Abstract
Context: Macrotyloma uniflorum (Lam.) Verdc. (Leguminosae) seeds, known as the poor man’s pulse crop in India, have been used as a food and also used in the traditional method for treatment of kidney stones, diabetes, obesity, etc.
Objective: To investigate the antidiabetic effect of α-amylase inhibitor isolated from the seeds of Macrotyloma uniflorum seeds in streptozotocin-nicotinamide induced diabetic mice.
Materials and method: α-Amylase inhibitor was purified usng a carboxymethyl cellulose (CMC) column. Kinetic studies were done using mouse pancreatic and human salivary α-amylase. Its antidiabetic effect was studied in streptozotocin-nicotinamide-induced diabetic mice. Biochemical parameters such as serum total cholesterol, aspartate aminotransferase (AST) and alanine aminotransferase (ALT) levels were determined. Histopathological investigation was performed on the pancreas, kidney, and liver tissue samples.
Results: Macrotyloma uniflorum α-amylase inhibitor (MUAI) inhibited both the mouse pancreatic and human salivary α-amylase in a non-competitive manner with Ki values of 11 and 8.8 µM and IC50 value of 30 and 12.5 µg/mL, respectively. It decreased the serum glucose level in the treated diabetic mice. Histological findings suggested minimum pathological changes in the treated diabetic mice as compared to the diabetic control.
Discussion and conclusion: The results suggest that MUAI has an antihyperglycemic activity and therefore can be used in the dietary treatment of non-insulin dependent diabetes mellitus.
Introduction
Macrotyloma uniflorum (Lam.) Verdc. (Leguminosae), commonly known as horse gram and as kulthi in India, is an important legume grown by small-scale farmers. It is largely cultivated in dry areas of Australia, Burma, India, and Sri Lanka (CitationSiddhuraju & Manian, 2007). It is used as a vegetable in India and known as the poor man’s pulse crop in southern India (CitationSiddhuraju & Manian, 2007). The whole seed is reported to be a good source of protein (17.95.3%), carbohydrates (51.960.9%), essential amino acids, energy; it has a low lipid content (0.582.06%) and is an excellent source of iron and molybdenum (CitationBravo et al., 1998). The seeds are dry roasted and eaten after boiling or frying either whole or as meal (CitationChinta et al., 2008). They have been used for the treatment of heart conditions, asthma, kidney stones, bronchitis, leukoderma, urinary discharges, and obesity (CitationKawsar et al., 2009; CitationSiddhuraju & Manian, 2007). They possess slow digestible starch, which is considered to have low postprandial glucose response when consumed by diabetic patients (CitationBravo et al., 1998).
Type II diabetes is caused by impaired secretion of insulin resulting in high postprandial glucose levels. The control of postprandial hyperglycemia is an important strategy in the management of type II diabetes mellitus and reducing chronic complications associated with the disease (CitationAli et al., 2006; CitationSubramanian et al., 2008). One of the therapeutic approaches for reducing postprandial hyperglycemia in patients with diabetes mellitus is to prevent absorption of carbohydrate after food uptake. The digestion of carbohydrates in the intestine is achieved by pancreatic α-amylases and other α-glucosidases. These enzymes are inhibited by complex oligosaccharides like acarbose thereby reducing the postprandial digestion and absorption of starch (CitationDavis & Granner, 2001).
Inhibitors of α-amylases have applications in modifying and controlling α-amylase action that have medical applications such as the influence on blood glucose, serum insulin and starch loading tests in animals and man (CitationPuls & Keup, 1973). Screening of α-glucosidase inhibitors from plants and synthetic sources is increasing. Inhibitors of these enzymes have been recently developed from natural sources (CitationShim et al., 2003). However, extensive studies on the antidiabetic potential of α-amylase inhibitors from Macrotyloma uniflorum seeds are not reported. Therefore, the objective of the present investigation was to evaluate the possible role of α-amylase inhibitor isolated from the seeds of Macrotyloma uniflorum in the management of type II diabetes mellitus by using streptozotocin-nicotinamide-induced diabetic mice as model.
