Abstract
Aim: To determine whether normal human amnion-derived mesenchymal stromal cells (hAMSCs) secrete trophic mediators able to inhibit human prostate cancer cell lines growth.
Methods: Human prostate cancer and normal cell lines were used. Mesenchymal stromal cells (MSC) were isolated through mechanical and enzymatic digestion from amniotic membranes and were evaluated for specific mesenchymal stromal cells antigens. Cell proliferation was examined by MTT assay. Staining with propidium iodide (PI) followed by flow cytometry was used to detect cell cycle phase.
Results: hAMSC showed proper mesenchymal stem cells phenotype. We found that hAMSC conditioned media (CM) inhibited prostate cancer cells proliferation. Indeed, we demonstrated that hAMSC CM treatment increased percentage of G1 cancer cells and decreased percentage of cancer cells in S and G2M phases, suggesting that the hAMSC factors slow progression of prostate cancer cells through cell cycle inhibition.
Conclusions: Our study provide evidences that hAMSC microenvironment secretes soluble factors able to inhibit prostate cancer cells growth. This may represent a novel strategy to control proliferation of prostate cancer through modulation of the host microenvironment.
Introduction
Prostate cancer is the most commonly diagnosed tumor in western countries, representing the second leading cause of death [Citation1]. The main clinical problem in prostate cancer is the conversion of hormone-sensitive cancers to hormone-refractory disease after treatment with endocrine therapy [Citation2,Citation3]. Although prostate cancer detected at early stage can be successfully eradicated by radical prostatectomy and radiotherapy, no curative treatment exists for metastatic diseases [Citation4]. Indeed, development of more effective therapies has to be considered a priority of worldwide biomedical community. Human mesenchymal stem cells (MSCs) are multipotent adult stem cells, identified in human postnatal bone marrow (BM) and in muscle, periosteum and adipose tissue [Citation5–12]. MSC have unique immunomodulatory properties being able to inhibit inflammation and immunological responses both in vitro and in vivo [Citation13–20]. However, clinical use of BM-derived MSCs is limited for the relatively invasive procedure and a markedly reduction in cell number and differentiation capacity with age [Citation21–23]. Recently, different tissues of fetal origins like the placental membranes and cord blood have been studied as new sources of MSCs for their relative ease of collection and the much younger cellular age compared to adult tissues [Citation24–26]. MSCs have been isolated mainly from the placental basal plate [Citation27,Citation28] and amniotic membranes [Citation29]. The opportunity of having fetal tissues that are spontaneously expelled and usually discarded after delivery without ethical conflicts and characterized by high-yield in stem cell recovery, make amniotic membranes an attractive and exciting alternative source [Citation30]. The effects of mesenchymal stem cells (MSC) on human malignancies growth and metastatization are controversial [Citation31–34]. Several studies have shown enhancement of tumor growth and development, potentially through immunomodulatory and pro-angiogenic properties of MSCs, whereas others have shown tumor growth inhibition and extended survival [Citation35–37]. For example, it has been described that MSCs had potential inhibitory effects on tumor cell growth in vitro and in vivo by inducing cancer cells apoptosis [Citation36]. Importantly, it has been shown that MSC are able to inhibit metastasis of hepatocellular carcinoma (HCC) [Citation38,Citation39] and breast cancer cell proliferation [Citation40]. Moreover, MSCs exhibited potent antitumor effects in a model of Kaposi's sarcoma (KS), a highly inflammatory angiogenic tumor [Citation41]. Prostate cancer is a heterogeneous disease from both molecular and clinical points of view. As most carcinomas, it comprises various cell populations with marked differences in proliferation and differentiation abilities as well as different capacity to reconstitute the tumor upon transplantation. These observations lead to the hypothesis that tumor cells might arise from a subset of putative progenitors named cancer stem cells (CSC) [Citation42–45]. CSC were already isolated and characterized from different human malignancies [Citation46–51]. In the adult, stem cells reside in a physiologically limited and specialized microenvironment [Citation52,Citation53], that plays a pivotal role in preserving a constant pool of stem cells, thus ensuring tissue homeostasis [Citation54–59]. It is possible that cancer stem cells originate from mutations in progenitor cells that undergo uncontrolled proliferation [Citation54]. It has also been suggested that cancer stem progenitors may arise from a microenvironment similar to that of normal stem cells but shifted towards dominant growth promoting signals. Our hypothesis is that stem cells secret factors that acts in paracrine/autocrine way to control tissue hyperplasia and/or tumor formation. Cancer stem cell may have lost these molecules. In support of this hypothesis, we previously demonstrated that human embryonic stem cells were able to inhibit epithelial cancer cell proliferation through secreted molecules [Citation60]. The objective of this study was to determine if human amniotic derived stem cells (hAMSC) contain factors that inhibit growth of human prostate cancer cells.
