Abstract
Increasing evidence demonstrates that stress or depression can lead to atrophy and cell loss in the hippocampus. In contrast, antidepressant treatment significantly reduces apoptosis in the dentate granule cell layer and subgranular zone in animal models of depression. In the present study, we investigated the neuroprotective action of SCLM, the total saponins extracted from Chaihu-jia-longgu-muli-tang, a traditional Chinese medicinal formula which was prescribed 1000 years ago, in the reduction of apoptosis in hippocampal neurons using an experimental chronic mild stress (CMS) model. Mice were subjected to the CMS procedure for a period of 21 consecutive days. SCLM (100 mg/kg, p.o.) or fluoxetine (20 mg/ kg, p.o.) was administered during the stress periods. CMS mice showed a decreased sucrose intake over 21 days, and an increase in the number of TUNEL-positive neurons as well as up-regulation of the apoptotic-related factors, such as Bax and caspase-3 in the hippocampus, compared with control mice. On the other hand, the administration of SCLM (100 mg/kg) and fluoxetine (20 mg/kg) reversed these effects induced by CMS, showing a significant increase of sucrose intake and a dramatic reduction of TUNEL-positive neurons and decreased expression of Bax and caspase-3 proteins. The present results suggest that SCLM possesses a significant antidepressant-like property, and this effect may be through protection against stress-induced neuronal apoptosis by affecting the expression of Bax and caspase-3 proteins in the hippocampus. These findings provide important information that the anti-apoptotic effect of herbal medicine therapy may be beneficial for the treatment of depression.
Introduction
With the development of new imaging tools in the last two decades, increasing evidence has accumulated that depression is accompanied by structural brain changes, especially volume loss of the hippocampus. These structural alterations in the hippocampus include dendritic remodeling of the CA3 pyramidal cells (CitationMagarinos & McEwen, 1995; CitationMcEwen, 2000) and suppression of adult neurogenesis in the dentate gyrus (CitationGould et al., 1997, Citation1998; CitationHeine et al., 2004). On the other hand, in a maternal separation paradigm, CitationLee et al. (2001) reported a protective effect of fluoxetine administration on stress-induced increases in terminal deoxynucleotidyl transferase-mediated dUTP-biotin nick-end labeling (TUNEL)-positive cells in the dentate gyrus. It was also reported that antidepressant treatment with tianeptine significantly reduced TUNEL-identified apoptosis in the dentate granule cell layer and subgranular zone in the chronically stressed tree shrew (CitationLucassen et al., 2004). Based on these findings, we hypothesized that the neuroprotective effect of chronic antidepressant treatment could, at least in part, be mediated through its anti-apoptotic activity in the hippocampus of stressed animal models. Thus, it is important for antidepressant treatment to protect hippocampal neurons against apoptosis in major depression.
In Oriental society, herbs and herbal preparations are widely used as medicines by consumers, with a long history. Some of them may be effective alternatives in the treatment of neuropsychiatric disease such as depression. Chaihu-jia-longgu-muli-tang (saiko-ka-ryukotsu-borei-to in Japanese), a traditional Chinese medicine, has been used as a remedy for more than 1000 years. Recent studies demonstrated that Chaihu-jia-longgu-muli-tang has been widely used in a variety of clinical situations for the treatment of stress-related neuropsychiatric disorders, with very low side effects (CitationIijima et al., 1995). Compositionally, this herbal medicine is made of 11 indigenous medicines: Radix Bupleuri, Bupleurum chinense DC. or Bupleurum scorzonerifolium Willd. (Umbelliferae); Radix Ginseng, Panax ginseng C. A. Mey. (Araliaceae); Rhizoma Pinelliae, Pinelliae ternate (Thunb.) Breit. (Araceae); Poria, Poria cocos (Schw.) Wolf (Polyporaceae); Radix Scutellariae, Scutellaria baicalensis Georgi (Labiatae); Cortex Cinnamomi, Cinnamomum cassia Presl (Lauraceae); Radix et Rhizoma Rhei, Rheum palmatum L., R. palmatum L. var. tanguticum Maxim. ex Rgl., or R. officinale Baill. (Polygonaceae); Concha Ostreae, Ostrea gigas Thunberg, Ostrea talienwhanensis Crosse, or Ostre a rivularis Gould (Osteridae); Os Draconis: this is the skeleton fossil of prehistoric mammals such as hipparion, rhinoceros, deer, ox, elephant, etc.; Rhizoma Zingiberis Recens, Zingiber officinale (Willd.) Rosc. (Zingiberaceae); and Fructus Zizyphi Jujubae, Ziziphus jujuba Mill. (Rhamnaceae). The composition and the amount of each component as a percentage of the whole medicine are shown in . SLCM, a mixture of total saponins extracted from Chaihu-jia-longgu-muli-tang, was assessed in a pilot study, and it was demonstrated that subchronic administration of SCLM (100 and 200 mg/kg, p.o.) for 7 days significantly decreased immobility time in the forced swimming and tail suspension test in mice, indicating a possible antidepressant-like effect. Meanwhile, SCLM showed in vitro a significant neuroprotective action against corticosterone-induced lesions in the rat pheochromocytoma cell line PC12 (unpublished results).