Material and methods
Collection and authentication of plant
Macrotyloma uniflorum seeds were purchased from the local market and authenticated by A.M. Mujumdar at the Agharkar Research Institute, Department of Botany, Pune. The voucher specimen was deposited at that institute (Authentication no. 08-162).
Drugs and chemicals
Streptozotocin and nicotinamide were purchased from SRL (Mumbai, India). Acarbose (Micro Labs, Bangalore, India) and glucose estimation kit (glucose oxidase/peroxidase kit, Accurex Biomedical, Mumbai, India) were purchased from respective vendors. All the biochemical estimation kits were purchased from Pathozyme Diagnostics (Kolhapur, India). All other chemicals and reagents used were of analytical grade.
Preparation of the extract and isolation of the α-amylase inhibitor
Macrotyloma uniflorum seed meal (100 g) was extracted with 500 mL of physiological saline (0.145 M NaCl) for 4 h at 4°C. The extract was centrifuged at 12,080 g and the supernatant thus obtained was subjected to fractional precipitation with ammonium sulfate. The protein fraction precipitating between 30% and 80% saturation of ammonium sulfate was collected by centrifugation, dissolved in minimum amount of distilled water, extensively dialyzed against distilled water and finally against acetate buffer (pH 5.4,10 mM). The dialyzed protein solution, clarified by centrifugation (Fraction A) was used to isolate α-amylase inhibitor by ion exchange chromatography on a CMC column at pH 5.4 using acetate buffer (10 mM).
The adsorbed proteins were eluted with a discontinuous gradient (0.05 M, 0.2 M, 0.5 M and 1 M) of sodium chloride. Fractions of 5 mL each were collected and monitored for protein content by monitoring the absorbance at 280 nm on a spectrophotometer (Jasco V-630 spectrophotometer, Tokyo, Japan) and α-amylase inhibitory activity. α-Amylase inhibitor rich fractions were pooled, lyophilized and used for the studies.
Animals and research protocol approval
Swiss albino male mice (30 ± 5 g) were purchased from the Institute of Veterinary and Biological Products, Pune, India. The animals were housed in an air-conditioned room at a temperature of 22° ± 2°C and relative humidity of 45% to 55% under 12 h light:12 h dark cycle. The animals had free access to food pellets (Chakan Oil Mills, Pune, India) except when starvation was required. Water was provided ad libitum. The experimental protocol was approved by the Institutional Animal Ethics Committee (IAEC) of Pune University, Pune, India.
Drug solutions
MUAI and acarbose were dissolved in distilled water. Streptozotocin was dissolved in citrate buffer (pH 4.5) and nicotinamide was dissolved in normal physiological saline.
In vivo study
Induction of diabetes and determination of serum glucose
Diabetes was induced in the mice as follows; overnight fasted mice were treated with nicotinamide (110 mg/kg, i.p.) followed by streptozotocin (200 mg/kg, i.p.) 15 min later in all mice except the non-diabetic control (CitationBadole & Bodhankar, 2010). Animals were fed with glucose solution (5%) for 12 h to avoid hypoglycemia. Hyperglycemia was confirmed after 3 days. A steady state of hyperglycemia was reached after 10 days. Serum glucose was determined by the glucose oxidase peroxidase method. Mice having serum glucose between 200-300 mg/dL were selected for the study.
Effect of MUAI on serum glucose in streptozotocin-nicotinamide-induced diabetic mice
The mice were divided into the following groups (n = 6). Group I: control, II: diabetic control (administered with 2% starch solution), III: acarbose (50 mg/kg), IV: MUAI (1 mg/kg), V: MUAI (1 mg/kg)+2% starch solution. MUAI and acarbose were administered orally.
Chronic study involved repeated administration of acarbose, MUAI and MUAI+2% starch solution for 28 days (once a day) at predetermined time and serum glucose was determined in samples withdrawn after 6 h of acarbose, MUAI and MUAI+2% starch administration on day 7, 14, 21 and 28 (CitationBadole & Bodhankar, 2010). The data were represented as mean serum glucose levels and standard error of mean (SEM) were calculated.