Materials and methods
Materials
PC-3 and LNCaP cells were kindly provided by Dr Pandini G (Department of Clinical and Molecular Biomedicine, Endocrinology Unit, University of Catania, Italy) were derived from grade IV prostate adenocarcinoma and a brain lesion in patient with widespread metastatic prostate carcinoma respectively. PNT1A cells (Sigma, Milan, Italy) were derived from human post pubertal normal prostate. Culture medium and supplements were purchased from Gibco (Life Technologies, Italy). MTT (3,[4,5-dimethylthiazol-2-yl]-2,5-diphenyltetrazolium bromide), Propidium iodide (PI), RNAse type A and dimethyl sulfoxide (DMSO) were purchased from Sigma (Milan, Italy). All the AMSC antibodies were purchased from Myltenyi Biotec (Bologna, Italy).
Placental tissue collection and isolation of MSC from human amniotic membranes
Placental tissue was collected immediately after delivery from singleton physiological term pregnancies. Patients with diabetes, infections, kidney disease, congenital malformations and chromosomal anomalies (number and/or structure) were excluded. Placental membranes were excised from the placenta and amnion and chorion were separated. Cells were isolated through enzymatic digestion. Briefly, the amnion was mechanically minced and incubated with 100 U/ml collagenase type I (Gibco, Life Technologies, Italy) plus 5 µg/ml DNAse I (Life Technologies, Italy) for 2 h. Next, cells were washed and resuspended in Dulbecco's modified Minimum Essential Medium (DMEM) supplemented with 10% Fetal Bovine Serum (FBS Australian origin, Italy) and seeded in T150 flask. Importantly, the culture media was not supplemented with basic fibroblast growth factor (bFGF) in order to maintain the original biochemical and molecular features of the hAMSCs. The cultures were maintained at 37 °C and 5% CO2.
Cell culture conditions
Cancer cell lines as well as PNT1A cells were maintained in RPMI 1640 medium supplemented with 10% fetal bovine serum (FBS), l-glutamine (2 mM), penicillin (100 U/ml), streptomycin (100 µg/ml) and fungizone (250 µg/ml). hAMSC were cultured in DMEM supplemented with 10% fetal bovine serum and gentamycin. All cells were grown at 37 °C in a humidified incubator in an atmosphere of 5% CO2 in air.
hAMSC characterization
At passage 3, hAMSC were evaluated for specific mesenchymal stromal cells antigens by flow cytometry. The following markers were investigated: HLA-DR, CD105, CD166, CD90, CD34, CD73, CD133, CD20, CD326, CD31, CD45 and CD14. All the antibodies were purchased from Myltenyi Biotec (Myltenyi Biotec, Bologna, Italy). Moreover, Oct4 and Nanog mRNA expression levels were assessed in hAMSCs by semi-quantitative RT PCR in order to verify cellular stemness. Primers sequences were the following: Oct4 FW(5′-CGT GAA GCTG GAG AAG GAG AAG CTG-3′) RV(5′-CAA GGG CCG CAG CTT ACA CAT GTT C-3′); Nanog FW(5′-AAT ACC TCA GCC TCC AGC AGA TG-3′) RV(5′-CTG CGT CAC ACC ATT GCT ATT CT-3′).
Conditioned medium culture
Starting from culture passage 3, conditioned medium (CM) was produced by incubating hAMSC cells with DMEM medium for 3 days and 1 week. AMSC cells were grown on T150 flask with 25 ml of DMEM medium. CM was harvested and passed through a 0.2 μm syringe filter to remove cellular debris.
Cell proliferation assay
Cell proliferation was determined by MTT reduction method and by direct cell counts. Cells were plated at a density of 5 × 103 cells/well in 200 µl of growth medium in 96-well plates and at density of 70 × 103 or 10 × 103 cells/well in 2 ml of growth medium in 6-well plates . Twenty-four hours later, medium was removed and cells were incubated with different conditioned media as previously indicated. Next, 30 µl of 5 mg/ml MTT in phosphate buffered saline (PBS) solution were added to each well and cells were incubated for 4 h at 37 °C. Finally, medium was aspirated and DMSO was added to each well. Absorbance at 570 nM was determined by spectrophotometry. Also, direct cell counting was performed using a hemocytometer and trypan-blue dye. Cells were harvested using trypsin–EDTA and counted under light microscopy. Stained cells were also counted to indicate the amount of dead cells present.
