Abstract
β-Sitosterol is used as a dietary supplement for lowering plasma cholesterol, and has atherosclerosis preventive, anti-inflammatory, antimicrobial, antipyretic, induced apoptosis, and anticancer effects. In order to understand the effect of the molecule we have investigated the molecule theoretically. The structural, vibrational, and electronic properties of the β-sitosterol molecule have been investigated theoretically by performing molecular mechanics (MM+ force field), semiempirical self-consistent-field molecular-orbital (PM3), and density functional theory (B3LYP) calculations. The geometry of the considered molecule has been optimized; the vibrational dynamics and the electronic properties have been calculated in its ground state in the gas phase. The optimized structure of the molecule is not planar, and its heat of formation is exothermic. The calculated infrared spectrum for β-sitosterol agrees well qualitatively with the experimentally determined FTIR spectrum. The interfrontier molecular orbitals are localized mainly on the double C-C bond, and the energy difference of the corresponding orbitals is relatively small, which makes the molecule kinetically stable. According to the calculated dipole moment, β-sitosterol is a polar molecule. The calculated results for the β-sitosterol molecule in the present study will aid in elucidation of the mechanism of action and may further be used in lipid metabolism drug design studies.
Introduction
Epidemiological studies have shown that the consumption of a diet rich in vegetables and fruits reduces the incidence of various types of cancer, diabetes, and cardiovascular and other chronic diseases, with a possible contribution from plant sterols (CitationLing & Jones, 1995; CitationPegel, 1997; CitationStone & Van Horn, 2002; CitationLeitzmann, 2005; CitationRudkowska, 2008).
Plant sterols are found in large amounts in vegetables, in unrefined plant oils, seeds, nuts, and legumes, and enter the mammalian body only via the diet. Animals can synthesize only cholesterol, whereas plants produce little cholesterol but β-sitosterol, stigmasterol, and campesterol as the major sterols. About half of the dietary cholesterol and merely 5% to sometimes 12.5% of plant sterols are absorbed and are detectable in the blood (CitationHeinemann et al., 1993; CitationPegel, 1997; CitationBosner et al., 1999; CitationGylling & Miettinen, 2005; CitationRyan et al., 2007; CitationVecka et al., 2007). A specific and regulatory transporter system (ABCG5 or ABCG8) functions in absorption and resecretion back into the enteric lumen. Sitosterol binds selectively to the transporters. Genetic mutations may lead to an accumulation of plant sterols in different tissues with different biological effects. Since plant sterols are not metabolized, their plasma concentrations depend on their intestinal absorption and biliary elimination rates. Consumption of large quantities of plant sterols inhibits cholesterol absorption and reduces total serum cholesterol and low density lipoprotein cholesterol by 10% and 15%, respectively (CitationLing & Jones, 1995; CitationAwad & Fink, 2000; CitationBerge et al., 2000; CitationDuester, 2001; CitationOvesná et al., 2004; CitationFernandez & Vega-López, 2005; CitationGylling & Miettinen, 2005; CitationJansen et al., 2006;CitationHazard & Patel, 2007; CitationVecka et al., 2007; Kidambi & Patel, Citation2008; CitationReynolds et al., 2008; CitationRudkowska, 2008; Patch et al., 2006).
β-Sitosterol is the major phytosterol in higher plants such as olive, avocado, and soya bean, and is abundant in vegetable oils, nuts, and fruits. It is found in the serum and tissues of healthy individuals at concentrations 800–1000 times lower than that of endogenous cholesterol. β-Sitosterol differs from cholesterol only in an extra ethyl group on the C-24 in the side chain, providing more bulk (volume) to the molecule in comparison to cholesterol, making the molecule more hydrophobic than cholesterol and poorly soluble in water and oil phases. Recently, β-sitosterol alone and in combination with similar phytosterols has been considered as a dietary supplement for lowering plasma cholesterol, and for its atherosclerosis preventive, anti-inflammatory, antimicrobial, antipyretic, induced apoptosis, and anticancer effects. However, certain individuals with a higher absorption rate of sitosterol should use sitosterol with caution (CitationBouic & Lamprecht, 1999; CitationAwad & Fink, 2000; CitationGerber, 2002; CitationJourdain et al., 2006; CitationJayaprakasha et al., 2007; CitationMoon et al., 2007; CitationAwad et al., 2008).