Effect on body weight, food and water intake
During the study period of 28 days, the mice were weighed daily on an electronic balance (Kern EMB 220-1, Balingen, Germany). Food intake was determined by measuring the difference between the pre-weighed food and the weight of the remaining food in hopper and spilled food, after every 24 h. Water intake was measured by recording the quantity of water remaining in the feeding bottle. Body weight, food and water intake of mice were recorded daily but data is presented of days 0 (after 10 days injection of streptozotocin-nicotinamide), 7, 14, 21 and 28 only.
Biochemical parameters
On day 28, blood samples were collected by retro-orbital puncture technique using capillary tubes coated with disodium ethylenediamine tetraacetate (anti-coagulant). Biochemical parameters, i.e., cholesterol, triglycerides, high density lipoprotein, low density lipoprotein (lipid profile); aspartate aminotransferase (AST), alanine aminotransferase (ALT) were determined colorimetrically (Systronics spectrophotometer 104, Gujarat, India) by using the kits specific for the test.
Histopathology of vital organs
At the end of day 28, the animals were sacrificed after collecting blood for biochemical test under ether anesthesia. The pancreas, kidneys and liver were immediately removed. The isolated organs were trimmed into small pieces and preserved in 10% formalin for 24 h. Specimens were cut in sections of 35 µm thickness and stained by hematoxyline-eosin stain. The photomicrographs of each tissue section were observed using Nikon Eclipse 50i microscope (Nikon, Tokyo, Japan) with 40× magnification.
Effect of MUAI on oral glucose tolerance test (OGTT) in non-diabetic and diabetic mice
The non-diabetic and diabetic mice were divided into five groups (n = 6); group I control, group II diabetic control (administered with 2% starch solution), group III acarbose (50 mg/kg), group IV MUAI (1 mg/kg), group V MUAI+2% starch solution. MUAI and acarbose were given orally. D-glucose (2.5 g/kg, p.o.) was administered in non-diabetic as well as diabetic mice 4 h pretreatment with acarbose or MUAI. Serum glucose levels were estimated before and 2 h after glucose loading. The data were represented as mean serum glucose levels and standard errors of mean (SEM) were calculated.
In vitro study
Isolation of α-amylase from the pancreas of mice
Pancreas excised after sacrificing the animals under ether anesthesia were homogenized in 10 mM phosphate buffered saline of pH 7.4 (CitationAni & Naidu et al., 2008) containing protease inhibitor (10 mM). The homogenate was clarified by centrifugation (11,000 g) at 4°C for 15 min. The clear supernatant was applied to a Sephadex G-75 column equilibrated with saline. The fractions having maximum α-amylase activity were used for the kinetic studies.
Effect of MUAI on the pancreatic α-amylase activity
The inhibitory activity of MUAI on the mouse pancreatic α-amylase and human salivary α-amylase was studied essentially according to the procedure of CitationBernfeld (1955). 1.42 U of the mouse pancreatic or 1.47 U of human salivary α-amylase were preincubated with MUAI (0.1 mL, 100 μg) in buffer (10 mM phosphate buffer, pH 7.4) at 37°C for 60 min in a total reaction volume of 0.5 mL. α-Amylase reaction was initiated by the addition of 1% soluble starch. Controls without the inhibitor were run simultaneously. The reducing sugar was estimated using the dinitro salicylic acid (DNSA) method (CitationSadasivam & Manickam, 2005).
Enzyme kinetic studies on the inhibition of α-amylase by MUAI
α-Amylase (mouse pancreatic or human salivary) was incubated with MUAI in 10 mM phosphate buffer (pH 7.4) at 37°C for 1 h in a total reaction volume of 0.5 mL, before initiating the reaction with different concentrations of the substrate (0.54% starch solution). The 50% inhibition concentration (IC50) values were calculated. Lineweaver Burk plots were drawn and the values of Kiwere obtained from the plots.
