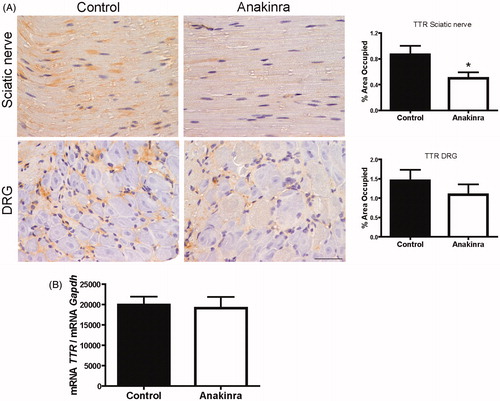
We next investigated TTR mRNA levels by qPCR and found that Anakinra did not alter liver TTR transcription ().
Anakinra prevents neurodegeneration
Fiber degeneration, first affecting the unmyelinated and low-diameter myelinated fibers, is a clinical feature of FAP [Citation5,Citation28]. We next analyzed the density of myelinated and unmyelinated fibers in sciatic nerve of Anakinra-treated animals and untreated age-matched controls. Treatment prevented loss of 46% of unmyelinated fibers, as compared with controls, with no impact on myelinated fibers ().
Figure 2. Anakinra prevents neurodegeneration in the Hsf/V30M FAP mouse model. Morphometric analyses of sciatic nerve segments in Anakinra-treated and control animals. (A) Fiber density assessed in semithin sections showing no impact of IL-1 blocking on myelinated fibers. Scale bar: 50 µm, n = 6 animals within each group. (B) Electron microscopy representative images, and correspondent chart, indicating higher unmyelinated fiber density in Anakinra-treated animals, as compared to controls. Scale bar: 2 µm, n = 6 animals per group (*p < 0.05).
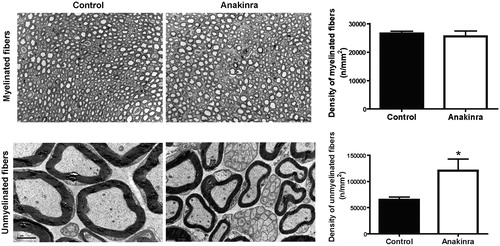
Anakinra decreases expression of IL-1 signaling pathway mediators and NF-κB p65 nucleus translocation
Transcription of pro-inflammatory mediators in DRG was analyzed by qPCR. Il-1β, Myd88 and Irak1 levels were found reduced in Anakinra-treated animals (77, 36 and 48% reduction, respectively; ). Regarding Tnf-α expression, we did not observe any significant difference between control and Anakinra-treated animals ().
Figure 3. Expression of IL-1 signaling pathway mediators and evaluation of NF-κB activation throughout p65 subunit translocation to the nucleus. (A) Downregulation of pro-inflammatory mediators expression in DRG of Anakinra-treated animals (n = 7), as compared to controls (n = 7), evaluated by qPCR (*p < 0.05). Normalization was performed against Gapdh mRNA. No impact of Anakinra was observed regarding Tnf-α expression. (B) IL-1β protein levels in the peripheral nervous system assessed by SQ-IHC (n = 10 per group), with respective semi-quantification (presented as mean ± SEM; ***p < 0.001; *p < 0.05), demonstrating lower immunostaining in Anakinra-treated mice in both nerve and DRG. Scale bar 50 µm. (C) Representative SQ-IHC pictures of p65 NF-κB subunit in sciatic nerve of mice treated with Anakinra as compared to age-matched controls (n = 10 in each group). Mice treated with Anakinra presented lower p65 nuclear staining as compared with controls (arrows), suggesting a reduction in p65 nucleus translocation. Scale bar 50 µm. Chart shows quantification of immunohistochemical images, and data represent mean ± SEM (**p < 0.01).
