Abstract
Context: Various Ocimum species (Labiateae) are commonly used for the treatment of inflammation, stress, diarrhea, and as an antioxidant drug in the Indian ethnic system of medicine.
Objective: The present study was carried out to investigate the antimelanoma and radioprotective activity of different species of Ocimum in C57BL and Swiss albino mice.
Materials and methods: The antimelanoma activity of 50% alcoholic aqueous leaf extract of five species of Ocimum [Ocimum sanctum (SE), Ocimum gratissimum (GE), Ocimum basilicum (BE), Ocimum canum (CE), and Ocimum kilimandscharicum (KE)] alone or in combination with radiotherapy was determined on the basis of tumor volume, body weight, and survival rate of animals. The radioprotective potential of different species of Ocimum was determined by chromosomal aberration assay. The effect of the alcoholic aqueous extract of different species of Ocimum was also evaluated for the estimation of glutathione level and glutathione S-transferase activity in Swiss albino mice.
Results: The 50% alcoholic aqueous extract of different species of Ocimum administered orally (200 mg/kg, p.o.) resulted in significant reduction in tumor volume, increase in average body weight, and survival rate of mice. The various extracts showed modulatory influence against lethal irradiation doses of gamma radiation in terms of radiation-induced chromosomal damage, while at the same time induced an increase in reduced glutathione level and GST activity.
Discussion and conclusion: These findings demonstrate that Ocimum species have antimelanoma and radioprotective activity against B16F10 metastatic melanoma cell line-induced metastasis and could be exploited as one of the potential sources for plant-based pharmaceutical products.
Introduction
Melanoma represents a significant worldwide public health risk and, from the standpoint of incidence, is the fastest growing of all cancer types. Malignant melanoma is the most aggressive form of skin cancer, with a mortality rate that has risen about 2% annually since 1960. Although early stage melanoma can be cured surgically, once melanoma metastasizes to major organs (stage IV), it is almost always incurable (CitationRigel & Carucci, 2000). There are few chemotherapeutic agents available for treating metastatic melanoma, and those that have been used have all yielded unsatisfactory results. No single chemotherapeutic agent currently offers a response rate greater than 25%, and treatment is invariably accompanied by significant side effects, including myelosuppression, nausea, and emesis (CitationThompson et al., 2005; CitationSatzger et al., 2007; CitationSmalley & Herlyn, 2009). There is no standard treatment for patients with metastatic melanoma. The combination of radiotherapy (RT) and chemotherapy is an appealing approach that has led to improved treatment in patients with advanced solid tumors because of its independent cell-killing effect. However, rising recurrence rate, acute toxicity, side effects coupled with an absence of effective adjuvant therapy highlight the absolute requirement for future research into this cancer type along with development of new agents with least toxicity. The success of antimetastatic therapy depends on environment while limiting overall toxicity, and therefore it has been suggested that adjuvant therapy may be more effective than monotherapies (CitationPooja et al., 2007).
Medicinal plants serve as a good source of pharmacological compounds and plant-based medicinal practices have effectively been used in indigenous systems of medicine from time immemorial. One such genus, Ocimum (Labiateae), commonly known as tulsi, is available throughout India and is well known for its medicinal properties (CitationUma Devi et al., 2000). It has been shown to have analgesic, anti-inflammatory (CitationGodhwani et al., 1987), antistress (CitationBhargava & Singh, 1981), wound healing (CitationSomashekar et al., 2006), and antidiarrheal properties (CitationEl-said et al., 1969). The extract of this plant had been shown to exhibit anticarcinogenic activity against 7,12-dimethylbenzanthracene-induced skin papilloma in male Swiss albino mice (CitationPrashar et al., 1994). Ocimum extract has been found to have antioxidant activity (CitationGanasoundari et al., 1997), which may play an important role in cancer prevention. Considering the pronounced antioxidant and anticarcinogenic activity, the 50% alcoholic aqueous leaf extract of different species of Ocimum (OE) alone or in combination with RT was evaluated against B16F10 melanoma cell line-induced metastasis in C57BL mice.
Materials and methods
Plant material and preparation of leaf extract
The leaves of five different species of Ocimum: Ocimum sanctum (SE), Ocimum gratissimum (GE), Ocimum basilicum (BE), Ocimum canum (CE), and Ocimum kilimandscharicum (KE) were collected in September 2008 from the Herbal Garden, Jawaharlal Nehru Cancer Hospital and Research Center, Bhopal, India. The leaves were shade-dried and powdered. A voucher specimen was deposited in the herbarium at the Jawaharlal Nehru Cancer Hospital and Research Center (OC: 004-09). The leaves of all species of Ocimum were air-dried and then reduced to powder with mortar and pestles. The powder (200 g) of each species was extracted with maceration at 90°C with 4 L mixture of methanol:distilled water (50:50). The macerated mixture was filtered through muslin cloth and the solvent was removed under reduced pressure (p = 120 mm Hg) to yield 13 g of dark solid extract. The extract was stored at a temperature of −20°C pending the time for biological investigations. All residues were dissolved in olive oil just before administration and screened for their antimelanoma and radioprotective activity on B16F10-induced skin cancer in C57BL mice.