Statistical analysis
Data were expressed as mean ± SEM. Statistical analysis was carried out by repeated measure ANOVA followed by post hoc Tukey test performed using GraphPad InStat version 3.00 for Windows Vista™ BASIC, GraphPad Software, San Diego, CA. P <0.05 was considered statistically significant.
Results
In vivo
Effect of MUAI on serum glucose in streptozotocin-nicotinamide induced diabetic mice
In the chronic study, daily administration of MUAI (1 mg/kg) and MUAI+2% starch once a day for 28 days caused a significant (P <0.001) reduction in the serum glucose level as compared to diabetic control mice. The difference in serum glucose before and after drug treatment was calculated. The reduction in serum glucose level after administration of acarbose, MUAI and MUAI+2% starch were 119.65, 61.64 and 181.64 mg/dL, respectively, on day 28 ().
Table 1. Effect of MUAI on serum glucose level, body weight, food and water intake in streptozotocin-nicotinamide induced diabetic mice.
Effect on body weight, food and water intake
The initial body weights were similar in control, diabetic control, acarbose, MUAI and MUAI+2% starch solution groups. Body weight of diabetic control mice decreased during the study period. Administration of MUAI and MUAI+2% starch solution significantly (P <0.01) prevented decrease in body weight (). Food and water intake were significantly (P <0.01) decreased in acarbose, MUAI and MUAI+2% starch solution treated mice as compared to diabetic control mice ().
Biochemical parameters
Serum total cholesterol was significantly (P <0.01) decreased in MUAI and MUAI+2% starch solution treated mice as compared to diabetic control mice (). The high density lipoprotein levels significantly (P <0.01) increased in MUAI and MUAI+2% starch solution treated mice as compared to diabetic control mice. However, triglycerides, low density lipoprotein and very low density lipoprotein levels in all the groups did not change significantly. No significant decrease was observed in the serum aspartate aminotransferase (AST) and alanine aminotransferase (ALT) levels in acarbose, MUAI and MUAI+2% starch solution treated mice with respect to diabetic control mice.
Table 2. Effect of MUAI on biochemical parameters in streptozotocin-nicotinamide induced diabetes in mice.
Histopathology of vital organs
Non-diabetic mice showed normal structure of pancreas () whereas decrease in the size of islets was observed in the diabetic control (). Acarbose (), MUAI () and MUAI+2% starch solution () treated mice showed normal sized islets.
Figure 1. Photomicrographs of the histology of the mice pancreas. (A) Non-diabetic; (B) Diabetic control; (C) Acarbose (50 mg/kg, p.o.); (D) MUAI (1 mg/kg, p.o.); (E) MUAI (1 mg/kg, p.o.) +2% starch solution.
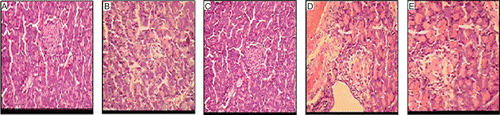
Non-diabetic mice showed normal structure of kidneys () whereas kidneys of diabetic control mice showed tubular dilation, interstitial inflammation, mesengial hyperplasia and glomerular basement membrane thickening (). Acarbose (), MUAI () and MUAI+2% starch () treated mice showed minimum pathological changes.
Figure 2. Photomicrographs of histology of mice kidney. (A) Non-diabetic; (B) Diabetic control; (C) Acarbose (50 mg/kg, p.o.); (D) MUAI (1 mg/kg, p.o.); (E) MUAI (1 mg/kg, p.o.)+2% starch solution.
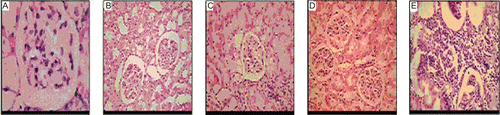
Non-diabetic mice showed normal structure of liver (). Moderate inflammation was observed in the liver of diabetic control mice () whereas acarbose (), MUAI () and MUAI+2% starch solution () treated mice showed mild lobular inflammation.