Table 1. Composition of Chaihu-jia-longgu-muli-tang and the amount of each component as a percentage of the whole medicine.
Chronic mild stress (CMS) is generally thought to be the most promising and valuable model to study depression in animals, simulating several human depressive symptoms (CitationWillner, 1997). It has been reported that the CMS regimen decreases the consumption of sucrose solution (anhedonia). Chronic stress alone increased the numbers of apoptotic cells in hippocampal subregions and cortex in the adult tree shrew (CitationLucassen et al. 2001), while antidepressant treatment had an anti-apoptotic effect in stressed animals (CitationLucassen et al. 2004), suggesting that chronic antidepressant treatment may have a neuroprotective effect.
The present study was designed to examine the possible inhibitory action of SCLM on apoptosis in the hippocampus using the CMS model in mice. For this purpose, we examined the effects of SCLM on the chronic stress-induced increase in TUNEL-positive hippocampal neurons, as well as the apoptotic-related expression of Bax and caspase-3 proteins.
Materials and methods
Plant material
The medicinal plants used to prepare Chaihu-jia-longgu-muli-tang are listed in . They were purchased from Nanjing Herbal Materials Company (Nanjing, China). Voucher specimens were conserved at the herbarium of China Pharmaceutical University, and authenticated by Professor Qin Minjian, School of Chinese Materia Medica, China Pharmaceutical University, based on their microscopic and macroscopic characteristics.
Preparation of saponins
SCLM extracted from Chaihu-jia-longgu-muli-tang was prepared using the following procedure. The mixed medicinal materials weighing about 300 g were defatted in a Soxhlet apparatus with n-hexane, and the defatted herbal medicine was extracted with methanol. The methanol-soluble fraction was collected and evaporated. The remaining syrup was then mixed with 80% n-butanol and the n-butanol-soluble fraction was separated using a separatory funnel. After further extraction with 80% n-butanol, the extract was dried under a vacuum. The n-butanol fraction was used as the crude saponin extract. The saponin extract was 3.3 g (yield 1.1%, w/w). The extract consisted mainly of triterpenoid saponins according to phytochemical tests, the vanillin–perchloric acid reaction, and thin layer chromatography (TLC) analytical conditions. SCLM was dissolved in distilled water just before use.
Animal care and treatment
Male KM mice weighing 18–22 g were purchased from the Experimental Animal Center of China Pharmaceutical University. The animals were housed in groups under controlled conditions of light (12 h light/dark cycle, lights on at 7:00 a.m.), humidity (50 ± 10%), and temperature (25 ± 1°C), with free access to food and water. The animals were acclimatized to the laboratory for at least 3 days before they were tested, and were randomly assigned to the various experimental groups (n = 8/group in chronic mild stress and sucrose intake assay; n = 5/group in immunohistochemical measurement) and weighed daily. All experiments followed a protocol approved by the local animal Ethics Committee and the local government. All experiments were done in accordance with the recommendations of the European Union regarding animal experimentation (Directive of the European Council 86/609/EC).
Chronic mild stress model
The chronic mild stress regimen used in this study was based on the procedure originally designed by CitationWillner et al. (1992) and adapted to mice (CitationDucottet & Belzung, 2004). Mice were divided into four equal groups (n = 8): control, stress + vehicle, stress + SCLM (100 mg/kg, p.o.), and stress + fluoxetine (20 mg/kg, p.o., purity 99%, bought from Sigma, USA). Stressed animals were subjected to the CMS procedure for a period of 21 successive days. Various stressors were applied in a random order several times a day during the 21-day procedure: cage tilt at 45°, housing in an empty cage (without sawdust), tail pinch (1 cm from the end of the tail), placement in an empty cage with 1.5 cm water, reversal of the light/dark cycle, swimming in cold water (4°C), and housing in damp sawdust. All the stressors and/or sequences were administered at different time points every day (see ). The stress procedure did not involve any food or water deprivation. Stressed mice were singly housed at the start of the procedure, while control mice were maintained group-housed during the whole experiment in a separate room. SCLM (100 mg/kg) or fluoxetine (20 mg/kg) was administrated orally once a day for 21 days during the stress process, while vehicle control and vehicle stress were given orally as distilled water only once daily for 21 days. Two measures of sensitivity to chronic stress were recorded: sucrose intake and body weight. At least 1 day of rest was provided between a stressor and a measure in order to avoid a direct effect of the last stressor on the subsequent observations.