Animals and chemicals
Random bred, male C57BL and Swiss albino mice, 6–8 weeks old with body weights of 24 ± 2 g, were obtained from the Animal House of Jawaharlal Nehru Cancer Hospital and Research Center, Bhopal, and kept in the departmental animal rooms with controlled temperature of 23 ± 5°C, 60 ± 5% humidity and with a 12-h light–dark cycle. They were fed a standard diet and water. The mice were acclimatized for 1 week before experimentation. The animal care and handling was done according to the guidelines set by the World Health Organization (WHO), Geneva, Switzerland, and the Indian National Science Academy (INSA), New Delhi, India. Necessary approvals were obtained from the Institutional Animal Ethical Committee (CPCSEA registration no. 500/01/a/CPCSEA/2001, reference no. 207/225-IAEC/2008, project no. 54). 1-Chloro-2,4-dinitrobenzene (CDNB), 5-dithio-bis-2-nitrobenzoic acid (DTNB), reduced glutathione, and bovine serum albumin (BSA) were procured from Sigma, St. Louis, MO. All other required chemicals were of highest purity and obtained from local suppliers.
Cell culture
B16F10, a highly metastatic skin selected subline derived from C57BL murine melanoma (CitationFidler, 1973), was purchased from the National Center for Cell Science, Pune, India. The cell line was maintained as a continuous culture in DMEM (Hi-Media, Mumbai, India) supplemented with 10% fetal bovine serum (Gibco, Grand Island, NY), 100 units/mL penicillin, and 100 µg/mL streptomycin. Cells were grown in a humidified atmosphere of 5% CO2 and 95% air at 37°C. Media was replenished every third day.
Radioprotection
Mice were irradiated by 60Co source in the cobalt teletherapy unit (Siemens, Munich, Germany) at the Radiation Oncology Department, Jawaharlal Nehru Cancer Hospital and Research Center, Bhopal, India, with an isocentric three-beam technique (CitationGunilla et al., 2003). The source to skin distance (SSD) was 80 cm with irradiation time 1.89 min. RT was given simultaneously with 2 Gy fractions in 2 days to a dose of 4 Gy to a total dose in the three courses of 12 Gy.
Chromosomal aberration assay
The radioprotective effect of OE was investigated by taking chromosome aberrations as the end point. The method of CitationGanasoundari et al. (1997) was followed for this activity. Metaphase plates were prepared from femur marrow on Day 20 and the frequency of aberrant cells and individual aberrations, such as ring (r), fragment (frg), deletion (del), acrocentric association (ACA), pulverization (PVZ), translocation (t), minute (M), acentric fragment (AF), dicentric (DC), and break (Brk), were scored.
Experimental design
Different extracts were given orally daily for 20 days. RT was given on Days 4, 5, 11, 12, 18, and 19, each time at 2 Gy up to a total of 12 Gy (CitationFrykholm et al., 1989). The radiation treatment was repeated every week (). Mice were shaved on the vertical side and challenged intradermally with B16F10 cells (2 × 105/mL) in phosphate-buffered saline (PBS). Animals were randomly assigned to six groups (10 mice/group). Group I: Olive oil (50 µL/mouse) was given orally throughout the experiment. Group II: B16f10 cells (2 × 105/mL) in PBS were injected intradermally. Each mouse was kept in conditions as mentioned earlier. In addition to standard diet, mice were given olive oil orally. Group III: The melanoma cells (2 × 105/mL) were injected intradermally. Along with standard diet mice were orally given a dose of 200 mg/kg body weight of 50% OE in 50 µL olive oil. Group IV: RT was given with the dose and schedule shown in . Each mouse was kept in conditions as mentioned earlier. In addition to standard diet, mice were given olive oil orally. Group V: The melanoma cells (2 × 105/mL) were injected intradermally. Each mouse was subjected to γ-radiation as shown in . Along with standard diet, mice were given olive oil orally. Group VI: The melanoma cells (2 × 105/mL) were injected intradermally. Each mouse was subjected to γ-radiation as prescribed (). Along with standard diet, mice were given orally a dose of 200 mg/kg body weight (bw) of OE in 50 µL olive oil. Grouping of animals was same for all species of Ocimum. Every alternate day, tumor volume (TV) was measured with the help of vernier calipers. The body weight and survival rate of mice were recorded twice a week. TV was calculated by the formula:
The length is a, and b is the breadth of tumor implant (in mm). TVs were then converted into relative tumor volume (RTV) (CitationSabatino et al., 2003) using the formula:
TVo is the tumor volume at Day 1 and TVx is the tumor volume on the respective day.