DNA cell cycle analysis
Cells were plated at a density of 50 × 103 cells/well in 2 ml of growth medium in 6-well plates. Upon reaching of 50–60% confluence, cell cultures were synchronized by serum deprivation as follows. The medium was aspirated and cells were rinsed with PBS. Cells were then overlaid with 2 ml of 0.5% stripped serum in RPMI 1640 and incubated for 48 h. At the end of the synchronization process, the medium was replaced by DMEM unconditioned medium (control) and AMSCs CM p3 Day 3 and 1 week.
Cells were harvested into 15 ml tubes, centrifuged and washed with ice-cold PBS. Cells (1 × 106 per test) were fixed in 70% cold ethanol for 30–60 min at −20 °C, washed with cold PBS and resuspended in a PBS solution containing 50 µg/ml of PI and 100 U/ml of RNAse type A. Cells were then left to incubate in the dark at room temperature. After 30 min, flow cytometry was performed after gating to eliminate doublets and debris.
Statistical analysis
Results are reported as mean ± SEM. Data were analyzed by one-way analysis of variance (ANOVA) followed by the Bonferroni post hoc test using SPSS 18.0 for Windows (SPSS Inc., Chicago, IL). A statistically significant difference was accepted when the p value was lower than 0.05.
Results
Amniotic membrane derived stromal cells showed proper MSC antigens profile
hAMSC were analyzed for the expression of typical hAMSCs markers by flow cytometry. Purity of hAMSC population was determined by lack of hematopoietic and endothelial antigens HLAII, CD34, CD133, CD20, CD326, CD31, CD45, CD14 and the expression of mesenchymal cell-associated antigens CD105, CD166, CD90 and CD73. Moreover, in support of the hypothesis that hAMSC may display pluripotency these cells properly expressed both Oct4 and Nanog mRNA (data not shown).
Effects of hAMSC CM on prostate cancer cell proliferation
To determine whether hAMSC cells produce paracrine mediators that affect prostate cancer cells, we studied the effect of hAMSCs conditioned medium (AMSC CM) on LNCaP and PC3 cell culture growth. Since hAMSCs cells are grown in DMEM, the appropriate control for the hAMSCs CM was DMEM unconditioned medium. For the first set of experiments, RPMI medium + 10% serum was used as additional control. Since cell lines consistently grew as well in DMEM as in RPMI 1640, this control was discontinued. Conditioned medium was collected from hAMSCs cells at passage 3 after 3 days and 1 week of incubation. The medium from LNCaP and PC3 cells seeded into 96- or 6-well plates 24 h earlier was replaced with RPMI 1640, DMEM or DMEM + AMSC cell CM and MTT dye-reduction assay or hemocytometer counting were performed at 0, 24, 48 or 72 h.
hAMSCs CM inhibited proliferation of both LNCaP and PC3 cells as compared to DMEM and RPMI 1640 medium at 24–72 h. There were no differences between cells exposed to DMEM and RPMI 1640 (p > 0.05). Specifically, hAMSCs CM p3 Day 3 induced a significant inhibition of LNCaP proliferation after 48–72 h (p < 0.001, p < 0.01), while hAMSCs CM p3 1 week resulted in stronger inhibition that was evident as early as 24 h of culture (p < 0.01). Similar results were obtained for PC3 prostate cancer cells. This effect was observed by both MTT () and direct cell count () techniques. Regarding dead cells, no significant differences were observed among DMEM unconditioned medium and hAMSCs CM (data not shown).
Figure 1. Cell proliferation rate in LNCaP, PC3 and PNT1A cells treated by AMSCs Conditioned Media. Cell proliferation in LNCaP (A), PC3 (B) and PNT1A (C) cells treated by unconditioned DMEM, Day 3 or 1 week AMSCs Conditioned Media was determined by MTT assay at time 0, 24, 48 and 72 h of culture. Statistical significance has been considered as p < 0.05 (*).
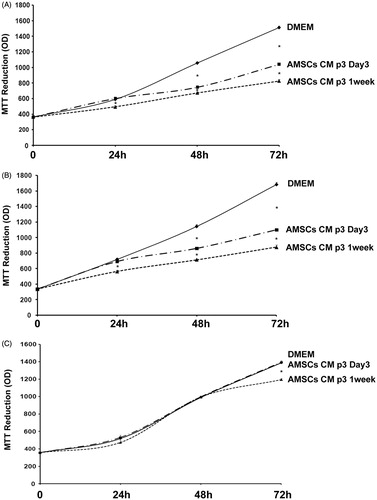
Figure 2. Cell proliferation in LNCaP, PC3 and PNT1A cells treated by AMSCs Conditioned Media as assessed by direct cell count and Trypan Blue exclusion. LNCaP (A), PC3 (B) and PNT1A (C) cell number where treated by unconditioned DMEM, Day 3 or 1 week AMSCs Conditioned Media at time 0, 24, 48 and 72 h of culture. Statistical significance has been considered as p < 0.05 (*).
