Abstract
Objective: This study aimed to determine the effects of orchidectomy and supraphysiological testosterone replacement on trabecular structure and gene expression in the bone.
Methods: Twenty-four 3-month old male rats were randomized into sham (SH), orchidectomized (ORX) and testosterone-treated (TE) groups. Orchidectomy was performed on the ORX and TE group. Weekly testosterone enanthate intramuscular injection at 7 mg/kg body weight was administered to the TE group for 8 weeks while the other groups received peanut oil as vehicle. Blood was collected before and after treatment for serum testosterone analysis. The femora and tibiae were harvested after the treatment period for trabecular structure and gene expression analysis.
Results: The trabecular bone volume decreased significantly and the porosity increased significantly in the ORX group compared to the SH group (p < 0.05). Testosterone treatment prevented all these changes (p < 0.05). The expression of osteogenic genes decreased significantly in the ORX group compared to the SH group (p < 0.05). Testosterone treatment decreased the expressions of RANKL and OPG genes significantly (p < 0.05).
Conclusion: Orchidectomy-induced degeneration in trabecular structure is caused by a decrease in the expressions of osteogenic genes. Supraphysiological testosterone replacement is able to prevent these degenerative changes in the bone despite the modest changes in gene expression.
Introduction
Male osteoporosis is a significant healthcare problem and economic burden to the society [Citation1,Citation2]. Testosterone deficiency either due to late-onset hypogonadism or androgen deprivation therapy is the major cause of male osteoporosis [Citation3,Citation4]. Testosterone deficiency due to orchidectomy has been found to cause degenerative changes in trabecular structure in animal models of osteoporosis [Citation5,Citation6]. Thus, the orchidectomized rat model has been used as a standard male hypogonadal osteoporosis model in the screening of anti-osteoporotic agents [Citation7]. However, the underlying mechanism of osteoporosis due to testosterone deficiency has not been well established. The available studies have reported either high bone remodeling indicated by high bone formation and high bone resorption rate [Citation8,Citation9], or an imbalance in bone remodeling indicated by increased bone resorption per se in the orchidectomized animals [Citation10,Citation11].
The issue above could be resolved by determining the difference in the expression of osteogenic and osteoclastic genes in the bone between normal and orchidectomized animals. Previous in vitro studies have demonstrated that androgen promotes the differentiation and mineralization of osteoblasts [Citation12,Citation13]. It can also suppress osteoclastogenesis induced by receptor activator of nuclear factor kappa-B ligand (RANKL) and macrophage colony-stimulating factor (MCSF) [Citation14]. However, there are limited in vivo studies on gene expression changes in the bone due to testosterone deficiency.
A meta-analysis by Tracz et al. has indicated that testosterone replacement only produces modest improvement in lumbar bone mineral density [Citation15]. Thus, supraphysiological testosterone treatment may produce better effects. In fact, supraphysiological doses of testosterone have been reported to prevent degenerative changes in the trabecular bone structure in rats [Citation16,Citation17]. In comparison, testosterone replacement at physiological doses only modestly improves bone structure in rats [Citation18]. There is no clear evidence on the skeletal effects of high dose testosterone in humans. The modulation of gene expression in the bone by a supraphysiological testosterone dose has not been quantified.
This study aimed to determine the effects of orchidectomy and testosterone replacement at supraphysiological dose on structural changes and gene expression in trabecular bone. We hypothesized that orchidectomy would cause degenerative changes in trabecular bone structure associated with decreased osteogenic gene expression and increased osteoclastic gene expression. We also speculated that supraphysiological testosterone replacement would prevent these changes within 8 weeks. The information garnered from this study will provide insight on the mechanism of bone loss in an orchidectomized rat model and enable better application of this model in the understanding of the pathophysiology of osteoporosis due to testosterone deficiency, as well as in the screening of anti-osteoporotic agents.