Morphological observations of tumor
The TV, body weight, and number of animals that survived the 20-day period were recorded. Mice were sacrificed by cervical dislocation at the termination of experiment. The skin tumor was excised and prepared for histopathological examination by immersion fixation in 10% neutral buffered formalin for several days at room temperature. Fixed tissues were processed for paraffin-wax embedding, sectioned 4–6 µm thick, stained with hematoxylin and eosin (H&E), and evaluated under light microscope.
Estimation of reduced glutathione and glutathione S-transferase activity
Animals were randomly assigned to seven groups (10 mice/group): group 1, olive oil (50 µL/mouse) was given orally throughout the experiment; group 2 received 0.75% butylated hydroxyanisole (BHA) diet orally throughout the experiment; group 3, along with normal diet mice were orally given a dose of 200 mg/kg bw of SE in 50 µL olive oil; group 4, along with normal diet mice were orally given a dose of 200 mg/kg bw of GE in 50 µL olive oil; group 5, along with normal diet mice were orally given a dose of 200 mg/kg bw of BE in 50 µL olive oil; group 6, along with normal diet mice were orally given a dose of 200 mg/kg bw of CE in 50 µL olive oil; group 7, along with normal diet mice were orally given a dose of 200 mg/kg bw of KE in 50 µL olive oil.
Preparation of homogenates and supernatant fraction of liver, lung, and stomach
The animals were killed by cervical dislocation after 15 days treatment. The liver was perfused in situ immediately with cold 0.9% NaCl and thereafter excised, and rinsed in chilled 0.15 M Tris–KCl (pH 7.4). The liver was then blotted dry, weighed, and homogenized in ice-cold 0.15 M Tris–KCl buffer (pH 7.4) to yield a 10% (w/v) homogenate. Aliquots (0.5 mL) of this homogenate were used for assaying reduced glutathione, whereas the remainder was centrifuged at 10,000 g for 20 min. The resulting supernatant was transferred into pre-cooled polypropylene centrifugation tubes and centrifuged again at 15,000 g for 30 min in a high-speed refrigerated centrifuge (L5B, DuPont, Tonawanda, NY). The supernatant fraction after discarding any floating lipid layer was used for assaying glutathione-S-transferase enzyme activity. The lung and stomach were removed and rinsed in chilled 0.15 M Tris–KCl (pH 7.4). The stomach was slit open longitudinally, cleaned, and flushed with the buffer 6–8 times. The lung was cut into small pieces. The lung and stomach were then blotted dry, weighed, and homogenized in ice-cold 0.15 M Tris–KCl buffer (pH 7.4) to yield a 10% (w/v) homogenate. Aliquots of 0.5 mL of this homogenate were used for assaying reduced glutathione, whereas the remainder was centrifuged at 800 g for 10 min. The resulting supernatant was transferred into pre-cooled polypropylene centrifugation tubes and centrifuged at 15,000 g for 30 min in a high-speed refrigerated centrifuge (DuPont). The supernatant fraction, appropriately diluted, was used for assaying glutathione S-transferase activity.
Assay methods
The cytosolic or supernatant glutathione S-transferase activity was determined spectrophotometrically at 37°C (CitationHabig et al., 1974). The reaction mixture (3 mL) contained 1.7 mL of 100 mM phosphate buffer (pH 6.5) and 0.1 mL of 30 mM CDNB. After pre-incubating the reaction mixture at 37°C for 5 min, the reaction was started by the addition of 0.1 mL diluted cytosol or supernatant, and the absorbance was recorded at 340 nm. Reaction mixture without the enzyme was used as control. The specific activity of glutathione S-transferase is expressed as µmol of GSH–CDNB conjugate formed per min per mg protein using an extinction coefficient of 9.6 mM−1cm−1. The protein content was measured according to Bradford’s method using BSA as standard (CitationBradford, 1976). Tissue levels of reduced glutathione were determined by the method described by CitationMoron et al. (1979). Homogenates were immediately precipitated with 0.1 mL volume of 25% trichloroacetic acid and the precipitate was removed after centrifugation (10,000 g). Free SH group was assayed in a total 3 mL volume by the addition of 2 mL of 0.6 mM DTNB prepared in 0.2 M phosphate buffer (pH 8), to 0.1 mL of the supernatant and then reading the absorbance at 412 nm. Glutathione was used as standard to calculate µmol GSH/g tissue.