Table 2. Procedure of the chronic mild stress model.
Sucrose intake test and measurement of body weight
Mice were first trained to consume a palatable 1% sucrose solution over a 3-day period during laboratory acclimation, with free access to both water and a sucrose solution. This was followed by a 24 h period during which only sucrose bottles were available, and by a 6 h sucrose consumption test in single cages, during which both bottles were available. This was done in order to familiarize mice with the sucrose preference procedure. Then, sucrose preference testing was carried out before the beginning of CMS (initial values) and on day 2 after its end (final values). During testing, mice were singly housed with both sucrose and tap water in 15 mL glass bottles. Bottles were available at 9:00 h and removed at 15:00 h so that testing occurred during the dark phase, as recommended by CitationD’Aquila et al. (1997). The weight of the sucrose solution was recorded. CMS-induced sucrose intake changes were defined as differences between final and initial values. Bottles were counterbalanced across the left and right sides of the feeding compartment, and alternated in position between the different testing sessions to prevent a position habit.
Body weight was measured before (initial values) and after the chronic stress (final values), and CMS-induced weight changes were obtained by subtracting these two values.
Tissue preparation
Each mouse was deeply anesthetized with nembutal (50 mg/kg, i.p.) and was transcardially perfused with 100 mL saline followed by 200 mL cold 0.01 M phosphate buffered saline (PBS), pH 7.4, containing 4% paraformaldehyde. The brain was removed from the skull and the hippocampus was dislodged and postfixed with 4% paraformaldehyde in phosphate buffer for 1 day. Subsequently, sections (7 μm) were cut on a Vibratome (Leica), and sections were processed for TUNEL staining or immunohistochemical assay. All sections were coded, and the code was broken only when all quantification of the assay was completed.
TUNEL staining
TUNEL staining was performed using a commercially available kit (in situ Apoptosis Detection Kit; Boster, China) following the manufacturer’s protocol, as described before (CitationOmman et al. 2004). Briefly, TUNEL was performed on 7 μm thick sections attached to slides obtained from fixed brains of control, stressed, or fluoxetine- or SCLM-treated stressed mice, 21 days following the CMS. Tissue sections were deparaffinized by serial washing using xylene and dehydrated to ethanol 50% and distilled water, and endogenous peroxidase was blocked by treatment with methanol containing 3% H2O2. After washing with distilled water and PBS, sections were incubated with the TUNEL reaction mixture for 1 h at 37°C. Incorporation of labeled oligonucleotides was stopped by briefly rinsing the sections in distilled water and PBS. Sections were preincubated with PBS/1% bovine serum albumin (BSA) and incubated with peroxidase-conjugated avidin (ABC-elite kit; Boster, China) 1:1000 in PBS/1% BSA overnight at 4°C. After washing in PBS, sections were incubated with 0.5 mg/mL diaminobenzidine (DAB) (Sigma) in 0.05 mol/L Tris/HCl (pH 7.5) with 0.02% H2O2 for 10 min, washed twice in distilled water, and lightly counterstained with Mayer’s hematoxylin before mounting on coverslips. Apoptotic indices for the hippocampus of control and stressed animals are presented as the number of TUNEL-positive nuclei/1000 cells, and/or the number of TUNEL-positive nuclei/high powered field (HPF, ×20). Six non-overlapping HPFs at ×20 magnification of a brain region were scored for each region/animal and the mean was calculated to obtain a single value/region/animal. At least four slides from each mouse were analyzed for this purpose.
Each experiment included negative and positive controls. Negative controls were performed using double-distilled H2O to replace terminal deoxynucleotidyl transferase (TdT) enzyme. The negative control sections did not display TUNEL-positive cells. The positive control sections were incubated, prior to TUNEL staining, with DNA polymerase I (10 μg/mL, 10 min at room temperature) to induce DNA strand breaks.