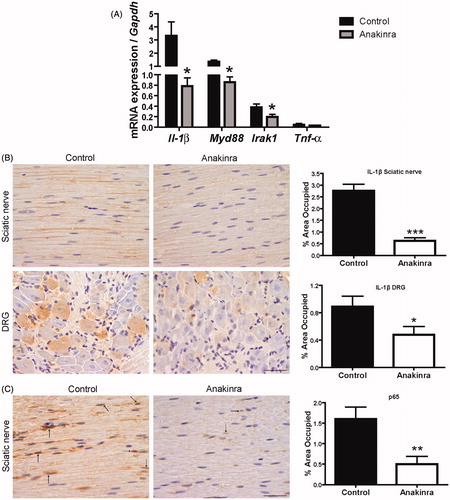
Since Il-1β mRNA was downregulated in DRG of Anakinra-treated mice, we next assessed IL-1β protein levels in the peripheral nervous system by semi-quantitative immunohistochemistry (SQ-IHC). We found that this pro-inflammatory cytokine was significantly reduced in treated as compared with untreated animals, in both sciatic nerve and DRG ().
A previous report has shown that TTR aggregates bind to RAGE and activate NF-κB [Citation11]. Since Anakinra prevented TTR deposition in nerve tissue, associated with neuroprotection, we next addressed whether these effects influence or might be influenced by NF-κB activation. By SQ-IHC analysis, we found minor cytoplasmic and nuclear labeling of NF-κB p65 subunit in sciatic nerve of mice treated with Anakinra, suggesting a reduction of this transcription factor nucleus translocation (, arrows). Thus, our data indicate Anakinra suppression of the general activity of IL-1 and NF-κB p65 nucleus translocation.
Blocking IL-1 signals prevents neuronal apoptosis and Hsp-27 transcription
Prevention of apoptosis by Anakinra was previously described in a rat islet model and in a model of experimental acute myocardial infarction [Citation18,Citation25]. Since apoptosis has also been implicated on the pathogenic mechanisms in FAP [Citation29], we next investigated the effect of Anakinra in Fas death receptor and cleaved caspase-3 levels in the peripheral nervous system. Both apoptotic markers were found significantly reduced in sciatic nerve and DRG of Anakinra-treated mice, as compared with untreated age-matched controls (). These results prompted us to investigate the transcriptional levels of Hsp-27 in DRG. We observed a significant decrease (70% reduction) in Hsp-27 mRNA levels in mice treated with Anakinra as compared to the untreated group ().
Figure 4. Anakinra suppresses apoptotic cell death in the peripheral nervous system and reduces expression of Hsp-27. (A) Representative SQ-IHC images of Fas death receptor in the peripheral nervous system and respective quantifications (n = 10 per group), demonstrating lower levels of Fas in treated animals. Data represent mean ± SEM (***p < 0.001). (B) Cleaved caspase-3 immunostaining in sciatic nerve and DRG of Hsf/V30M mice treated with Anakinra (right panels, n = 10) and age-matched controls (left panels, n = 10). Scale bar 50 µm. Charts characterize the quantification of immunohistochemical images, and data represent mean ± SEM (**p < 0.01 and ***p < 0.001). (C) Inhibition of Hsp-27 mRNA levels in DRG of Anakinra-treated mice as compared with controls (n = 7 of each group). The relative mRNA expression was normalized to Gapdh (***p < 0.001).
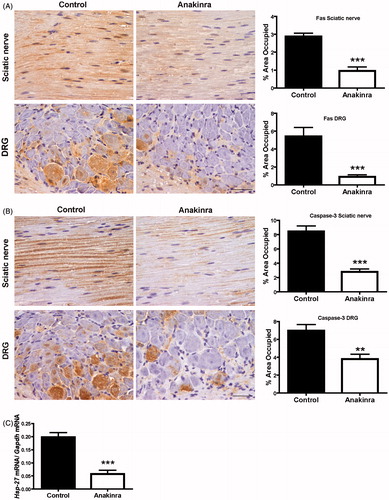
Blocking IL-1 signaling pathway prevents endoplasmic-reticulum (ER) and nitrative stress
Evidence suggests a link between inflammation driven by IL-1 and ER stress [Citation30], pathway also involved in the progress of FAP disease [Citation31]. To test this hypothesis in our model, we decided to evaluate levels of BiP, a central regulator for ER stress, in DRG. By western blotting, we found a significant reduction of BiP in treated mice (), indicating a direct effect of Anakinra in ER stress, since TTR deposition in this organ is not significantly affected.