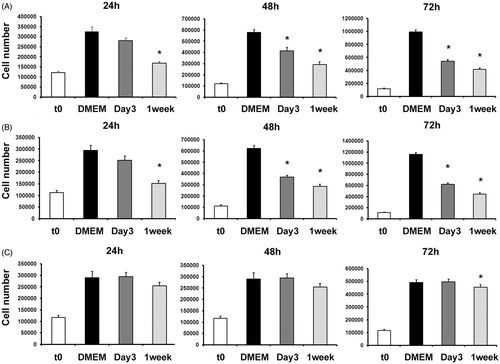
hAMSC CM did not affect normal prostate cells growth
We studied the ability of hAMSCs-CM to inhibit PNT1A normal human prostate cells growth. These cells were tested under the same conditions described above for cancer cells. Only hAMSC CM p3 1 week resulted in slightly decreased cell number of PNT1A (p < 0.01) and this effect was observed only after 72 h of culture as determined by both MTT () and direct cell count ().
Effect of hAMSC CM on prostate cancer cell cycle
We tested the effect of hAMSC CM on LNCaP and PC3 cell cycle progression. Cells were treated with hAMSC CM or unconditioned DMEM and stained with PI for FACS analysis. PI binds DNA and detects percentage of cells in each phase of the cell cycle.
We found increased LNCaP cell number in G1 phase (64.7 and 61.2%) when cell were treated by AMSC CM p3 Day3 and 1 week compared to cells treated with unconditioned DMEM (54.5%) and decreased cell number in S phase (11 and 12.5%) and in G2/M phase (15.7 and 17.7%) compared to control DMEM (S phase = 15.6%, G2/M = 22.2%; p < 0.05, ).
Figure 3. DNA Cell cycle progression in LNCaP cells treated by unconditioned DMEM (A left panel), Day 3 (B left panel) or 1 week (C left panel) AMSCs Conditioned Media as assessed by Propidium Iodide staining followed by Flow Cytometry. A, B and C right panels: representative plots of FACS analysis, E = G1 phase; F = G2/M phase; B = S phase.
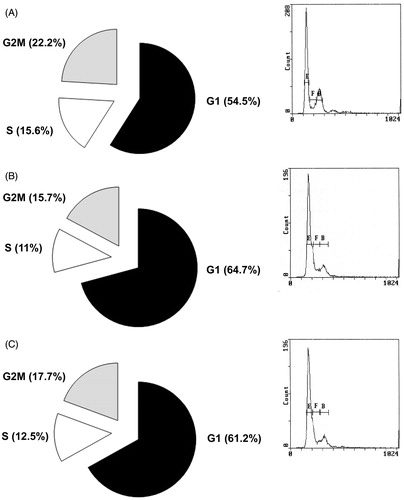
Finally, we observed an increased number of PC3 cells in G1 Phase (73.2% and 68.5%) when treated by AMSC CM p3 Day 3 and 1 week compared to cells treated with DMEM CM (54.2%), and decreased cell number in S phase (10.6 and 12.1%) and in G2/M phase (15.5 and 18%) compared to control DMEM (S phase = 16.5%, G2/M = 23.5%; p < 0.05; ).
Figure 4. DNA Cell cycle progression in PC3 cells treated by unconditioned DMEM (A left panel), Day 3 (B left panel) or 1 week (C left panel) AMSCs Conditioned Media as assessed by Propidium Iodide staining followed by Flow Cytometry. A, B and C right panels: representative plots of FACS analysis, E = G1 phase; F = G2/M phase; B = S phase.