Immunohistochemistry
For Bax and caspase-3 immunohistochemical assay, specimens were transferred to adhesive slides, and dried in an autoclave at 37°C overnight and at 60°C for 20 min. They were deparaffinized and dehydrated by immersion into xylene twice for 10 min and alcohol twice for 2 min. The specimens were then placed in 3% H2O2 for 5 min to inhibit endogenous peroxidase. Bax and caspase-3 primary antibodies were obtained from Boster Biotechnology (China); these specifically recognize rabbit anti-Bax (diluted 1:200) and rabbit anti-caspase-3 (diluted 1:150). Sections were then washed in PBS and incubated for 1 h in the presence of biotinylated rabbit anti-mouse secondary antibody (Antibody Diagnostic, Inc., USA). Sections were washed again in PBS and further processed by sequential incubations in ABC reagent (avidin–peroxidase complex) and diaminobenzidine (DAB) (Sino-American Biotechnology Company, Beijing) chromogen for 20 min. The expression of Bax and caspase-3 proteins was quantified as a gray value (GV) using computer image analysis. The images were processed by the CMIAS-98A Pathological Image Analytical Computer Research System (Beijing University of Aeronautics and Astronautics Image Analysis Center, Beijing, China) and connected to a light microscope (BH-2 microscope; Olympus, Tokyo, Japan). A researcher blind to the treatment group of each section analyzed the cells positive for Bax and caspase-3 proteins. Five individual 3000 μm2 fields were measured on each section and five sections for each mouse were analyzed.
Statistical analysis
Data are presented as the mean ± SEM for each experimental endpoint. Group differences relative to control were determined by one-way analysis of variance (ANOVA) followed by Dunnett’s t-test. Apoptotic indices were analyzed by non-paired t-test. Statistical significance was accepted as p < 0.05.
Results
Sucrose intake and body weight
Sucrose consumption in control and CMS mice is shown in . Chronic mild stress induced a significant reduction of the sucrose intake compared with control mice (–0.27 ± 0.11 g, p < 0.001), while with chronic administration of SCLM (100 mg/kg) or fluoxetine (20 mg/kg) for 21 days, the reduced sucrose intake was markedly reversed compared to vehicle-treated stressed mice (–0.089 ± 0.06, −0.075 ± 0.066 g, respectively). There was no significant difference of body weight among the groups (p > 0.05).
Table 3. Effect of SCLM and fluoxetine on CMS-induced changes of sucrose intake and body weight in mice.
SCLM reduces TUNEL-positive neuron numbers in hippocampus in stressed mice
In vehicle-treated control mice, most of the neurons were TUNEL-negative, showing a normal size of nucleus and a lower apoptotic index (). After 21 days of stress treatment, the dying neurons exhibited typical apoptotic morphology, including condensed chromatin, shrunken nuclei, and apoptotic bodies, appearing TUNEL-positive (). SCLM (100 mg/kg) or fluoxetine (10 mg/kg) treatment resulted in a significant alteration in the hippocampal levels of apoptotic neurons toward their control values (), showing a sharp decrease of the numbers of TUNEL-positive neurons.
Figure 1. (a) SCLM inhibited levels of TUNEL-positive cells in the hippocampus of stressed mice. (A) Control; (B) stressed mice: numerous TUNEL-positive neurons could be observed, dark brown (arrows); (C) fluoxetine-treated (20 mg/kg) stressed mice: TUNEL-positive neurons were significantly decreased (arrows); (D) SCLM-treated (100 mg/kg) stressed mice: TUNEL-positive neurons were significantly reduced (arrows). (b) Effect of SCLM on apoptotic index in hippocampus of stressed mice. Apoptotic indices were calculated from sections of control and stressed mouse brain, as number of stained nuclei/1000 cells/HPF as described in “Materials and methods” (0.132 ± 0.025, 0.782 ± 0.079, respectively). Note that the numbers of TUNEL-positive nuclei were increased in dentate gyrus after 21-day stress treatment as compared to respective controls. SCLM (100 mg/kg) or fluoxetine (20 mg/kg) treatment resulted in a significant decrease in levels of apoptotic hippocampal neurons toward control values (0.294 ± 0.038, 0.282 ± 0.028, respectively). Values are mean ± SEM, n = 5. Data analysis was performed using non-paired t-test. ###p < 0.001, compared with control group, **p < 0.001, compared with vehicle-treated stressed mice.