Figure 5. Anakinra reduced ER and nitrative stress. (A) Western blot quantification of BiP in DRG from mice treated with Anakinra (n = 7) and non-treated age-matched controls (n = 7). Histogram denotes normalized BiP/Tubulin density quantification ± SEM (*p < 0.05). (B) Representative SQ-IHC pictures of 3-nitrotyrosine in sciatic nerve of mice treated with Anakinra (right panel, n = 10) and age-matched controls (left panel, n = 10). Scale bar 50 µm. Chart represents the quantification of immunohistochemical images, and data represent mean ± SEM (**p < 0.01).
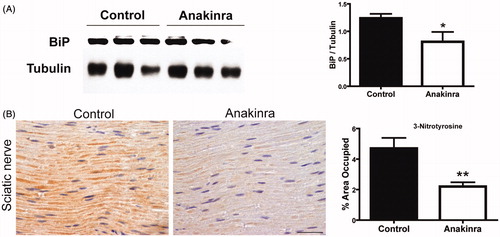
Nitration of tyrosine residues has been suggested as a marker of peroxynitrite-mediated tissue injury in TTR amyloidosis [Citation32] being the nitrative stress an integral factor in neurodegeneration. Accordingly, we further investigated whether neuroprotection of unmyelinated axons could also result from decreased levels of peroxynitrite formation. Sciatic nerve was immunohistochemically examined for evidence of 3-nitrotyrosine, a stable biochemical marker for this process. 3-Nitrotyrosine reactivity was detected within axons and was remarkably decreased after blocking IL-1 ().
Discussion
The presence of congophilic amyloid deposits is a unifying characteristic of the extensive analysis of FAP nerves. Nevertheless, the lack of a cause–effect relationship between amyloid deposition and neurodegeneration in FAP suggests that other TTR molecular intermediaries, namely pre-fibrillar oligomeric species, might be the primary neurotoxic culprits [Citation16,Citation33,Citation34]. In fact, it has been shown that nerves from FAP 0 patients (asymptomatic individuals presenting aggregate deposition but lacking amyloid) exhibit early signs of oxidative damage and inflammatory stress [Citation4]. Moreover, TTR non-fibrillar deposition in the sciatic nerves of FAP patients has been shown to induce pro-inflammatory cytokine synthesis by axons and a correlation between increased IL-1β levels and fiber degeneration has been established [Citation4].
In the present study, we investigated the effect of early administration (before aggregate deposition started) of the IL-1 antagonist Anakinra in TTR deposition and neurotoxicity, using a well-established mouse model of FAP.
We found that inhibition of IL-1 lowered the pre-fibrillar TTR deposition in sciatic nerve, which correlated with increased number of unmyelinated fibers. In contrast, Anakinra treatment did not interfere with TTR deposition in the gastrointestinal tract. This observation might be explained by the presence of endogenous microflora, exposition to oral pathogens and specific immune regulatory networks of the gastrointestinal tract, not operating in the peripheral nerve.
IL-1 has been implicated in several chronic degenerative disease processes, including the development of atherosclerosis and rheumatoid arthritis [Citation35,Citation36], being a key player in neuroinflammation and neurodegeneration, and regulating the expression of several transcription factors, including NF-κB [Citation37]. IL-1 activation results in phosphorylation and degradation of IκB proteins, releasing NF-κB and allowing its translocation to the nucleus, where it controls the expression of downstream pro-inflammatory genes [Citation14]. The involvement of NF-kB in FAP has been described [Citation11,Citation24,Citation38]. In vitro studies have shown that TTR aggregates interact with RAGE triggering nuclear translocation of NF-kB [Citation11].