Materials and methods
Animal treatment
Male Sprague–Dawley rats (n = 24) aged 3 months old and weighted 250–300 g were procured from the Animal Research Unit of Universiti Kebangsaan Malaysia. The rats were housed in a vivarium located at the Pharmacology Department, Universiti Kebangsaan Malaysia under standard conditions [room temperature; natural light/dark cycle; tap water and standard rat diet (Gold Coin, Klang, Selangor) ad libitum]. They underwent 7 d of acclimatization before being put through any treatment. They were randomized into three groups, i.e. sham (SH), orchidectomized (ORX) and testosterone enanthate-treated (TE) group. Bilateral orchidectomy was performed on the ORX and TE group under anesthesia (Ketapex:Xylazil 1:1). The SH group underwent the same surgical procedure but their testes were not removed. Treatment commenced a week after the surgery to allow the wound to heal. The TE group received intramuscular injection of testosterone enanthate (Jesalis Pharma, Jena, Germany) at 7 mg/kg body weight weekly while the other two groups received peanut oil as the vehicle. Blood was collected via the orbital sinus before and after treatment, and serum was extracted for measurement of testosterone level. After 8 weeks of treatment, the rats were sacrificed and their femur was extracted and stored in −70 °C for gene expression analysis. Their tibiae was extracted and stored in formalin for X-ray microtomography. The research protocol had been reviewed and approved by the Animal Ethics Committee, Universiti Kebangsaan Malaysia (Code: FP/FAR/2012/IMA/18-JULY/445-JULY-2012-APRIL-2014).
Testosterone level
Serum testosterone level was measured using enzyme-linked immunosorbent assay (Cusabio, Wuhan, China).
X-ray microtomography (uCT)
The trabecular structure at the proximal tibia of the rats was scanned using a μCT 40 scanner (Scanco Medical, Brüttisellen, Switzerland). The images were obtained at 70 kVp and 114 μA with high resolution. The integration time was set at 200 ms. Two hundred slices starting from 1.5 mm under the lowest point of the proximal growth plate of the tibia were selected as the volume of interest. After the scan, the trabecular region of the bone was selected using a semi-automated method. Reconstruction and evaluation of the trabecular bone were conducted using the Scanco software (Scanco Medical, Brüttisellen, Switzerland). The structural indices obtained were bone volume (BV/TV), structural model index (SMI), connectivity density (Conn.D.), trabecular number (Tb.N.), trabecular thickness (Tb.Th.) and trabecular separation (Tb.Sp.).
Gene expression analysis
The expression of osteogenic and osteoclastic genes in the distal femur was quantified using QuantiGene Plex 2.0® technique (Panomics/Affymetrix, Santa Clara, CA). The genes of interest with their accession numbers were listed in . The oligonucleotide capture probes for mRNA were designed by the manufacturer. The metaphysical region of the distal femur was extracted and homogenized using a high-speed homogenizer (OMNI Bead Ruptor 24, Kennesaw, GA) and steel beads at 4 °C. The tissue lysate was digested at 65 °C to release the mRNA and centrifuged to precipitate the debris. The supernatant of the tissue lysate was loaded into a 96-well plate with capture reagent and probe set. Hybridization with pre-amplifier, amplifier and biotinylated label was performed after an overnight incubation at 54 °C. Luminescence proportional to mRNA captured by the bead was measured using a Luminex analyser (Bio-Rad, Hercules, CA) and it was converted into mean fluorescence intensity (MFI) specific for each gene. The expressions of these genes were normalized with the gene expression of beta-glucuronidase.
Table 1. Genes of interest in the current study.
Statistical analysis
Statistical Package for Social Sciences version 20 (IBM, Armonk, NY) was used to perform the statistical analysis. The normality of the data was examined using the Shapiro–Wilk test. All data were normal and further analysis was conducted using parametric tests. The comparisons of bone structural indices and gene expression levels among the three study groups were performed using one-way analysis of variance (ANOVA) with post-hoc pairwise comparison. The comparison of serum testosterone level before and after treatment among the study groups was performed using mixed designed ANOVA. The data were expressed in mean and standard error of mean (SEM). Statistical significance was set at p < 0.05.
Results
Mixed design ANOVA indicated that serum testosterone level of the rats differed significantly after 8 weeks of treatment (time effect p < 0.05). There was a significant interaction between time and group effect on testosterone level in the rats (interaction effect p < 0.05). Testosterone level in the TE group increased significantly (p < 0.05) while the level in the ORX group decreased significantly (p < 0.05) after 8 weeks. On the other hand, testosterone level in the SH group did not change significantly after 8 weeks (p > 0.05). Testosterone level was significantly higher in the SH group compared to the ORX group, and was significantly higher in the TE group compared to the ORX and SH group after 8 weeks of treatment (p < 0.05) ().