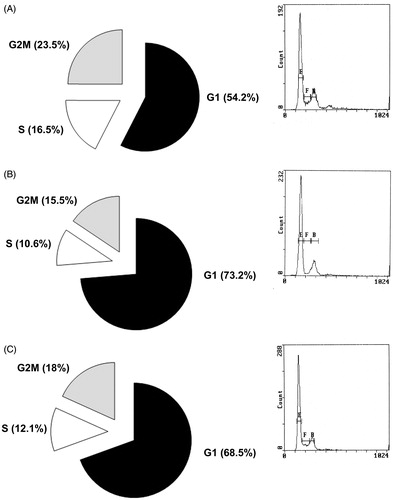
Discussion
Our study demonstrated that hAMSCs conditioned media inhibited prostate cancer cell proliferation, while little inhibitory effect was observed on normal human prostate cell lines. Amniotic membrane-derived MSC have functional characteristic comparable to those from adult bone marrow [Citation30,Citation61,Citation62]. Indeed, they express low levels of HLA-ABC, but not HLA-DR [Citation62,Citation63], suggesting that these cells are immunoprivileged [Citation64]. Moreover, we confirmed that hAMSCs expressed the Oct-4 mRNA. Noteworthy, Oct-4 transcript is found in totipotent embryonic stem cells, while it is down-regulated during cell differentiation [Citation30,Citation65,Citation66]. Therefore, amniotic membranes represent a remarkable and ethically acceptable alternative source of pluripotent mesenchymal cells [Citation30]. Nowadays, considerable attention is directed towards the niche and those molecular signals that control stem cells proliferation and differentiation. The availability of pharmacological compounds that target specific signaling pathways makes the molecular manipulation of stem cell niche an attractive alternative to cell therapy. Thus, it is fundamental to understand hAMSC microenvironment and its effect on prostate cancer cell lines.
In this study, we showed that soluble signals produced by hAMSC influenced cancer cell growth. We demonstrated the strongest effect on prostate cancer cell proliferation inhibition at 72 h. On the other hand, intriguing results were obtained showing only a slight inhibition of human PNT1A normal prostate cell proliferation when CM was used after 72 h of culture. Our data suggest that hAMSCs trophic mediators may not affect proliferation of non-malignant human cells. This observation must be confirmed by performing further investigations on other cell types.
It has been previously demonstrated that MSCs inhibited rat intracranial glioma cell growth [Citation67]. These results are consistent with reports by others [Citation68,Citation69] who demonstrated growth suppression of lung cancer cells by MSCs soluble factors. Likewise, there are evidences that human embryonic stem cells have an inhibitory effect on cancer cell proliferation [Citation60,Citation70].
Our findings open to the possibility of a new cell-free treatment based on hAMSC-derived soluble molecules. Instead of using cells themselves for therapy [Citation71,Citation72], it will likely be possible to use the hAMSC-CM, thereby by-passing the practical and ethical issues typical of cell therapies. Stem and cancer cells share several features, including loss of contact inhibition and immortality. Furthermore, they have similar signaling pathways that regulate self-renewal and differentiation [Citation73–75].
Tight regulation of cell cycle is pivotal to maintain proper balance between proliferation, differentiation and death. Cyclin dependent kinases (cdk) play a role as gatekeepers to prevent inappropriate cell proliferation [Citation76]. Cell cycle phases have checkpoints that regulate cell cycle progression or arrest. During G1 phase, mitogenic factors stimulate the expression of proto-oncogenes and, in turn, increased proliferation. These mitogenic pathways induce D-type cyclins that promote Cdk4/Cdk6 activity, followed by expression of E1 and E2 cyclins, that increase Cdk2 activity [Citation77]. These complexes lead to G1/S transition.
Inhibitory signals, such as oxidative stress and DNA damage, may influence G1/S transition and induce the expression of anti-proliferative molecules. Block of cell cycle progression might be at other checkpoints such as S and G2/M phases [Citation77]. During checkpoints, cells remain arrested until damage is repaired. Cancer cells arise when these checkpoints fail and damaged cells survive. The balance among mitogenic, anti-mitogenic, apoptotic and stress response signals determines cell fate. Restoring cell cycle checkpoints or blocking cancer cells in G1 will prevent cancer growth.
Our hypothesis is that stem cells could act in paracrine/autocrine ways to control cell cycle thus limiting tissue hyperplasia and tumor formation. Cancer stem cells may have lost these abilities. Thus, we investigated cell cycle in prostate cancer cells treated by hAMSC conditioned medium. We found increased percentage of cells in G1, accompanied by decrease of S and G2M phase cells compared to controls. Indeed, our data suggest that CM blocked cancer cells progression through cell cycle, resulting in prostate cancer cell proliferation inhibition.
In conclusion, in this study, we demonstrated that human amniotic stromal cells produce soluble factors able to block prostate cancer proliferation by slowing cell cycle progression. Our results suggest the opportunity to exploit hAMSCs biology to treat human epithelial malignancies.
Declaration of interest
The authors declare that they have no conflict of interest. The authors alone are responsible for the content and writing of this article. Dr Giuffrida Domenica is supported by Fondazione Umberto Veronesi (Italy).
Acknowledgements
The authors acknowledge Dr Bonanno Oriana and Dr Gili Elisa, University of Catania, for their contribution in flow cytometry analysis.