In this study, we assessed the effect of IL-1 inhibition on NF-κB activation in sciatic nerve and found a significant reduction of NF-κB p65 nucleus translocation in Anakinra-treated animals, which is consistent with decreased TTR extracellular load and increased density of unmyelinated fibrils. It is important to emphasize that the influence of NF-κB on cell survival can be protective or deleterious, depending on the cell type, its developmental stage and the pathological conditions [Citation39]. For instance, in glia, NF-κB is inducible and mediates inflammatory responses that aggravate diseases such as ischemia, autoimmune encephalomyelitis and Alzheimer’s disease [Citation39,Citation40]. Thus, inhibition of NF-κB in glia might ameliorate disease, whereas activation in neurons might enhance memory [Citation39]. In FAP, NF-κB activation occurs as a response to extracellular aggregate insult. Whether this action is neuroprotective or neurotoxic, it is still not fully understood, but there is probably a threshold beyond which NF-κB activation starts to be noxious to cells. Moreover, the fact that other inflammatory mediators such as Tnf-α levels were not affected by the treatment support the specificity of Anakinra action into the IL-1 signaling pathway.
In FAP, like in other neurodegenerative disorders, accumulation of aberrant misfolded proteins activates the unfolded protein response (UPR) by the ER to restore and maintain homeostasis in the ER [Citation31,Citation41]. However, if the ER stress is continued, or the adaptive responses fail, apoptotic cell death ensues. Thus, we further investigated the effect of Anakinra administration on ER chaperone BiP/GRP78 and found that it significantly impairs ER-stress in treated animals.
Compelling evidence has shown that members of the IL-1 family of cytokines are intricately involved in inflammatory and death-inducing signaling platforms. Therefore, IL-1 has been further suggested as a rational therapeutic target for several pathological conditions. In vivo administration of IL-1 receptor antagonist in mice models as diverse as acute myocardial infarction [Citation25], spinal cord injury [Citation42], renal ischemia-reperfusion [Citation43] or cancer chemotherapy [Citation44] has been proved efficient in preventing activation of pro-apoptotic molecules or activating pro-survival signaling transduction pathways. In agreement with these observations, in our study early treatment of FAP mice with Anakinra resulted in significantly decreased levels of Fas death-receptor, cleaved caspase-3 and chaperone Hsp-27 in the peripheral nervous system.
The reaction of nitric oxide with superoxide produces the peroxynitrite anion [Citation45]. Peroxynitrite is a powerful oxidant that can nitrate aromatic amino acid residues such as tyrosine to form 3-nitrotyrosine. Hence, 3-nitrotyrosine is a valuable marker of peroxynitrite production. Immunostaining of FAP nerves with antibody to 3-nitrotyrosine demonstrated immunoreactive material in FAP 0 and FAP tissue in an axonal distribution [Citation4]. Our results indicate significant decrease of 3-nitrotyrosine in the sciatic nerve, which is in accordance with Anakinra inhibitory effect on TTR deposition and suggestive of reduction of cytotoxicity and neuroprotection.
Since Anakinra has no effect on TTR stability (unpublished data), we hypothesized that decreased TTR aggregation and deposition in tissues might be due to a restored extracellular environment characterized by decreased levels of inflammatory mediators, nitrative species and apoptotic cell death. Thus, by interrupting the positive feedback loop between TTR accumulation in tissues and inflammation, Anakinra prevents TTR deposition in the peripheral nervous system. Future studies will further elucidate the molecular mechanisms behind Anakinra inhibitory effect on TTR deposition.