Effect of MUAI on OGTT in non-diabetic and diabetic mice
In OGTT, it was observed that the rise in the serum glucose level after 6 h was significantly (P< 0.001) lower in MUAI, MUAI+2% starch and acarbose treated mice as compared to the diabetic mice ( and ).
Table 3. Effect of MUAI on OGTT in non-diabetic mice.
Table 4. Effect of MUAI on OGTT in diabetic mice.
In vitro studies
Enzyme kinetic studies on the inhibition of pancreatic α-amylase by MUAI
It was observed from the Lineweaver Burke plot that MUAI inhibited both the mouse pancreatic α-amylase and human salivary α-amylase in a non-competitive manner with Ki values of 11 and 8.8 µM and having IC50 values of 30 and 12.5 µg/mL, respectively.
Discussion
Macrotyloma uniflorum has been traditionally used in the treatment of a number of disorders such as heart conditions, asthma, kidney stones, bronchitis, leukoderma, urinary discharges, obesity, etc. (CitationKawsar et al., 2009; CitationSiddhuraju & Manian, 2007). One of the major problems associated with diabetes is postprandial hyperglycemia. Currently a lot of attention is being given to diet therapy in the management of diabetes. Pulses have low glycemic index (GI) value which is important in the dietary treatment of diabetes mellitus (Rizkala et al., 2002).
In the present study we have evaluated the possible role of α-amylase inhibitor from Macrotyloma uniflorum seeds in the management of type II diabetes mellitus. Preliminary studies in our laboratory in streptozotocin-nicotinamide-induced diabetic mice showed that oral administration of MUAI at a single dose (1 mg/kg, p.o.) exhibited a maximum decrease in the serum glucose level at 6 h, without causing any toxic effects such as flatulence or diarrhea. Therefore the oral glucose tolerance test was designed in such a way that glucose load was administered 4 h after pre-treatment with MUAI and serum glucose level determined 2 h later. Pretreatment with MUAI for 4 h showed effective improvement in impaired glucose tolerance as compared to diabetic control without causing a hypoglycemic state.
MUAI plays a role in the carbohydrate metabolism, possibly by binding to the amylase, thereby causing a delay in carbohydrate digestion and absorption. MUAI when administered, in combination with a 2% starch solution, showed better activity than that of MUAI alone. A possible explanation for this may be that starch induces more amylase and therefore more inhibition of amylase occurs ().
Body weight in diabetic mice was decreased during the period of the study possibly due to catabolism of fats and protein. Due to insulin deficiency protein content is decreased in muscular tissues by proteolysis (CitationVats et al., 2004). Similar results were observed in acarbose, MUAI and MUAI+2% starch solution treated mice. It has been reported that regular use of α-glucosidase inhibitors tends to lower body weight (CitationTripathi, 2008). On the other hand, chronic treatment with MUAI and MUAI+2% starch solution prevented a decrease in body weight of the treated diabetic mice as compared to the diabetic control mice. Reduction in hyperglycemia prevented weight loss.
Non-diabetic mice showed slight fluctuation in food and water intake, while in diabetic control mice there was an increase in food and water intake. Acarbose, MUAI and MUAI+2% starch treated mice showed a decrease in food and water intake as compared to the diabetic control mice. The decrease in the food and water intake in the MUAI treated mice may be attributed to α-amylase inhibition. Proteinaceous α-amylase inhibitors produce satiety by delaying gastric emptying (CitationTormo et al., 2004). Pancreatic histology of diabetic control mice showed small size of the islets of Langerhans while acarbose, MUAI and MUAI+2% starch treated mice showed normal size of the islets which confirmed protection of pancreatic β cells from the toxic effect of streptozotocin.