Figure 1. Serum testosterone level of the study groups. Orchidectomy was performed at week 0 and all rats were sacrificed at week 8. Testosterone enanthate at 7 mg/kg body weight was administered to the TE group for 8 weeks. Testosterone level decreased with orchidectomy and increased with testosterone enanthate treatment. #Indicates significant difference (p < 0.05) versus week 0 within the treatment group. *Indicates significant difference (p < 0.05) versus the SH group at week 8.
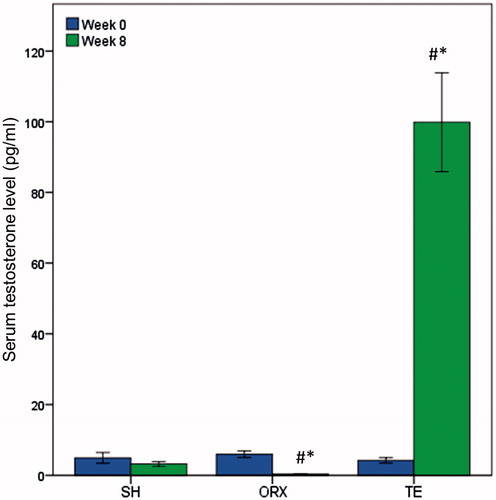
Orchidectomy induced significant reduction in BV/TV, Conn.D. and Tb.N., and a significant increase in Tb.Sp. at the proximal tibia of the ORX group compared to the SH group (p < 0.05). Treatment with testosterone enanthate prevented these degenerative changes on the trabecular bone in the rats (p < 0.05). The differences in SMI and Tb.Th. were not significantly different among the three groups (p > 0.05) (; ).
Figure 2. The trabecular structure at the proximal tibia of the rats in each study group. The image was obtained after 8 weeks of treatment and reconstructed using a μCT device. The trabecular structure of the ORX group was more porous compared to the SH and TE group.
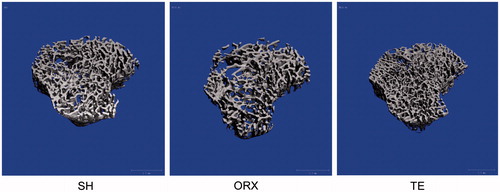
Table 2. Trabecular structural indices of the study group.
Orchidectomy also caused significant reduction in the expression of osteogenic genes, such as ALPL, BGLAP and COL1a1 compared to the SH group (p < 0.05). The expression of osteoclastic genes, such as ACP5, CTSK and ITGB3, as well as RANKL and OPG, were not affected by orchidectomy (p > 0.05). Treatment with testosterone enanthate decreased the expression of OPG gene significantly compared to the SH and ORX groups (p < 0.05), and the expression of RANKL compared to the ORX group (p < 0.05). The expression of osteogenic genes increased marginally but not significantly in the TE group compared to the ORX group ().
Figure 3. The gene expression of the study groups. Gene expression was measured from sample extracted from the metaphysial region of distal tibia using multiplex quantigene technique. The mRNA expression of the target gene was normalized with the mRNA expression of beta-glucuronidase. Orchidectomy decreased the expression of osteogenic genes significantly (p < 0.05). Testosterone treatment significantly decreased the gene expressions of OPG and RANKL (p < 0.05). Letter ‘a’ indicates significant difference (p < 0.05) versus the SH group; ‘b’ indicates significant difference (p < 0.05) versus the ORX group.
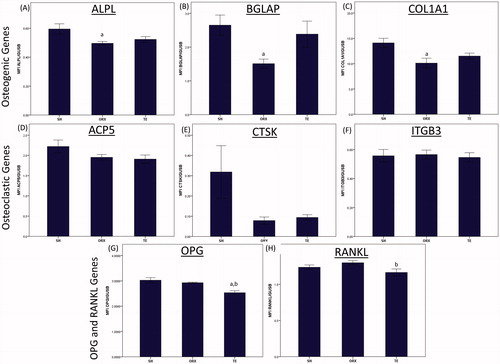
Discussion
The current study demonstrated that orchidectomy produced a significant deterioration in trabecular bone structure in the rats, which might be caused by decreased expression of osteogenic genes. No significant changes in the expression of osteoclastic genes, as well as RANKL and OPG genes were observed with orchidectomy. Intervention with a supraphysiological dose of testosterone enanthate successfully prevented the degeneration in trabecular bone structure. Testosterone enanthate also caused a significant decrease in the expression of RANKL and OPG genes. Taken together, supraphysiological dose of testosterone enanthate could prevent osteoporosis due to testosterone deficiency, but its effects on gene expression related to bone remodeling was transient.