Statistical analysis
Data obtained are presented as means ± standard error of mean (SEM) for the number of animals in each group (n = 10). The differences between the data obtained from test animal groups and the data obtained from control animal groups were subjected to one-way analysis of variance (ANOVA; 95% confidence interval), followed by Dunnett’s test. Values of P <0.05 compared with the control group were considered as being significantly different.
Results
There was significant reduction in TV of mice treated with different species of Ocimum. At the end of the study (20 days), the TV of the control group (group II) increased 15-fold; however, in group IV it increased 3.5-fold. TV increase of the SE-treated group was significantly lower (1.34-fold) in comparison with group II, whereas it was 2.21-, 2.51-, 4-, and 8-folds for the groups treated with GE, BE, CE, and KE, respectively. When compared with group IV, TV increase was 0.53-, 0.70-, 0.94-, 1.64-, and 2.34-folds for the groups treated with SE, GE, BE, CE, and KE, respectively (). The average body weight of B16F10 cell-treated mice did not differ from that of the vehicle-treated mice throughout the study. However, there was a slight increase in the average body weight of OE-treated mice at the termination of the study (). The effect of different species of Ocimum on the survival of tumor-bearing mice is shown in . The survival rate of mice decreased significantly in B16F10 cell line-treated mice compared with the vehicle-treated group. The survival rate of the vehicle-treated group was 100%, whereas it was 40% for B16F10-treated group. Survival of the SE-treated group was significantly higher (90%) in comparison with the B16F10-treated group, whereas it was 80, 70, 70, and 60% for the groups treated with GE, BE, CE, and KE, respectively.
Figure 2. Comparison between the extracts of different species of Ocimum against tumor volume; aP < 0.001, bP < 0.01, cP < 0.05, dP < 0.07 versus B16F10 alone. SE, Ocimum sanctum extract; GE, Ocimum gratissimum extract; BE, Ocimum basilicum extract; CE, Ocimum canum extract; and KE, Ocimum kilimandscharicum extract.
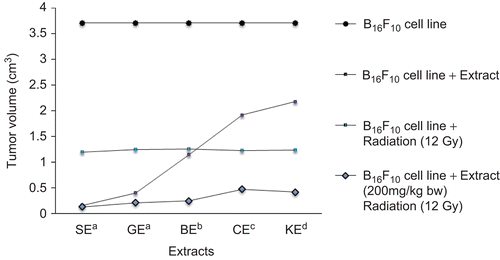
Figure 3. Effect of extracts of Ocimum species on body weight of mice; *P < 0.01 versus B16F10 alone. SE, Ocimum sanctum extract; GE, Ocimum gratissimum extract; BE, Ocimum basilicum extract; CE, Ocimum canum extract; and KE, Ocimum kilimandscharicum extract.
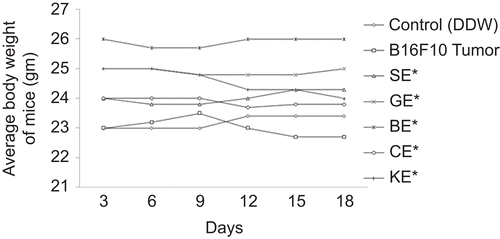
Figure 4. Effect of extracts of Ocimum species on survival rate of mice; *P <0.01 versus B16F10 alone. SE, Ocimum sanctum extract; GE, Ocimum gratissimum extract; BE, Ocimum basilicum extract; CE, Ocimum canum extract; and KE, Ocimum kilimandscharicum extract.
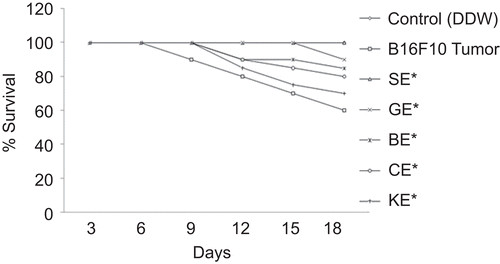
Modulatory influence of OE was also observed against lethal irradiation dose of γ-radiation in terms of radiation-induced chromosomal damage (). A total of 500 metaphases were counted for each group. All the metaphases were found to be normal in the control group (). In the radiation control group, the percentage of aberrant metaphases was found to be 87.13%. The mice receiving a radiation dose of 12 Gy along with dose of 200 mg/kg bw of SE showed maximum radioprotection (65.2%). SE showed total absence of ACA, PVZ, AF, and double minute. However, there was a reduction in r, del, and frg. On the other hand, BE and GE showed good radiation protection in terms of genomic stability. They showed maximum chromosomal protection of 58.6 and 53.5%, respectively. When BE was subjected to 12 Gy radiation, it showed complete elimination of PVZ and AF, whereas r, frg, del, ACA, and M are being reduced to 1/4. GE also showed a total absence of AF, DC, Brk, and translocation. Radiation significantly increased the percentage of aberrant cells, along with all types of aberration. Treatment with CE and KE showed partial protection to bone marrow, that is, 36.1 and 32.8%, respectively. No complex aberrations such as AF, DC were noted.