References
- Frydenberg M, Stricker PD, Kaye KW. Prostate cancer diagnosis and management. Lancet 1997;349:1681–7
- Bentel JM, Tilley WD. Androgen receptors in prostate cancer. J Endocrinol 1996;151:1–11
- Small EJ, Vogelzang NJ. Second-line hormonal therapy for advanced prostate cancer: a shifting paradigm. J Clin Oncol 1997;15:382–8
- van der Horst G, van den Hoogen C, Buijs JT, et al. Targeting of alpha(v)-integrins in stem/progenitor cells and supportive microenvironment impairs bone metastasis in human prostate cancer. Neoplasia 2011;13:516–25
- Nakahara H, Dennis JE, Bruder SP, et al. In vitro differentiation of bone and hypertrophic cartilage from periosteal-derived cells. Exp Cell Res 1991;195:492–503
- Zuk PA, Zhu M, Mizuno H, et al. Multilineage cells from human adipose tissue: implications for cell-based therapies. Tissue Eng 2001;7:211–28
- Jankowski RJ, Deasy BM, Huard J. Muscle-derived stem cells. Gene Ther 2002;9:642–7
- Young HE, Steele TA, Bray RA, et al. Human reserve pluripotent mesenchymal stem cells are present in the connective tissues of skeletal muscle and dermis derived from fetal, adult, and geriatric donors. Anat Rec 2001;264:51–62
- Pittenger MF, Mackay AM, Beck SC, et al. Multilineage potential of adult human mesenchymal stem cells. Science 1999;284:143–7
- Zuk PA, Zhu M, Ashjian P, et al. Human adipose tissue is a source of multipotent stem cells. Mol Biol Cell 2002;13:4279–95
- Zvaifler NJ, Marinova-Mutafchieva L, Adams G, et al. Mesenchymal precursor cells in the blood of normal individuals. Arthritis Res 2000;2:477–88
- Nathanson MA. Bone matrix-directed chondrogenesis of muscle in vitro. Clin Orthop Relat Res 1985:142–58
- Bartholomew A, Sturgeon C, Siatskas M, et al. Mesenchymal stem cells suppress lymphocyte proliferation in vitro and prolong skin graft survival in vivo. Exp Hematol 2002;30:42–8
- Uccelli A, Moretta L, Pistoia V. Immunoregulatory function of mesenchymal stem cells. Eur J Immunol 2006;36:2566–73
- Miyahara Y, Nagaya N, Kataoka M, et al. Monolayered mesenchymal stem cells repair scarred myocardium after myocardial infarction. Nat Med 2006;12:459–65
- Abdel-Latif A, Bolli R, Tleyjeh IM, et al. Adult bone marrow-derived cells for cardiac repair: a systematic review and meta-analysis. Arch Intern Med 2007;167:989–97
- Nagaya N, Kangawa K, Itoh T, et al. Transplantation of mesenchymal stem cells improves cardiac function in a rat model of dilated cardiomyopathy. Circulation 2005;112:1128–35
- Ohnishi S, Yanagawa B, Tanaka K, et al. Transplantation of mesenchymal stem cells attenuates myocardial injury and dysfunction in a rat model of acute myocarditis. J Mol Cell Cardiol 2007;42:88–97
- Rojas M, Xu J, Woods CR, et al. Bone marrow-derived mesenchymal stem cells in repair of the injured lung. Am J Respir Cell Mol Biol 2005;33:145–52
- Abdel Aziz MT, Atta HM, Mahfouz S, et al. Therapeutic potential of bone marrow-derived mesenchymal stem cells on experimental liver fibrosis. Clin Biochem 2007;40:893–9
- Panepucci RA, Siufi JL, Silva WAJr, et al. Comparison of gene expression of umbilical cord vein and bone marrow-derived mesenchymal stem cells. Stem Cells 2004;22:1263–78
- Caplan AI. The mesengenic process. Clin Plast Surg 1994;21:429–35
- D'Ippolito G, Schiller PC, Ricordi C, et al. Age-related osteogenic potential of mesenchymal stromal stem cells from human vertebral bone marrow. J Bone Miner Res 1999;14:1115–22
- Soncini M, Vertua E, Gibelli L, et al. Isolation and characterization of mesenchymal cells from human fetal membranes. J Tissue Eng Regen Med 2007;1:296–305
- Campagnoli C, Roberts IA, Kumar S, et al. Identification of mesenchymal stem/progenitor cells in human first-trimester fetal blood, liver, and bone marrow. Blood 2001;98:2396–402
- in 't Anker PS, Noort WA, Scherjon SA, et al. Mesenchymal stem cells in human second-trimester bone marrow, liver, lung, and spleen exhibit a similar immunophenotype but a heterogeneous multilineage differentiation potential. Haematologica 2003;88:845–52