The objective of this work was to investigate theoretically the β-sitosterol molecule, due to its biological and medical importance, and to provide information for further studies. To the knowledge of the authors there is no detailed theoretical study of the molecule, apart from that considered here. Theoretical (quantum chemical) approaches are good tools to investigate small molecules; we have investigated the structural features and electronic properties theoretically in this work.
Computational methods
The geometry of the β-sitosterol molecule has been optimized using different levels of quantum chemical calculations. Preoptimization was performed by applying the molecular mechanics (MM) method (CitationBurkert & Allinger, 1982) using the MM+ force field (CitationAllinger, 1977). The high computational speed of molecular mechanics makes it easier to perform better optimization using a higher level of computational method. The preoptimized structure was taken, and the semiempirical self-consistent-field molecular-orbital (SCF-MO) method (CitationStewart, 1990) at PM3 (CitationStewart, 1989) level within the restricted Hartree–Fock (RHF) formalism (CitationRoothaan, 1951) was applied to fully optimize the structure and calculate the vibrational spectra. Geometrical optimization was carried out using a conjugate gradient method (Polak–Ribiere algorithm) (Fletcher, 2000). The root mean square (RMS) gradient of 10−5 was set to obtain sufficient structural optimization. Harmonic frequency analysis indicated that all stationary points were true minima (there was no imaginary frequency). At the final stage, the geometry taken from PM3 was used to perform single point calculation with density functional theory (DFT) (CitationKohn & Sham, 1965) using Becke’s three-parameter exchange functional (CitationBecke, 1993) with the Lee–Yang–Parr correlation functional (B3LYP) (CitationLee et al., 1988). DFT calculation was realized using a 6-31G* basis set (CitationHehre et al., 1972). The calculations MM and PM3 were carried out with the HyperChem 7.5 program package (Citation2002), whereas the DFT/B3LYP/6-31G* calculation was carried out with the Gaussian-03 program package (Citation2004).
Results and discussion
Optimized structure
The chemical formula of β-sitosterol is C28H48O. Preoptimization by the MM method using the MM+ force field is quite fast and gives a reliable optimized structure. In the second stage of the optimization procedure, the PM3 method has been applied by taking care of relatively fine criteria. shows the final form of the optimized structure (ball and stick model with labels) of β-sitosterol. PM3 optimization has not changed the MM optimized structure much. As seen from , β-sitosterol appears non-planar in the middle. The energy contributions after MM optimization are given in . It can be seen from that all types of energy contribution are positive; the dominant contributions are due to dihedral, van der Waals (vdW), and angle interactions. The remaining contributions (bond, stretch-bend, and electrostatic) are relatively small. Some of the calculated energy values after PM3 optimization are given in . According to PM3 calculations, the heat of formation of β-sitosterol is exothermic and has a value around–124 kcal/mol; the binding energy is about–7469 kcal/mol. The bond lengths of the optimized structure are displayed in , which includes only the C-C bonds. There is only one double bond in the β-sitosterol molecule, which is located in the middle ring. The C-C double bond is ∼1.34 Å, whereas the C-C single bonds vary between 1.48 and 1.55 Å. There is one oxygen atom in the present system; the C-O single bond length is ∼1.41 Å. C-H bonds are almost the same, ∼1.11 Å, and the O-H bond is ∼0.95 Å. We should note that the structure and bond lengths are valid for the case that the molecule is electronically in its ground state and isolated, namely in the gas phase.
Figure 1. Ball and stick model with labels of optimized structure of the β-sitosterol molecule. Red: oxygen; dark gray: carbon; light gray: hydrogen (PM3 result).
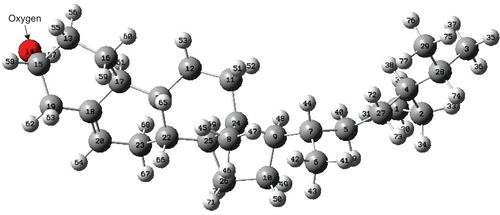
Figure 2. Bond lengths between carbon atoms in β-sitosterol molecule; hydrogen atoms are not displayed (PM3 result).