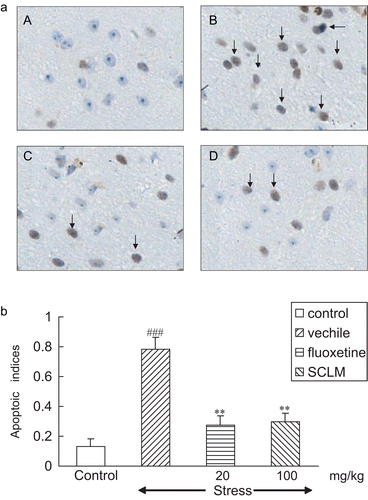
Apoptotic indices were calculated from sections of control and stressed mouse brain as the number of stained nuclei/1000 cells/HPF (0.132 ± 0.025, 0.782 ± 0.079, respectively, p < 0.001). Note that the numbers of TUNEL-positive nuclei were increased in the hippocampus after the 21-day stress treatment as compared to respective controls. SCLM (100 mg/kg) or fluoxetine (20 mg/kg) treatment resulted in a significant decrease in the levels of apoptotic hippocampal neurons toward control values (0.294 ± 0.038, 0.282 ± 0.028, respectively, p < 0.001) as shown in .
SCLM inhibits Bax and caspase-3 expression in hippocampus of stressed mice
To confirm the inhibitory effect of SCLM on apoptotic hippocampal neurons of stressed mice, Bax and caspase-3 activities were determined by immunohistochemical assay. In parallel to TUNEL-staining, Bax- and caspase-3-positive cells were markedly increased in the hippocampus of stressed mice. In contrast, in stressed mice that began receiving SCLM (100 mg/kg) or fluoxetine (20 mg/kg) supplement for 21 days, the number of caspase-3- and Bax-positive cells was significantly reduced in the brain after CMS induction ( and ).
Figure 2. (a) SCLM inhibited up-regulation of Bax in the hippocampus of stressed mice. (A) Control; (B) stressed mice: numerous Bax-positive neurons could be observed, dark brown (arrow); (C) fluoxetine-treated (20 mg/kg) stressed mice: Bax-positive neurons were significantly decreased; (D) SCLM-treated (100 mg/kg) stressed mice: Bax-positive neurons were significantly reduced. (b) Staining was quantified as gray values (GVs) using computer image analysis (n = 5). CMS significantly increased the GV of Bax in the hippocampus compared with control mice (158.54 ± 19.05, 34.98 ± 8.02, respectively), while SCLM (100 mg/kg) or fluoxetine (20 mg/kg) treatment resulted in a significant decrease in GV levels (41.76 ± 12.58, 38.04 ± 11.94, respectively). Data analysis was performed using Dunnett’s t-test. ###p < 0.001, compared with control group, ***p < 0.001, compared with vehicle-treated stressed mice.
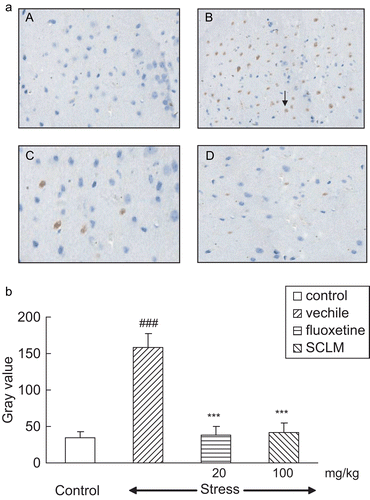
Figure 3. (a) SCLM inhibited up-regulation of caspase-3 in the hippocampus of stressed mice. (A) Control; (B) stressed mice: numerous neurons with positive staining of caspase-3 could be observed, dark brown (arrow); (C) fluoxetine-treated (20 mg/kg) stressed mice: caspase-3-positive neurons were significantly reduced; (D) SCLM-treated (100 mg/kg) stressed mice: caspase-3-positive neurons were significantly decreased. (b) Staining was quantified as gray values (GVs) using computer image analysis (n = 5). CMS induced a significant elevation of caspase-3 GV as compared to control (185.92 ± 20.93, 53.92 ± 5.96, respectively); with SCLM (100 mg/kg) or fluoxetine (20 mg/kg) treatment for 21 days, these increases were reversed markedly (63.48 ± 16.23, 59.72 ± 12.32, respectively). Data analysis was performed using Dunnett’s t-test. ###p < 0.001, compared with control group, ***p < 0.001, compared with vehicle-treated stressed mice.