In the study by Yarrow et al., orchidectomy reduced serum testosterone of young rats by 99% and weekly testosterone enanthate administration at 7 mg/kg for 1 month increased serum testosterone level 12.5-folds compared to the sham group [Citation16]. The current study found a larger fold of increment (30.1-fold, TE versus SH group after 8 weeks) probably because the treatment period was doubled in this study. Testosterone enanthate displayed delayed-releasing property, permitting it to stay in the circulation for a prolonged period [Citation19]. Weekly administration of testosterone enanthate allowed the serum testosterone level to build up continuously, reaching a supraphysiological level.
The effects of orchidectomy and supraphysiological testosterone administration on three aspects of trabecular bone structure, i.e. bone amount (BV/TV and Tb.N.), bone shape (SMI and Tb.N.) and porosity (Conn.D. and Tb.Sp) were studied. Orchidectomy posed significant adverse effects on bone amount and porosity but not on bone shape. Similar observation was obtained by Libouban et al. [Citation6] in orchidectomized young rats, whereby they demonstrated that BV/TV, Tb.N. and Tb.Sp. were affected significantly as early as 4 weeks post-orchidectomy but Tb.Th. was not changed after 16 weeks. Supraphysiological testosterone administration prevented these changes but did not improve any trabecular bone indices better than the normal control. The anti-osteoporotic effects of supraphysiological dose of testosterone had been demonstrated in rats [Citation16,Citation17]. In comparison, treatment with a physiological dose of testosterone only increased trabecular bone structural indices marginally in rats [Citation18]. In one study, testosterone enanthate treatment at 7 mg/kg for a shorter duration of time (6 weeks) only improved bone structural indices marginally [Citation20].
This study indicated that osteoporosis induced by orchidectomy was caused predominantly by a decrease in osteogenic gene expression. In contrast to this study, Soung et al. [Citation21] showed that orchidectomy caused increased osteogenic gene expression probably due to increased bone remodeling. This difference might be caused by the age of the rats, in which young (3-month old) rats were used in this study but aged (13-month old) rats were used in their study. Using tartrate-resistant acid phosphatase gene expression as a measure of bone resorption, Soung et al. [Citation21] showed that the effect of orchidectomy on bone resorption gene was merely marginal, which was similar to the present study. Falahati-Nini et al. [Citation22] investigated the distinct effects of testosterone and estradiol on bone remodeling in men with suppressed sex hormone production and they found that testosterone exerted significant effects on bone formation but limited effects on bone resorption compared to estradiol. This was supported by an in vitro study, showing that testosterone treatment only affected two osteoclastic genes while estradiol treatment affected six osteoclastic genes in monocyte-derived osteoclasts donated by men [Citation23]. Hence, we suggest that osteoporosis due to testosterone deficiency in young rats was due to an imbalance in bone remodeling process, whereby reduced bone formation in combination with preserved bone resorption lead to a net bone loss, indicated by degenerative structural change in the trabecular bone.
The gene expression of RANKL was marginally elevated in orchidectomized rats in this study. This finding was in accord with the previous studies in orchidectomized rats [Citation8]. Proell et al. [Citation8] demonstrated the marginally increased in RANKL mRNA expression could translate to a significant increase in soluble RANKL protein level in bone marrow of orchidectomized rats. The expression of OPG gene did not change significantly with orchidectomy in this study. This is different from the observation by Shuid et al. [Citation11], whereby they found decreased OPG level in the orchidectomized rats. In other studies, the expression of OPG and RANKL genes were found to be higher in orchidectomized rats [Citation24], which coincided with increased serum OPG and RANKL levels in hypogonadal men [Citation25]. The difference could be attributed to the age of the animal, whereby changes in OPG and RANKL expression in young rats used in this study were transient compared to aged rats.
Testosterone enanthate treatment did not change the expressions of most genes related to bone remodeling. The expression of RANKL and OPG genes decreased significantly in the TE group. Previous studies had reported decreased RANKL and OPG levels with testosterone treatment [Citation26,Citation27]. However, the decrease in RANKL gene expression was not accompanied by a decrease in osteoclastic gene expression in this study. This may be due to a decreased level of OPG in the TE group. Hence, the net stimulation for osteoclastogenesis remained constant. Although the increase in OPG level in hypogonadal men was speculated to be a physiological response to the concurrent increase in RANKL level [Citation25], the suppression of OPG with testosterone administration could occur independently of RANKL. An in vitro study showed that OPG protein and mRNA level decreased dose-dependently with testosterone treatment [Citation28]. This might be the reason testosterone was not an effective anti-resorptive agent as compared to estrogen.