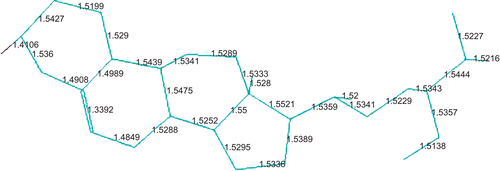
Table 1. Energy contributions (in kcal/mol) after molecular mechanics (MM) method with MM+ force field.
Table 2. Calculated energies (in kcal/mol) after PM3 method.
Vibrational analysis
The vibrational spectrum of β-sitosterol, namely the infrared spectrum (IRS) (in harmonic approximation), has been calculated within the PM3 level. The IRS, including both frequencies and the corresponding intensities, is shown in . There are 225 normal modes (harmonic vibrations) for the molecule studied. The first 16 modes with relatively larger intensity are given in . The vibration directions with relative intensities are displayed in . The vibration with the largest intensity takes place in the ring to which the oxygen atom is bonded. This vibration is due to the stretching of the C-C single bond close to the oxygen. The calculated IRS for β-sitosterol agrees well qualitatively with the experimentally determined Fourier transform infrared (FTIR) spectrum (CitationSigma Aldrich Co., 2009). However, PM3 harmonic frequencies should be scaled by 0.976 for comparison with the experimental values (CitationScott & Radom, 1996).
Table 3. The first 16 relatively larger infrared intensities (in km/mol) and the corresponding harmonic frequencies (in cm−1) after PM3 method.
Electronic properties
Electronic properties have been obtained by performing single point DFT/B3LYP/6-31G* level calculations using the PM3 optimized structure. Some of the calculated energy values are given in . Three-dimensional (3D) images of the highest occupied molecular orbital (HOMO) and the lowest unoccupied molecular orbital (LUMO) are displayed in . As seen from , both the HOMO and the LUMO are localized mainly on the C-C double bond. The HOMO–LUMO energy difference, Eg, has been calculated to be ∼6.8 eV. The HOMO–LUMO energy gap is used as a measure of kinetic stability; a large gap indicates that it is difficult to add electrons to a high LUMO and remove electrons from a deep HOMO (CitationDenis, 2008). However, in some cases the HOMO–LUMO rule fails. For example, it has been demonstrated that for very large molecules it cannot be used as a good indicator of kinetic stability (CitationYoshida & Aihara, 1999). However, β-sitosterol is a relatively small molecule. The calculated energy gap for β-sitosterol may be considered fairly small. Therefore, one may conclude that β-sitosterol may be considered a kinetically stable molecule.
Table 4. Some of the calculated quantities after DFT/B3LYP/6-31G* method.
Figure 5. 3D images of highest occupied molecular orbital (HOMO, upper) and lowest unoccupied molecular orbital (LUMO, lower) of β-sitosterol molecule (DFT/6-31G* results).
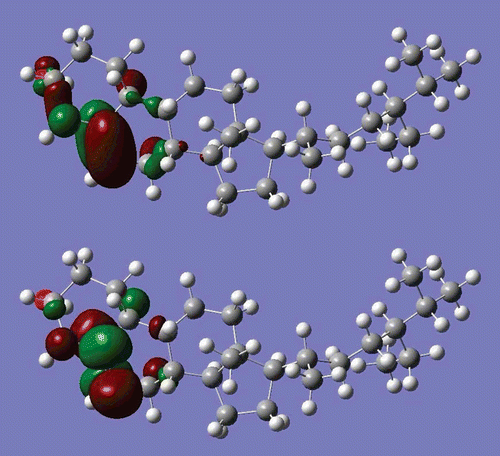
The calculated dipole moment of β-sitosterol is ∼2.5 debyes (D). This dipole moment value is relatively large. Comparing this value with that of water (exp. 1.85 D, same level of cal. 2.02 D), the β-sitosterol molecule has a relatively large dipole moment, and seems to be polar (hydrophilic). Excess charge (Mulliken charge) on the atoms is displayed in . The most interesting feature is the excess charge on the carbon atoms. For instance, a carbon atom bonded to oxygen has a positive excess charge of about +0.092|e|, and one of the double-bonded carbon atoms has a positive excess charge of about +0.139|e|; all the other carbon atoms have a negative excess charge, and vary between about–0.081|e| and–0.424|e|. Positive charge accumulation takes place on hydrogen atoms, and varies between +0.110|e| and +0.147|e|. Another interesting and important feature of charge accumulation is that carbon atoms labeled 7 and 9 and hydrogen atoms bonded to them have a relatively small charge accumulation. These carbon atoms are bonded to each other. This feature may be interpreted by the following. A small charge accumulation on two bonded atoms may cause a relatively weak bond. Therefore, hydrogen atoms bonded weakly to carbons that also form weak bonds may easily be abstracted, and play an important role in radical formation. Tejero and coworkers theoretical analysis for linoleic acid (CitationTejero et al., 2004) supports our argument. The negatively charged oxygen part of the molecule may also play an important role in the interaction of β-sitosterol with its environment. 3D images of charge density and electrostatic potential are displayed in . As seen from the electrostatic potential, the end of the molecule where oxygen is located is an attractive part of β-sitosterol.