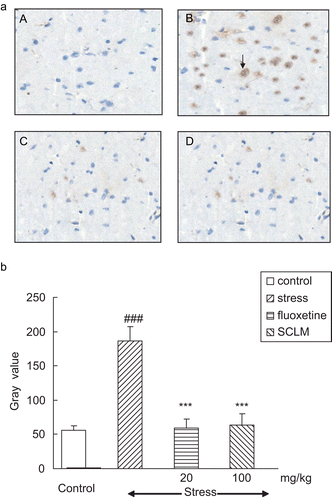
The values of Bax and caspase-3 protein expression were quantified as gray values (GVs) using computer image analysis. It can be seen in that CMS significantly increased the GV of Bax in the hippocampus compared with control mice (158.54 ± 19.05, 34.98 ± 8.02, respectively, p < 0.001), while SCLM (100 mg/kg) or fluoxetine (20 mg/kg) treatment resulted in a significant decrease in the GV levels (41.76 ± 12.58, 38.04 ± 11.94, respectively, p < 0.001) compared with vehicle-treated stressed mice. Meanwhile, CMS induced a significant elevation of caspase-3 GV as compared to control (185.92 ± 20.93, 53.92 ± 5.96, respectively, p < 0.001); with SCLM (100 mg/kg) or fluoxetine (20 mg/ kg) treatment for 21 days, these increases were reversed markedly (63.48 ± 16.23, 59.72 ± 12.32, respectively, p < 0.001), compared with vehicle-treated stressed mice ().
Discussion
The present study examined the suppressive effect of chronic SCLM treatment on apoptotic cells in the hippocampus of mice using the chronic mild stress model of depression. SCLM and the reference antidepressant fluoxetine markedly increased the CMS-induced reduction of sucrose intake, exerting a dramatic antidepressant-like effect, which was consistent with our previous observation in the tail suspension and forced swimming test (in a pilot study). In addition, both SCLM and fluoxetine had significant anti-apoptotic action on hippocampal cells in stressed mice.
As shown in , CMS induced a significant reduction of the sucrose intake compared with control mice, while with chronic administration of SCLM (100 mg/kg) or fluoxetine (20 mg/kg) for 21 days, the reduced sucrose intake was markedly reversed compared to vehicle-treated stressed mice. Furthermore, CMS increased the numbers of apoptotic cells, as demonstrated by the increase of positive labeling of apoptotic cells by TUNEL assay, confirming earlier results demonstrated in the chronic psychosocial stress model (CitationLucassen et al., 2001). In contrast, treatment with SCLM (100 mg/kg) for 21 days significantly reversed the elevated TUNEL-positive cells induced by the CMS model, which was consistent with the effect of the positive control fluoxetine.
More recent theories suggest that major depressive disorders may be associated with an impairment of structural plasticity and cellular resilience, and that antidepressant medications can normalize this impairment (CitationDuman et al., 1999; CitationManji & Duman, 2001). The hippocampus is the brain region that most often contributes to certain symptoms of depression. It has been reported that the hippocampal volume is reduced significantly in major depressive patients compared with healthy controls (CitationBremner et al., 2000). Exposure to chronic stress plays an important role in the etiology of depression, and can precipitate or worsen depression (CitationBrown et al., 2003; CitationGold & Chrousos, 2002). Stress procedures are often used as models in preclinical studies; as mentioned earlier, chronic stress alone increased the numbers of apoptotic cells in hippocampal subregions and the cortex in the adult tree shrew (CitationLucassen et al., 2001), which was probably due to a stress-induced increase in apoptotic neurons in the hippocampus.
Apoptosis, also known as programmed cell death, is a form of cell death that occurs during several pathological situations and contributes to cell replacement, tissue remodeling, and removal of damaged cells. The bax gene is a member of the Bcl-2 gene family and a major regulator of programmed cell death (CitationBrady & Gil-Gomez, 1998). A number of studies have shown that Bax protein interacts with the mitochondrial membrane resulting in loss of membrane integrity, cytochrome C release, caspase activation, and apoptosis (CitationDargusch et al., 2001). The caspases, a class of cysteine proteases, are considered to be central players in the apoptotic process and trigger a cascade of proteolytic cleavage events in mammalian cells. Caspase-3, the most widely studied member of the caspase family and one of the key executioners of apoptosis, was involved in neuronal apoptosis induced by different stimuli in vitro (CitationEldadah et al., 1997; CitationKrohn et al., 1998) and by different animal models (CitationNamura et al., 1998; CitationVelier et al., 1999). In our present study, the expression of Bax and caspase-3 proteins increased dramatically in the hippocampus of stressed mice compared to control mice, whereas, as can be seen in and , these effects were significantly reversed after chronic administration of SCLM or fluoxetine. According to our results, it can be suggested that the anti-apoptotic effect of SCLM may be mediated through the down-regulative action of Bax and caspase-3 protein expression. The decreased level of Bax and caspase-3 plays an important role in the regulation of cell survival. Therefore, we presume that the neuroprotective effect of SCLM might be through an increase of surviving cells as well as a decrease of dead cells. However, whether or not other apoptosis-related proteins are involved in the anti-apoptotic effect of SCLM needs further clarification.