There is a limited human study on the skeletal effects of supraphysiological testosterone administration. One issue of concern is the effect of high circulating testosterone level on the prostate. An animal study did find that supraphysiological dose of testosterone could cause prostate hypertrophy. Co-administration of a 5α-reductase inhibitor with supraphysiological testosterone was found to mitigate this side-effect while retaining the anabolic effects of testosterone on the skeletal system [Citation17,Citation29]. However, more studies should be conducted to ensure that this is safe to be applied in humans.
Several improvements could be made to improve this study. The protein expression of the gene studied could be quantified in the bone to validate the findings on gene expression. The bone remodeling markers in the serum could be determined to ascertain the effects of hypogonadism and supraphysiological testosterone administration on bone remodeling. The serum level of estrogen could be determined because testosterone could be converted to estrogen via aromatase enzyme.
Nonetheless, this study is novel because it clarified the underlying mechanism of osteoporosis induced by testosterone deficiency by quantifying the expression level of osteogenic and osteoclastic genes. It also demonstrated that the marginal increase in osteogenic gene expression induced by supraphysiological testosterone replacement can be translated to significant improvements in trabecular bone structure. It also showed that the orchidectomized rat is a suitable model for male osteoporosis due to testosterone deficiency and testosterone enanthate at supraphysiological dose can be a suitable positive control in the screening of potential anti-osteoporotic agents.
Conclusion
In conclusion, testosterone deficiency due to orchidectomy induces adverse changes in trabecular bone structure. This effect may be mediated via decreased expression of bone osteogenic genes. Testosterone at supraphysiological dose can prevent these osteoporotic changes despite the modest changes in gene expression. The orchidectomized young rat model can be utilized as a model for male osteoporosis for the screening of anti-osteoporotic agents.
Acknowledgements
We thank Dr. Thuan Bui (i-DNA Biotechnology Sdn. Bhd.) for his assistance in QuantiGene 2.0® procedure and Dr. Lim Fei Tieng (Hi-Tech Instruments Sdn. Bhd.) for her assistance in micro-CT measurement. We also thank laboratory technologists from Pharmacology Department for their kind assistance.
Declaration of interest
The authors declared no conflict of interest. This work is supported by Impak Perdana Grant (DIP-2012-07), Universiti Kebangsaan Malaysia. Chin K.Y. performed the experiment, analyzed the results and drafted the article. Ima-Nirwana S. supervised the project and provided critical review on the article.
References
- Burge R, Dawson-Hughes B, Solomon DH, et al. Incidence and economic burden of osteoporosis-related fractures in the United States, 2005-2025. J Bone Miner Res 2007;22:465–75
- Johnell O, Kanis JA. An estimate of the worldwide prevalence and disability associated with osteoporotic fractures. Osteoporos Int 2006;17:1726–33
- Chin KY, Ima-Nirwana S. Sex steroids and bone health status in men. Int J Endocrinol 2012;2012:208719
- Kaufman JM, Vermeulen A. The decline of androgen levels in elderly men and its clinical and therapeutic implications. Endocr Rev 2005;26:833–76
- Filipović B, Šošić-Jurjević B, Ajdžanović V, et al. The effects of sex steroids on thyroid C cells and trabecular bone structure in the rat model of male osteoporosis. J Anat 2013;222:313–20
- Libouban H, Moreau MF, Legrand E, et al. Comparison of histomorphometric descriptors of bone architecture with dual-energy X-ray absorptiometry for assessing bone loss in the orchidectomized rat. Osteoporos Int 2002;13:422–8
- Lelovas PP, Xanthos TT, Thoma SE, et al. The laboratory rat as an animal model for osteoporosis research. Comp Med 2008;58:424–30