Declaration of Interest
One of the authors (S.E.) would like to thank the Middle East Technical University (METU) for partial support through the project METU-BAP-08-11-DPT-2002-K120-510.
References
- Allinger NL (1977): Conformational analysis 130. MM2. A hydrocarbon force field utilizingV1 and V2 torsional terms. J Am Chem Soc 99: 8127–8134.
- Awad AB, Fink CS (2000): Phytosterols as anticancer dietary components: Evidence and mechanism of action. J Nutr 130: 2127–2130.
- Awad AB, Barta SL, Fink CS, Bradford PG (2008): Beta-Sitosterol enhances tamoxifen effectiveness on breast cancer cells by affecting ceramide metabolism. Mol Nutr Food Res 52: 419–426.
- Becke AD (1993): Density-functional thermochemistry. III. The role of exact exchange. J Chem Phys 98: 5648–5652.
- Berge KE, Tian H, Graf GA, Yu L, Grishin NV, Schultz J, Kwiterovich P, Shan B, Barnes R, Hobbs HH (2000): Accumulation of dietary cholesterol in sitosterolemia caused by mutations in adjacent ABC transporters. Science 290: 1709–1711.
- Bosner MS, Lange LG, Stenson WF, Ostlund RE Jr (1999): Percent cholesterol absorption in normal women and men quantified with dual stable isotopic tracers and negative ion mass spectrometry. J Lipid Res 40: 302–308.
- Bouic PJ, Lamprecht JH (1999): Plant sterols and sterolins: A review of their immune-modulating properties. Alternat Med Rev 4: 170–177.
- Burkert U, Allinger NL (1982): Molecular Mechanics. ACS Monograph 177. Washington, ACS.
- Denis PA (2008): Theoretical investigation of nitrogen distributed corannulenes. J Mol Struct (Theochem) 865: 8–13.
- Duester KC (2001): Avocado fruit is a rich source of beta-sitosterol. J Am Diet Assoc 101: 404–405.
- Fernandez ML, Vega-López S (2005): Efficacy and safety of sitosterol in the management of blood cholesterol levels. Cardiovasc Drug Rev 23: 57–70.
- Fletcher P (1990): Practical Methods of Optimization. Weinheim, Wiley-VCH.
- Gaussian-03, Revision D.01. Wallingford, CT, Gaussian, Inc., 2004.
- Gerber GS (2002):Phytotherapy for benign prostatic hyperplasia. Curr Urol Rep 3: 285–291.
- Gylling H, Miettinen TA (2005): The effect of plant stanol- and sterol-enriched foods on lipid metabolism, serum lipids and coronary heart disease. Ann Clin Biochem 42: 254–263.
- Hazard SE, Patel SB (2007): Sterolins ABCG5 and ABCG8: regulators of whole body dietary sterols. Pflügers Arch 453: 745–752.
- Hehre WJ, Ditchfield R, Pople JA (1972): Self-consistent molecular orbital methods. XII. Further extensions of gaussian-type basis sets for use in molecular orbital studies of organic molecules. J Chem Phys 56: 2257–2261.
- Heinemann T, Axtmann G, von Bergmann K (1993): Comparison of intestinal absorption of cholesterol with different plant sterols in man. Eur J Clin Invest 23: 827–831.
- HyperChem, Release 7.5. Gainesville, FL, Hypercube, Inc., 2002.
- Jansen PJ, Lütjohann D, Abildayeva K, Vanmierlo T, Plösch T, Plat J, von Bergmann K, Groen AJ, Ramaekers FC, Kuipers F, Mulder M (2006):Dietary plant sterols accumulate in the brain. Biochim Biophys Acta 1761: 445–453.