Therapeutic strategies for FAP, other than orthotropic liver transplantation, have been put forward as it is the case of tetrameric TTR stabilization [Citation46,Citation47], inhibition of TTR aggregation [Citation47,Citation48] or apoptosis [Citation29], disruption of TTR fibrils [Citation49], suppression of liver TTR synthesis with small interfering RNA (siRNA) [Citation50,Citation51] and drug combinatorial treatments [Citation52]. In what concerns combinatorial therapeutic strategies in FAP, doxycycline and tauroursodeoxycholic acid administration were shown to synergize for disruption and clearance of fibrillar deposits and for stabilization of disease progression both in mice and humans, respectively [Citation52,Citation53]. Considering the therapeutic potential of Anakinra shown in our study, we hypothesize that it can be a multi-target drug used as an alternative treatment or in a combinatorial approach, improving the efficacy of drugs acting on TTR silencing [Citation51], TTR stabilization [Citation46,Citation47] or amyloid fiber disruption [Citation49].
Overall, the present study brings to light, for the first time, the therapeutic potential of Anakinra in the context of FAP.
Conclusion
The present study paves the way to the therapeutic potential of Anakinra in FAP. Anakinra administration lead to the reduction of non-fibrillar TTR deposits in nerve tissue, inflammation mediated by IL-1, apoptosis, ER and nitrative stress, ultimately preventing unmyelinated fibers from degeneration. Furthermore, data reinforce the importance of inflammation on TTR amyloidosis and further demonstrate an important role of IL-1 in the pathogenesis of FAP.
Declaration of interest
The authors declare no competing financial or personal interests. This work was supported by FEDER funds through the Operational Competitiveness Program – COMPETE, a grant from Cordex and by national funding from Portuguese Foundation for Science and Technology (FCT) under the project PTDC/BIM-MEC/0282/2012, and fellowship to NPG (SFRH/BD/74304/2010).
Abbreviations | ||
BiP | = | binding immunoglobulin protein |
DRG | = | dorsal root ganglia |
ER | = | endoplasmic reticulum |
FAP | = | familial amyloidotic polyneuropathy |
Gapdh | = | glyceraldehyde 3-phosphate dehydrogenase |
Hsf-1 | = | heat shock factor 1 |
IL-1 | = | interleukin-1 |
Hsp-27 | = | heat shock protein-27 |
IL-1Ra | = | interleukin-1 receptor antagonist |
Irak1 | = | interleukin-1 receptor associated kinase-1 |
Myd88 | = | myeloid differentiation primary response gene 88 |
NF-κB | = | nuclear factor κB |
PBS | = | phosphate buffer saline |
qPCR | = | real-time polymerase chain reaction |
RAGE | = | receptor for advanced glycation end products |
SEM | = | standard error of the mean |
siRNA | = | small interfering RNA |
mRNA | = | messenger RNA |
SQ-IHC | = | semi-quantitative immunohistochemistry |
TNF-α | = | tumor necrosis factor α |
TTR | = | transthyretin |
UPR | = | unfolded protein response |
V30M | = | valine 30 methionine |
Acknowledgements
The authors would like to thank Rui Fernandes and Paula Gonçalves from IBMC for help with electron microscopy, paraffin tissue processing and immunohistochemical analysis, respectively. We also thank Nelson Ferreira for critical reading.