- Zhang X, Mitsuru A, Igura K, et al. Mesenchymal progenitor cells derived from chorionic villi of human placenta for cartilage tissue engineering. Biochem Biophys Res Commun 2006;340:944–52
- Rolfo A, Giuffrida D, Nuzzo AM, et al. Pro-inflammatory profile of preeclamptic placental mesenchymal stromal cells: new insights into the etiopathogenesis of preeclampsia. PLoS One 2013;8:e59403
- Bailo M, Soncini M, Vertua E, et al. Engraftment potential of human amnion and chorion cells derived from term placenta. Transplantation 2004;78:1439–48
- Alviano F, Fossati V, Marchionni C, et al. Term amniotic membrane is a high throughput source for multipotent mesenchymal stem cells with the ability to differentiate into endothelial cells in vitro. BMC Dev Biol 2007;7:11
- Studeny M, Marini FC, Dembinski JL, et al. Mesenchymal stem cells: potential precursors for tumor stroma and targeted-delivery vehicles for anticancer agents. J Natl Cancer Inst 2004;96:1593–603
- Fierro FA, Sierralta WD, Epunan MJ, Minguell JJ. Marrow-derived mesenchymal stem cells: role in epithelial tumor cell determination. Clin Exp Metastasis 2004;21:313–9
- Ohlsson LB, Varas L, Kjellman C, et al. Mesenchymal progenitor cell-mediated inhibition of tumor growth in vivo and in vitro in gelatin matrix. Exp Mol Pathol 2003;75:248–55
- Zhu Y, Sun Z, Han Q, et al. Human mesenchymal stem cells inhibit cancer cell proliferation by secreting dkk-1. Leukemia 2009;23:925–33
- Karnoub AE, Dash AB, Vo AP, et al. Mesenchymal stem cells within tumour stroma promote breast cancer metastasis. Nature 2007;449:557–63
- Lu YR, Yuan Y, Wang XJ, et al. The growth inhibitory effect of mesenchymal stem cells on tumor cells in vitro and in vivo. Cancer Biol Ther 2008;7:245–51
- Yu JM, Jun ES, Bae YC, Jung JS. Mesenchymal stem cells derived from human adipose tissues favor tumor cell growth in vivo. Stem Cells Dev 2008;17:463–73
- Li GC, Ye QH, Xue YH, et al. Human mesenchymal stem cells inhibit metastasis of a hepatocellular carcinoma model using the mhcc97-h cell line. Cancer Sci 2010;101:2546–53
- Livraghi T, Meloni F, Frosi A, et al. Treatment with stem cell differentiation stage factors of intermediate-advanced hepatocellular carcinoma: an open randomized clinical trial. Oncol Res 2005;15:399–408
- Qiao L, Zhao TJ, Wang FZ, et al. Nf-kappab downregulation may be involved the depression of tumor cell proliferation mediated by human mesenchymal stem cells. Acta Pharmacol Sin 2008;29:333–40
- Khakoo AY, Pati S, Anderson SA, et al. Human mesenchymal stem cells exert potent antitumorigenic effects in a model of kaposi's sarcoma. J Exp Med 2006;203:1235–47
- Li H, Chen X, Calhoun-Davis T, et al. Pc3 human prostate carcinoma cell holoclones contain self-renewing tumor-initiating cells. Cancer Res 2008;68:1820–5
- Collins AT, Berry PA, Hyde C, et al. Prospective identification of tumorigenic prostate cancer stem cells. Cancer Res 2005;65:10946–51
- Guzman-Ramirez N, Voller M, Wetterwald A, et al. In vitro propagation and characterization of neoplastic stem/progenitor-like cells from human prostate cancer tissue. Prostate 2009;69:1683–93
- Clarke MF, Fuller M. Stem cells and cancer: two faces of eve. Cell 2006;124:1111–5
- Lapidot T, Sirard C, Vormoor J, et al. A cell initiating human acute myeloid leukaemia after transplantation into scid mice. Nature 1994;367:645–8
- Al-Hajj M, Wicha MS, Benito-Hernandez A, et al. Prospective identification of tumorigenic breast cancer cells. Proc Natl Acad Sci USA 2003;100:3983–8
- Singh SK, Clarke ID, Terasaki M, et al. Identification of a cancer stem cell in human brain tumors. Cancer Res 2003;63:5821–8
- Lee CJ, Dosch J, Simeone DM. Pancreatic cancer stem cells. J Clin Oncol 2008;26:2806–12
- Ricci-Vitiani L, Lombardi DG, Pilozzi E, et al. Identification and expansion of human colon-cancer-initiating cells. Nature 2007;445:111–5