- Jayaprakasha GK, Mandadi KK, Poulose SM, Jadegoud Y, Gowda GAN, Bhimanagouda S, Patila BS (2007): Inhibition of colon cancer cell growth and antioxidant activity of bioactive compounds from Poncirus trifoliata (L.) Raf. Bioorg Med Chem 15: 4923–4932.
- Jourdain C, Tenca G, Deguercy A, Troplin P, Poelman D (2006): In-vitro effects of polyphenols from cocoa and beta-sitosterol on the growth of human prostate cancer and normal cells. Eur J Cancer Prev 15: 353–361.
- Kidambi S, Patel SB (2008): Sitosterolaemia: Pathophysiology, clinical presentation and laboratory diagnosis. J Clin Pathol 61: 588–594.
- Kohn W, Sham LJ (1965): Self-consistent equations including exchange and correlation effects. Phys Rev 140: A1133–A1138.
- Lee C, Yang W, Parr RG (1988): Development of the Colle-Salvetti correlation-energy formula into a functional of the electron density. Phys Rev B 37: 785–789.
- Leitzmann C (2005): Vegetarian diets: what are the advantages? Forum Nutr 57: 147–156.
- Ling WH, Jones PJ (1995): Dietary phytosterols: A review of metabolism, benefits and side effects. Life Sci 57: 195–206.
- Moon DO, Lee KJ, Choi YH, Kim GY (2007): Beta-sitosterol-induced-apoptosis is mediated by the activation of ERK and the downregulation of Akt in MCA-102 murine fibrosarcoma cells. Int Immunopharmacol 7: 1044–1053.
- Ovesná Z, Vachálková A, Horváthová K (2004): Taraxasterol and beta-sitosterol: New naturally compounds with chemoprotective/chemopreventive effects. Neoplasma 51: 407–414.
- Patch CS, Tapsell LC, Williams PG, Gordon M (2006): Plant sterols as dietary adjuvants in the reduction of cardiovascular risk: Theory and evidence. Vasc Health Risk Manag 2: 157–162.
- Pegel KH (1997) The importance of sitosterol and sitosterolin in human and animal nutrition. South Afr J Sci 93: 263–268.
- Reynolds TM, Mardani A, Twomey PJ, Wierzbickid AS (2008): Targeted versus global approaches to the management of hypercholesterolaemia. J R Soc Promot Health 128: 248–254.
- Roothaan CC (1951): New developments in molecular orbital theory. Rev Mod Phys 23: 69–89.
- Rudkowska I (2008): Functional foods for cardiovascular disease in women. Menopause Int 14: 63–69.
- Ryan E, Galvin K, O’Connor TP, Maguire AR, O’Brien NM (2007): Phytosterol, squalene, tocopherol content and fatty acid profile of selected seeds, grains, and legumes. Plant Foods Hum Nutr 62: 85–91.
- Scott AP, Radom L (1996): Harmonic vibrational frequencies: an evaluation of Hartree-Fock, Moller-Plesset, quadratic configuration interaction, density functional theory, and semiempirical scale factors. J Phys Chem 100: 16502–16513.
- Sigma Aldrich Co. (2009): Available at http://www.sigmaaldrich.com/spectra/ftir/ FTIR008804.pdf.
- Stewart JJP (1989): Optimization of parameters for semiempirical methods. I. Method. J Comput Chem 10: 209–220.
- Stewart JJP (1990): MOPAC: A semiempirical molecular orbital program. J Comput Aided Mol Design 4: 1–105.
- Stone NJ, Van Horn L (2002): Therapeutic lifestyle change and Adult Treatment Panel III: Evidence then and now. Curr Atheroscler Rep 4: 433–443.
- Tejero I, Eriksson LA, Gonzalez-Lafont Marquet AJ, Lluch JM (2004): Hydrogen abstraction by soybean lipoxygenase-1. Density functional theory study on active site models in terms of Gibbs free energies. J Phys Chem B 108: 13831–13838.
- Vecka M, Zák A, Tvrzická E (2007): Phytosterols as a functional food. Cas Lek Cesk 146: 337–342.
- Yoshida M, Aihara JI (1999): Validity of the weighted HOMO-LUMO energy separation as an index of kinetic stability for fullerenes with up to 120 carbon atoms. Phys Chem Chem Phys 1: 227–230.