Increasing advances have been applied to studies of antidepressants, and have resulted in the novel hypothesis that altered neurogenesis plays a key role in the etiology and treatment of depression (CitationDuman et al., 2001). Studies in experimental animals demonstrate that the decreased creation of new neurons in the adult hippocampus could contribute to the volume loss. In contrast, antidepressant treatment increases neurogenesis in the hippocampus of adult animals and antogonizes the effects of stress. Chronic mild stress produces a profound effect on neurogenesis, causing rapid and robust reductions in the proliferation of new neurons in the adult brain (CitationAlonso et al., 2004). In mice, the induction of adult hippocampal neurogenesis by environmental stimuli leads to an increase in volume of the dentate gyrus (CitationKempermann et al., 1997). It has also been suggested that the onset lag of the antidepressive effect might reflect the maturation period of a new neuron. In the present study, the antidepresant-like as well as the anti-apoptotic action of SCLM after chronic administration may be explained, at least partly, by the theory that neurogenesis generally is a slow process and a long-term adaptation of the functional systems; similarly, the potential contribution of adult neurogenesis to clinical improvement would occur on a long time scale.
In summary, from the results of the present study, we suggest that chronic mild stress significantly induces physical and neurological abnormalities such as a reduction of sucrose consumption and an increase of apoptotic cells in the hippocampus in mice. In addition, SCLM, total saponins extracted from Chaihu-jia-longgu-muli-tang, exerts a significant neuroprotective effect against stress-induced apoptosis in the hippocampus of stressed mice. The current findings support recent theories proposing that the therapeutic effects of antidepressants may be mediated, at least in part, through protective properties and an influence on neuron viability. It is possible that SCLM, by attenuating stress-induced apoptosis, may offer information important for the treatment of depression.
Declaration of interest
This research was supported by the Jiangsu Natural Science Foundation (BK2005149), Jiangsu, People’s Republic of China. The authors declared no biomedical financial interests or potential conflicts of interest.
References
- Alonso R, Griebel G, Pavone G, Stemmelin J, Le Fur G, Soubie P (2004): Blockade of CRF (1) or V (1b) receptors reverses stress-induced suppression of neurogenesis in a mouse model of depression. Mol Psychiatry 9: 278–286.
- Brady HJ, Gil-Gomez G (1998): The pro-apoptotic Bcl-2 family member, Bax. Int J Biochem Cell Biol 30: 647–650.
- Bremner JD, Narayan M, Anderson ER, Staib LH, Miller HL, Charney DS (2000): Hippocampal volume reduction in major depression. Am J Psychiatry 157: 115–118.
- Brown J, Cooper-Kuhn CM, Kempermann G, Van Praag H, Winkler J, Gage FH, Kuhn HG (2003): Enriched environment and physical activity stimulate hippocampal but not olfactory bulb neurogenesis. Eur J Neurosci 17: 2042–2046.
- D’Aquila PS, Newton J, Willner P (1997): Diurnal variation in the effects of chronic mild stress on sucrose intake and preference. Physiol Behav 62: 421–426.
- Dargusch R, Piasecki D, Tan S, Liu Y, Schubert D (2001): The role of Bax in glutamate-induced nerve cell death. J Neurochem 76: 295–301.
- Ducottet C, Belzung C (2004): Behaviour in the elevated plus-maze predicts coping after subchronic mild stress in mice. Physiol Behav 81: 417–426.
- Duman RS, Malberg J, Thome J (1999): Neural plasticity to stress and antidepressant treatment. Biol Psychiatry 46: 1181–1191.
- Duman R, Malberg J, Nakagawa S (2001): Regulation of adult neurogenesis by psychotropic drugs and stress. J Pharmacol Exp Ther 299: 401–407.