References
- Ehses JA, Lacraz G, Giroix MH, Schmidlin F, Coulaud J, Kassis N, Irminger JC, et al. IL-1 antagonism reduces hyperglycemia and tissue inflammation in the type 2 diabetic GK rat. Proc Natl Acad Sci USA 2009;33:13998–4003
- Hallegua DS, Weisman MH. Potential therapeutic uses of interleukin 1 receptor antagonists in human diseases. Ann Rheum Dis 2002;61:960–7
- Pizza V, Agresta A, D'Acunto CW, Festa M, Capasso A. Neuroinflamm-aging and neurodegenerative diseases: an overview. CNS Neurol Disord Drug Targets 2011;10:621–34
- Sousa MM, Du Yan S, Fernandes R, Guimaraes A, Stern D, Saraiva MJ. Familial amyloid polyneuropathy: receptor for advanced glycation end products-dependent triggering of neuronal inflammatory and apoptotic pathways. J Neurosci 2001;21:7576–86
- Coimbra A, Andrade C. Familial amyloid polyneuropathy: an electron microscope study of peripheral nerve in five cases. II. Nerve fibril changes. Brain 1971;94:207–12
- Herbert J, Wilcox JN, Pham KT, Fremeau RT, Zeviani MJ, Dwork A, Soprano DR, et al. Transthyretin: a choroid plexus-specific transport protein in human brain. The 1986 S. Weir Mitchell award. Neurology 1986;36:900–11
- Rowczenio D, Wechalekar A. Mutations in hereditary amyloidosis. Available from: http://amyloidosismutations.com ((c) 2010)
- Saraiva MJ, Birken S, Costa PP, Goodman DS. Amyloid fibril protein in familial amyloidotic polyneuropathy, Portuguese type. Definition of molecular abnormality in transthyretin (prealbumin). J Clin Invest 1984;74:104–19
- Colon W, Kelly JW. Partial denaturation of transthyretin is sufficient for amyloid fibril formation in vitro. Biochemistry 1992;31:8654–60
- Quintas A, Vaz DC, Cardoso I, Saraiva MJ, Brito RM. Tetramer dissociation and monomer partial unfolding precedes protofibril formation in amyloidogenic transthyretin variants. J Biol Chem 2001;276:27207–13
- Sousa MM, Yan SD, Stern D, Saraiva MJ. Interaction of the receptor for advanced glycation end products (RAGE) with transthyretin triggers nuclear transcription factor kB (NF-kB) activation. Lab Invest 2000;80:1101–10
- Collins T, Read MA, Neish AS, Whitley MZ, Thanos D, Maniatis T. Transcriptional regulation of endothelial cell adhesion molecules: NF-kappa B and cytokine-inducible enhancers. FASEB J 1995;9:899–909
- Rhee JW, Lee KW, Kim D, Lee Y, Jeon OH, Kwon HJ, Kim DS. NF-kappaB-dependent regulation of matrix metalloproteinase-9 gene expression by lipopolysaccharide in a macrophage cell line RAW 264.7. J Biochem Mol Biol 2007;40:88–94
- Hayden MS, Ghosh S. Signaling to NF-κB. Genes Dev 2004;18:2195–224
- Li J, Schmidt AM. Characterization and functional analysis of the promoter of RAGE, the receptor for advanced glycation end products. J Biol Chem 1997;272:16498–506
- Sousa MM, Cardoso I, Fernandes R, Guimarães A, Saraiva MJ. Deposition of transthyretin in early stages of familial amyloidotic polyneuropathy: evidence for toxicity of nonfibrillar aggregates. Am J Pathol 2001;159:1993–2000
- Gabey C, Lamacchia C, Palmer G. IL-1 pathways in inflammation and human diseases. Nat Rev Rheumatol 2010;6:232–41
- Schwarznau A, Hanson MS, Sperger JM, Schram BR, Danobeitia JS, Greenwood KK, Vijayan A, et al. IL-1beta receptor blockade protects islets against pro-inflammatory cytokine induced necrosis and apoptosis. J Cell Physiol 2009;220:341–47
- Arend WP, Malyak M, Guthridge CJ, Gabay C. Interleukin-1 receptor antagonist: role in biology. Annu Rev Immunol 1998;16:27–55