Diabetes mellitus often involves abnormal lipid metabolism which causes complications in diabetic subjects (CitationKrentz, 2003). Hyperglycemia produces a marked increase in serum triglycerides and total cholesterol (CitationSaxena et al., 2005). Lipid abnormalities along with premature atherosclerosis are the major cause of cardiovascular diseases in diabetic patients. The ideal treatment for diabetes, in addition to glycemic control, should have a favorable effect on lipid profile (CitationKesari et al., 2007). In the present study, acarbose, MUAI and MUAI+2% starch combination not only lowered cholesterol level but also enhanced the cardioprotective lipid, i.e., high density lipoprotein after 28 days treatment which is well in agreement with the observation that long-term acarbose treatment in diabetic subjects reduces cardiovascular events (CitationTripathi, 2008). These studies further support the use of horse gram in traditional medicine for the control of obesity.
Administration of acarbose, MUAI and MUAI+2% starch combination had no significant effect on the levels of the serum aspartate aminotransferase and alanine aminotransferase. The histological study of liver of diabetic control mice showed moderate inflammation. Preservation of normal architecture of liver by acarbose, MUAI and MUAI+2% starch combination justify their beneficial effects on liver.
Toxic effects of streptozotocin are not restricted to pancreatic β cells but it also causes renal injury, oxidative stress inflammation and endothelial dysfunction (CitationValentovic et al., 2006). Streptozotocin-induced diabetic mice have increased renal sensitivity for ischemic injury, which is characterized by tubular atrophy or dilation (CitationMelin et al., 2002). Renal tubular dilation, interstitial inflammation, mesangial hyperplasia and glomerular basement membrane thickening were seen in the histology of kidneys of diabetic mice. Acarbose, MUAI and MUAI+2% starch treated mice showed normal kidney histology except for mild mesangial hyperplasia.
MUAI inhibited in vitro the mouse pancreatic and human salivary α-amylase in a non-competitive manner with low Ki values of 11, and 8.8 µM, respectively showing its high affinity for both the amylases. The present investigation clearly shows that oral administration of MUAI in diabetic mice reduces the serum glucose levels. This may be attributable to the inhibition of salivary and pancreatic α-amylase in vivo, which is in good agreement with the in vitro kinetic studies.
In conclusion, the results obtained demonstrated that MUAI acts as an antihyperglycemic agent in streptozotocin-nicotinamide induced diabetic mice. It also decreased the total serum cholesterol level, thereby confirming the use of horse gram seeds in traditional medicine to treat diabetes and obesity.
Acknowledgments
We are thankful to Sachin Vetale, Poona College of Pharmacy, Pune, for helping in carrying out the animal studies and Sujit Joshi, M.D. Pathologist, Specialty Surgical and Hematopathology Centre, Pune, for carrying out the histology of the vital organs.
Declaration of interest
The authors wish to thank University Grants Commission (UGC), New Delhi, India, for the award of research grant. The authors declare that there is no conflict of interest associated with this paper. The authors alone are responsible for the content and writing of the paper.
References
- Ali H, Houghton PJ, Soumyanath A. (2006). α-Amylase inhibitory activities of some Malaysian plants used to treat diabetes with particular references to Phyllanthus amarus. J Ethnopharmacol, 107, 449–455.
- Ani V, Naidu KA. (2008). Antihyperglycemic activity of polyphenolic components of black/bitter cumin Centratherum anthelminticum (L.) Kuntze seeds. Eur Food Res Technol, 226, 897–903.
- Badole SL, Bodhankar SL. (2010). Antidiabetic activity of cycloart-23-ene-3β, 25-diol (B2) isolated from Pongamia pinnata (L. Pierre) in streptozotocin-nicotinamide induced diabetic mice. Eur J Pharmacol, 632, 103–109.
- Bernfeld P. (1955). Amylases, alpha and beta. Meth Enzymol, 1, 149–158.
- Bravo L, Siddhuraju P, Calixto FS. (1998). Effect of various processing methods on the in vitro starch digestibility and resistant starch content of Indian pulses. J Agric Food Chem, 46, 4667–4674.