- Proell V, Xu H, Schuler C, et al. Orchiectomy upregulates free soluble RANKL in bone marrow of aged rats. Bone 2009;45:677–81
- Gaumet-Meunier N, Coxam V, Robins S, et al. Gonadal steroids and bone metabolism in young castrated male rats. Calcif Tissue Int 2000;66:470–5
- Shuid AN, Abu Bakar MF, Abdul Shukor TA, et al. The anti-osteoporotic effect of Eurycoma Longifolia in aged orchidectomised rat model. Aging Male 2011;14:150–4
- Shuid A, El-arabi E, Effendy N, et al. Eurycoma longifolia upregulates osteoprotegerin gene expression in androgen- deficient osteoporosis rat model. BMC Complement Altern Med 2012;12:152
- Balkan W, Burnstein KL, Schiller PC, et al. Androgen-induced mineralization by MC3T3-E1 osteoblastic cells reveals a critical window of hormone responsiveness. Biochem Biophys Res Commun 2005;328:783–9
- Liu X-H, Kirschenbaum A, Yao S, Levine AC. Androgens promote preosteoblast differentiation via activation of the canonical Wnt signaling pathway. Ann N Y Acad Sci 2007;1116:423–31
- Huber DM, Bendixen AC, Pathrose P, et al. Androgens suppress osteoclast formation induced by RANKL and macrophage-colony stimulating factor. Endocrinology 2001;142:3800–8
- Tracz MJ, Sideras K, Boloña ER, et al. Testosterone use in men and its effects on bone health. A systematic review and meta-analysis of randomized placebo-controlled trials. J Clin Endocrinol Metab 2006;91:2011–16
- Yarrow JF, Conover CF, Purandare AV, et al. Supraphysiological testosterone enanthate administration prevents bone loss and augments bone strength in gonadectomized male and female rats. Am J Physiol Endocrinol Metab 2008;295:E1213–22
- Borst SE, Conover CF, Carter CS, et al. Anabolic effects of testosterone are preserved during inhibition of 5α-reductase. Am J Physiol Endocrinol Metab 2007;293:E507–14
- Tajul Ariff AS, Soelaiman IN, Pramanik J, Shuid AN. Effects of Eurycoma longifolia on testosterone level and bone structure in an aged orchidectomised rat model. Evid Based Complement Alternat Med 2012;2012:818072
- Tyagi A, Rajalakshmi B, Kumar VM. Effects of long-term treatment with testosterone enanthate in rhesus monkeys: I. Pharmacokinetics of testosterone, testicular volume and liver metabolism of testosterone. Int J Androl 1999;22:139–47
- Ramli R, Khamis MF, Shuid AN. Bone Micro-CT assessments in an orchidectomised rat model supplemented with Eurycoma longifolia. Evid Based Complement Alternat Med 2012;2012:501858
- Soung DY, Devareddy L, Khalil DA, et al. Soy affects trabecular microarchitecture and favorably alters select bone-specific gene expressions in a male rat model of osteoporosis. Calcif Tissue Int 2006;78:385–91
- Falahati-Nini A, Riggs BL, Atkinson EJ, et al. Relative contributions of testosterone and estrogen in regulating bone resorption and formation in normal elderly men. J Clin Invest 2000;106:1553–60
- Wang J, Stern PH. Sex-specific effects of estrogen and androgen on gene expression in human monocyte-derived osteoclasts. J Cell Biochem 2011;112:3714–21
- Franklin M, Bu SY, Lerner MR, et al. Dried plum prevents bone loss in a male osteoporosis model via IGF-I and the RANK pathway. Bone 2006;39:1331–42
- Pepene C, Crişan N, Coman I. Elevated serum receptor activator of nuclear factor kappa B ligand and osteoprotegerin levels in late-onset male hypogonadism. Clin Invest Med 2011;34:E232–7
- Frederiksen L, Glintborg D, Hojlund K, et al. Osteoprotegerin levels decrease during testosterone therapy in aging men and are associated with changed distribution of regional fat. Horm Metab Res 2013;45:308–13
- Steffens JP, Herrera BS, Coimbra LS, et al. Testosterone regulates bone response to inflammation. Horm Metab Res 2014;46:193–200
- Hofbauer LC, Hicok KC, Chen D, Khosla S. Regulation of osteoprotegerin production by androgens and anti-androgens in human osteoblastic lineage cells. Eur J Endocrinol 2002;147:269–73
- Borst SE, Lee JH, Conover CF. Inhibition of 5α-reductase blocks prostate effects of testosterone without blocking anabolic effects. Am J Physiol Endocrinol Metab 2005;288:E222–7