- Dinarello CA. A clinical perspective of IL-1β as the gatekeeper of inflammation. Eur J Immunol 2011;41:1203–17
- Dinarello CA, Simon A, Van der Meer JW. Treating inflammation by blocking interleukin-1 in a broad spectrum of diseases. Nat Rev Drug Discov 2012;11:633–52
- Botsios C, Sfriso P, Furlan A, Ostuni P, Biscaro M, Fiocco U, Todesco S, et al. Anakinra, a recombinant human IL-1 receptor antagonist, in clinical practice. Outcome in 60 patients with severe rheumatoid arthritis. Reumatismo 2007;59:32–7
- Larsen CM, Faulenbach M, Vaag A, Volund A, Ehses JA, Seifert B. Interleukin-1-receptor antagonist in type 2 diabetes mellitus. N Engl J Med 2007;356:1517–26
- Santos SD, Fernandes R, Saraiva MJ. The heat shock response modulates transthyretin deposition in the peripheral and autonomic nervous systems. Neurobiol Aging 2010;31:280–9
- Abbate A, Salloum FN, Vecile E, Das A, Hoke NN, Straino S, Biondi-Zoccai GG, et al. Anakinra, a recombinant human interleukin-1 receptor antagonist, inhibits apoptosis in experimental acute myocardial infarction. Circulation 2008;117:2670–83
- Salloum FN, Chau V, Varma A, Hoke NN, Toldo S, Biondi-Zoccai GG, Crea F, et al. Anakinra in experimental acute myocardial infarction-does dosage or duration of treatment matter? Cardiovasc Drugs Ther 2009;23:129–35
- Fleischmann R. Anakinra in the treatment of rheumatic disease. Expert Rev Clin Immunol 2006;2:331–40
- Araki S, Yi S. Pathology of familial amyloidotic polyneuropathy with TTR met 30 in Kumamoto, Japan. Neuropathology 2000;20:S47–51
- Macedo B, Batista AR, Ferreira N, Almeida MR, Saraiva MJ. Anti-apoptotic treatment reduces transthyretin deposition in a transgenic mouse model of Familial Amyloidotic Polyneuropathy. Biochim Biophys Acta 2008;1782:517–22
- Amin A, Choi SK, Galan M, Kassan M, Partyka M, Kadowitz P, Henrion D, et al. Chronic inhibition of endoplasmic reticulum stress and inflammation prevents ischaemia-induced vascularpathology in type II diabetic mice. J Pathol 2012;227:165–74
- Teixeira PF, Cerca F, Santos SD, Saraiva MJ. Endoplasmic reticulum stress associated with extracellular aggregates. Evidence from transthyretin deposition in familial amyloid polyneuropathy. J Biol Chem 2006;281:21998–2003
- Sousa MM, Ferrão J, Fernandes R, Guimarães A, Geraldes JB, Perdigoto R, Tomé L, et al. Deposition and passage of transthyretin through the blood-nerve barrier in recipients of familial amyloid polyneuropathy livers. Lab Invest 2004;84:865–73
- Andersson K, Olofsson A, Nielsen EH, Svehag SE, Lundgren E. Only amyloidogenic intermediates of transthyretin induce apoptosis. Biochem Biophys Res Commun 2002;294:309–14
- Reixach N, Deechongkit S, Jiang X, Kelly JW, Buxbaum JN. Tissue damage in the amyloidoses: transthyretin monomers and nonnative oligomers are the major cytotoxic species in tissue culture. Proc Natl Acad Sci USA 2004;101:2817–22
- Merhi-Soussi F, Kwak BR, Magne D, Chadjichristos C, Berti M, Pelli G, James RW, et al. Interleukin-1 plays a major role in vascular inflammation and atherosclerosis in male apolipoprotein E-knockout mice. Cardiovasc Res 2005;66:583–93
- Kay J, Calabrese L. The role of interleukin-1 in the pathogenesis of rheumatoid arthritis. Rheumatology (Oxford) 2004;43:iii2–9