- Chinta S, Reddy PC, Sairanganayakulu G, Thippeswamy M, Reddy PS, Reddy MK. (2008). Identification of stress induced genes from the drought tolerant semi-arid legume crop horsegram (Macrotyloma uniflorum (Lam.) Verdc.) through analysis of subtracted expressed sequence tags. Plant Sci, 175, 372–384.
- Davis SN, Granner DK. (2001). Insulin, oral hypoglycemic agents and the pharmacology of endocrine pancreas. In: Gilman AG, Goodman LS, Hardman JG, Limbard LE, eds. The Pharmacological Basis of Therapeutics tenth edition. New York: McGraw Hill, 1701–1703.
- Kawsar SM, Golam M, Nahar N, Huq E. (2009). Chemical constitutions and hemolytic zctivity of Macrotyloma uniflorum Linn. Int J Nat Eng Sci, 3, 63–66.
- Kesari AN, Kesari S, Singh SK, Gupta RK, Watal G. (2007). Studies on the glycemic and lipidemic effect of Murraya koenigii in experimental animals. J Ethnopharmacol, 112, 305–311.
- Krentz AJ. (2003). Lipoprotein abnormalities and their consequences for patients with type 2 diabetes. Diab Obes Metab, 5, S19–S27.
- Melin J, Hellberg O, Larsson E, Zezina L, Fellstrom BC. (2002). Protective effect of insulin on ischemic renal injury in diabetes mellitus. Kidney Int, 61, 1383–1392.
- Puls W, Keup U. (1973). Influence of an α-amylase inhibitor (BAY d 7791) on blood glucose, serum insulin and NEFA in starch loading tests in rats, dogs and man. Diabetologia, 9, 97–101.
- Rizkalla SW, Bellisle F, Slama G. (2002). Health benefits of low glycaemic index foods, such as pulses, in diabetic patients and healthy individuals. British J Nutr, 88, 255–262.
- Sadasivam S, Manickam A. (2005). Estimation of reducing sugar by dinitrosalicylic acid method. In: Biochemical Methods, second edition. New Delhi, India: New Age International, 6–7.
- Saxena R, Madhu V, Shukla R, Prabhu KM, Gambhir JK. (2005). Postprandial hypertriglyceridemia and oxidative stress in patients of type 2 diabetes mellitus with macrovascular complications. Clin Chim Acta, 359, 101–108.
- Shim YJ, Doe HK, Ahn SY, Kim YS, Seong JK, Park IS, Kim BH. (2003). Inhibitory effect of aqueous extract from the gall of Rhus chinensis on α glucosidase activity and postprandial blood glucose. J Ethnopharmacol, 85, 283–287.
- Siddhuraju P, Manian S. (2007). The antioxidant activity and free radical scavenging capacity of dietary phenolic extracts from horse gram (Macrotyloma uniflorum (Lam.) Verdc.) seeds. Food Chem, 105, 950–958.
- Subramanian R, Asmawi MZ, Sadikun A. (2008). In vitro α-glucosidase and α-amylase enzyme inhibitory effects of Andrographis paniculata extract and andrographolide. Acta Biochim Pol, 55, 391–398.
- Tormo MA, Gil Exojo I, Romero de Tejada A, Campillo JE. (2004). Hypoglycemic and anorexigenic activities of an α-amylase inhibitor from white kidney beans (Phaseolus vulgaris) in Wistar rats. Br J Nutr, 92, 785–790.
- Tripathi KD. (2008). Insulin, oral hypoglycemic drugs and glucagon. In: Essentials of Medical Pharmacology, sixth edition. New Delhi, India: Jaypee, Bros., Medical Publishers, 254–274.
- Valentovic MA, Alejandro N, Betts CA, Brown PI, Ramos K. (2006). Streptozotocin (STZ) diabetes enhances benzo(α)pyrene induced renal injury in Sprague Dawley rats. Toxicol Lett 164, 214–220.
- Vats V, Yadav SP, Grover JK. (2004). Ethanolic extract of Ocimum sanctum leaves partially attenuates streptozotocin induced alterations in glycogen content and carbohydrate metabolism in rats. J Ethnopharmacol, 90, 155–160.