- Kelly K, Yin JJ. Prostate cancer and metastasis initiating stem cells. Cell Res 2008;18:528–37
- Schofield R. The relationship between the spleen colony-forming cell and the haemopoietic stem cell. Blood Cells 1978;4:7–25
- Li L, Xie T. Stem cell niche: structure and function. Annu Rev Cell Dev Biol 2005;21:605–31
- Li L, Neaves WB. Normal stem cells and cancer stem cells: the niche matters. Cancer Res 2006;66:4553–7
- Tumbar T, Guasch G, Greco V, et al. Defining the epithelial stem cell niche in skin. Science 2004;303:359–63
- Zhang J, Niu C, Ye L, et al. Identification of the haematopoietic stem cell niche and control of the niche size. Nature 2003;425:836–41
- Arai F, Hirao A, Ohmura M, et al. Tie2/angiopoietin-1 signaling regulates hematopoietic stem cell quiescence in the bone marrow niche. Cell 2004;118:149–61
- Potten CS, Owen G, Booth D. Intestinal stem cells protect their genome by selective segregation of template DNA strands. J Cell Sci 2002;115:2381–8
- Cotsarelis G, Sun TT, Lavker RM. Label-retaining cells reside in the bulge area of pilosebaceous unit: implications for follicular stem cells, hair cycle, and skin carcinogenesis. Cell 1990;61:1329–37
- Giuffrida D, Rogers IM, Nagy A, et al. Human embryonic stem cells secrete soluble factors that inhibit cancer cell growth. Cell Prolif 2009;42:788–98
- In 't Anker PS, Scherjon SA, Kleijburg-van der Keur C, et al. Isolation of mesenchymal stem cells of fetal or maternal origin from human placenta. Stem Cells 2004;22:1338–45
- Portmann-Lanz CB, Schoeberlein A, Huber A, et al. Placental mesenchymal stem cells as potential autologous graft for pre- and perinatal neuroregeneration. Am J Obstet Gynecol 2006;194:664–73
- Wolbank S, Peterbauer A, Fahrner M, et al. Dose-dependent immunomodulatory effect of human stem cells from amniotic membrane: a comparison with human mesenchymal stem cells from adipose tissue. Tissue Eng 2007;13:1173–83
- Evangelista M, Soncini M, Parolini O. Placenta-derived stem cells: new hope for cell therapy? Cytotechnology 2008;58:33–42
- Loh YH, Wu Q, Chew JL, et al. The oct4 and nanog transcription network regulates pluripotency in mouse embryonic stem cells. Nat Genet 2006;38:431–40
- Zhao P, Ise H, Hongo M, et al. Human amniotic mesenchymal cells have some characteristics of cardiomyocytes. Transplantation 2005;79:528–35
- Nakamura K, Ito Y, Kawano Y, et al. Antitumor effect of genetically engineered mesenchymal stem cells in a rat glioma model. Gene Ther 2004;11:1155–64
- Hombauer H, Minguell JJ. Selective interactions between epithelial tumour cells and bone marrow mesenchymal stem cells. Br J Cancer 2000;82:1290–6
- Maestroni GJ, Hertens E, Galli P. Factor(s) from nonmacrophage bone marrow stromal cells inhibit lewis lung carcinoma and b16 melanoma growth in mice. Cell Mol Life Sci 1999;55:663–7
- Postovit LM, Margaryan NV, Seftor EA, et al. Human embryonic stem cell microenvironment suppresses the tumorigenic phenotype of aggressive cancer cells. Proc Natl Acad Sci USA 2008;105:4329–34
- Ohnishi S, Sumiyoshi H, Kitamura S, Nagaya N. Mesenchymal stem cells attenuate cardiac fibroblast proliferation and collagen synthesis through paracrine actions. FEBS Lett 2007;581:3961–6
- Caplan AI, Dennis JE. Mesenchymal stem cells as trophic mediators. J Cell Biochem 2006;98:1076–84
- Reya T, Clevers H. Wnt signalling in stem cells and cancer. Nature 2005;434:843–50
- Varga AC, Wrana JL. The disparate role of bmp in stem cell biology. Oncogene 2005;24:5713–21
- Androutsellis-Theotokis A, Leker RR, Soldner F, et al. Notch signalling regulates stem cell numbers in vitro and in vivo. Nature 2006;442:823–6
- Morgan DO. Cyclin-dependent kinases: engines, clocks, and microprocessors. Annu Rev Cell Dev Biol 1997;13:261–91
- Berthet C, Kaldis P. Cell-specific responses to loss of cyclin-dependent kinases. Oncogene 2007;26:4469–77