- Beg AA, Finco TS, Nantermet PV, Baldwin AS Jr. Tumor necrosis factor and interleukin-1 lead to phosphorylation and loss of I kappa B alpha: a mechanism for NF-kappa B activation. Mol Cell Biol 1993;13:3301–10
- Sousa MM, do Amaral JB, Guimarães A, Saraiva MJ. Up-regulation of the extracellular matrix remodeling genes, biglycan, neutrophil gelatinase-associated lipocalin, and matrix metalloproteinase-9 in familial amyloid polyneuropathy. FASEB J 2005;19:124–6
- Kaltschmidt B, Uherek M, Volk B, Baeuerle PA, Kaltschmidt C. Transcription factor NF-kappaB is activated in primary neurons by amyloid beta peptides and in neurons surrounding early plaques from patients with Alzheimer disease. Proc Natl Acad Sci USA 1997;94:2642–7
- Mattson MP, Camandola S. NF-κB in neuronal plasticity and neurodegenerative disorders. J Clin Invest 2001;107:247–54
- Hoozemans JJ, van Haastert ES, Nijholt DA, Rozemuller AJ, Eikelenboom P, Scheper W. The unfolded protein response is activated in pretangle neurons in Alzheimer's disease hippocampus. Am J Pathol 2009;174:1241–51
- Nesic O, Xu GY, McAdoo D, High KW, Hulsebosch C, Perez-Pol R. IL-1 receptor antagonist prevents apoptosis and caspase-3 activation after spinal cord injury. J Neurotrauma 2001;18:947–56
- Rusai K, Huang H, Sayed N, Strobl M, Roos M, Schmaderer C. Administration of interleukin-1 receptor antagonist ameliorates renal ischemia-reperfusion injury. Transpl Int 2008;21:572–80
- Wu ZQ, Han XD, Wang Y, Yuan KL, Jin ZM, Di JZ, Yan J, et al. Interleukin-1 receptor antagonist reduced apoptosis and attenuated intestinal mucositis in a 5-fluorouracil chemotherapy model in mice. Cancer Chemother Pharmacol 2011;68:87–96
- Jourd’heuil D, Jourd’heuil FL, Kutchukian PS, Musah RA, Wink DA, Grisham MB. Reaction of superoxide and nitric oxide with peroxynitrite. Implications for peroxynitrite-mediated oxidation reactions in vivo. J Biol Chem 2001;276:28799–805
- Bulawa CE, Connelly S, Devit M, Wang L, Weigel C, Fleming JA, Packman J, et al. Tafamidis, a potent and selective transthyretin kinetic stabilizer that inhibits the amyloid cascade. Proc Natl Acad Sci USA 2012;109:9629–34
- Ferreira N, Saraiva MJ, Almeida MR. Epigallocatechin-3-gallate as a potential therapeutic drug for TTR-related amyloidosis: “in vivo” evidence from FAP mice models. PLoS One 2012;7:e29933
- Ferreira N, Santos SA, Domingues MR, Saraiva MJ, Almeida MR. Dietary curcumin counteracts extracellular transthyretin deposition: Insights on the mechanism of amyloid inhibition. Biochim Biophys Acta 2013;1832:39–45
- Cardoso I, Saraiva MJ. Doxycycline disrupts transthyretin amyloid: evidence from studies in a FAP transgenic mice model. FASEB J 2006;20:234–9
- Kurosawa T, Igarashi S, Nishizawa M, Onodera O. Selective silencing of a mutant transthyretin allele by small interfering RNAs. Biochem Biophys Res Commun 2005;337:1012–8
- Alvarez R, Borland T, Chen Q, Milstein S, Nguyen T, Hinkle G, Kuchimanchi S, et al. ALN-TTR, an RNAi therapeutic for the treatment of transthyretin-mediated Amyloidosis [abstract]. Amyloid 2010;17:s1 51–2
- Cardoso I, Martins D, Ribeiro T, Merlini G, Saraiva MJ. Synergy of combined doxycycline/TUDCA treatment in lowering Transthyretin deposition and associated biomarkers: studies in FAP mouse models. J Transl Med 2010;8:74
- Obici L, Cortese A, Lozza A, Lucchetti J, Gobbi M, Palladini G. Doxycycline plus tauroursodeoxycholic acid for transthyretin amyloidosis: a phase II study. Amyloid 2012;19:34–6