- Eldadah BA, Yakovlev AG, Faden AI (1997): The role of CED-3-related cysteine proteases in apoptosis of cerebellar granule cells. J Neurosci 16: 6105–6113.
- Gold P, Chrousos GP (2002): Organization of the stress system and its dysregulation in melancholic and atypical depression: High vs low CRH/NE states. Mol Psychiatry 7: 254–275.
- Gould E, McEwen BS, Tanapat P, Galea LA, Fuchs E (1997): Neurogenesis in the dentate gyrus of the adult tree shrew is regulated by psychosocial stress and NMDA receptor activation. J Neurosci 17: 2492–2498.
- Gould E, Tanapat P, McEwen BS, Flugge G, Fuchs E (1998): Proliferation of granule cell precursors in the dentate gyrus of adult monkeys is diminished by stress. Proc Natl Acad Sci USA 95: 3168–3171.
- Heine VM, Maslam S, Zareno J, Joels M, Lucassen PJ (2004): Suppressed proliferation and apoptotic changes in the rat dentate gyrus after acute and chronic stress are reversible. Eur J Neurosci 19: 131–144.
- Iijima OT, Minematsu S, Maemura S, Waterson LA, Gopinath C, Connick HA (1995): Single oral dose toxicity study and a 13-week repeated dose study with a 4-week recovery period of TSUMURA saiko-ka-ryukotsu-borei-to (TJ-12) in rats. Jpn Pharmacol Ther 23(Suppl. 95): S1739–S1753.
- Kempermann G, Kuhn HG, Gage FH (1997): More hippocampal neurons in adult mice living in an enriched environment. Nature 386: 493–495.
- Krohn AJ, Preis E, Prehn JHM (1998): Staurosporine-induced apoptosis of cultured rat hippocampal neurons involves caspase-1-like proteases as upstream initiators and increased production of superoxide as a main downstream effector. J Neurosci 18: 8186–8197.
- Lee HJ, Kim JW, Yim SV, Kim MJ, Kim SA, Kim YJ (2001): Fluoxetine enhances cell proliferation and prevents apoptosis in dentate gyrus of maternally separated rats. Mol Psychiatry 6: 725–728.
- Lucassen PJ, Vollmann-Honsdorf GK, Gleisberg M, Czeh B, De Kloet ER, Fuchs E (2001): Chronic psychosocial stress differentially affects apoptosis in hippocampal subregions and cortex of the adult tree shrew. Eur J Neurosci 14: 161–166.
- Lucassen PJ, Fuchs E, Czeh B (2004): Antidepressant treatment with tianeptine reduces apoptosis in the hippocampal dentate gyrus and temporal cortex. Biol Psychiatry 55: 789–796.
- Magarinos AM, McEwen BS (1995): Stress-induced atrophy of apical dendrites of hippocampal CA3c neurons: Involvement of glucocorticoid secretion and excitatory amino acid receptors. Neuroscience 69: 89–98.
- Manji HK, Duman RS (2001). Impairments of neuroplasticity and cellular resilience in severe mood disorders: Implications for the development of novel therapeutics. Psychopharmacol Bull 35: 5–49.
- McEwen BS (2000): The neurobiology of stress: From serendipity to clinical relevance. Brain Res 886: 172–189.
- Namura S, Zhu J, Fink K, Endres M, Srinivasan A, Tomaselli KJ, Yuan J, Moskowitz MA (1998): Activation and cleavage of caspase-3 in apoptosis induced by experimental cerebral ischemia. J Neurosci 18: 3659–3668.
- Omman S, Finckbone V, Dertien J, Attrideg J, Henne W, Medina M, Mansouri B, Singh H, Strahlendorf H, Strahlendorf J (2004): Active caspase-3 expression during postnatal development of rat cerebellum is not systematically or consistently associated with apoptosis. J Comp Neurol 16: 154–173.
- Velier JJ, Ellison JA, Kikly KK, Spera PA, Barone FC, Feuerstein GZ (1999): Caspase-8 and caspase-3 are expressed by different populations of cortical neurons undergoing delayed cell death after focal stroke in rat. J Neurosci 19: 5932–5941.
- Willner P, Muscat R, Papp M (1992). Chronic mild stress-induced anhedonia: A realistic animal model of depression. Neurosci Biobehav Rev 16: 525–534.
- Willner P (1997): Validity, reliability and utility of the chronic mild stress model of depression: A 10-year review and evaluation. Psychopharmacology (Berl) 134: 319–329.