Table 1. Percentage of aberrant cells (mean ± SE) in mouse bone marrow after treatment with different species of Ocimum, each group of 10 animals.
Figure 5. Plates showing metaphase. (A) Control group showing normal metaphase. (B) Radiation control plate showing pulverization, minute, and fragment. (C) SE (200 mg)/kg) + RT (12 Gy)-treated group showing ring. (D) BE (200 mg/kg) + RT (12 Gy)-treated group showing acrocentric association (ACA). (E) GE (200 mg/kg) + RT (12 Gy)-treated group showing minute (M) and ring (r). (F) CE (200 mg/kg) + RT (12 Gy)-treated group showing acrocentric association (ACA), fragment (Frg), and deletion (del). (G) KE (200 mg/kg RT (12 Gy)-treated group showing acrocentric association (ACA), fragment (frg), and minute (M).
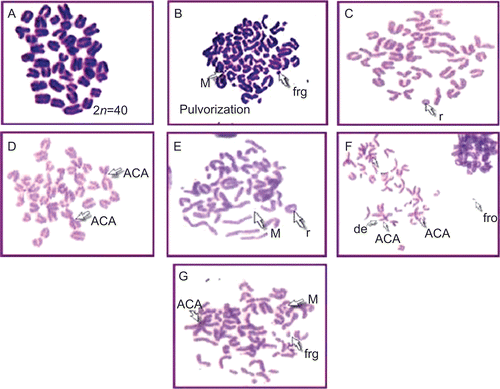
All the investigated extracts of Ocimum species exhibit significant increase in reduced glutathione level and significant elevation in GST enzyme activity in the liver, lung, and stomach of Swiss albino mice over that of vehicle-treated control animals (group 1). Mice that were treated with SE (group 3, 200 mg/kg bw) showed significant elevation in GST enzyme activity. This increase was 2.48-fold in the liver (P < 0.001), 1.4-fold in the lung (P < 0.01), and 1.46-fold in the stomach (P < 0.001) of mice. Animals treated with GE (group 4, 200 mg/kg bw) showed a 2.3-fold increase in GST enzyme activity in the liver (P < 0.001), 1.4-fold in the lung (P < 0.01), and 1.4-fold in the stomach (P < 0.001) of mice. Mice that were fed with BE (group 5, 200 mg/kg bw) also revealed significant elevation in GST activity. The increase was 2.3-fold in the liver (P <0.001), 1.48-fold in the lung (P < 0.01), and 1.45-fold in the stomach (P < 0.001) of mice. Animals treated with CE (200 mg/kg bw) and KE (200 mg/kg bw), groups 6 and 7, exhibited significant increase in GST activity. In the CE-treated group, this increase was 2.21-fold in the liver (P < 0.001), 1.4-fold in the lung (P < 0.01), and 1.44-fold in the stomach (P < 0.001), and 2.11-fold in the liver (P < 0.001), 1.2-fold in the lung (P < 0.01), and 1.42-fold in the stomach (P < 0.001) in the KE-treated group of mice. Animals that were fed with the diet containing BHA for 15 days (group 2) also showed significant elevation in GST activity. The increase was 4.23-fold (P < 0.05) in the liver, 1.23-fold (P < 0.05) in the lung, and 1.9-fold (P < 0.01) in the stomach ().
Table 2. Effect of oral feeding of different species of Ocimum leaf extract on reduced glutathione and glutathione S-transferase enzyme activities in mouse liver, stomach and lungs.
The reduced glutathione level on treatment with 200 mg/kg bw of SE for 15 days (group 3) was found to be elevated 1.79-fold (P < 0.001) in the liver, 1.76-fold (P < 0.001) in the lung, and 1.74-fold (P < 0.01) in the stomach, when compared with group 1. In GE-treated mice (group 4), the glutathione level was elevated 1.43-fold (P < 0.01) in the liver, 1.56-fold in the lung (P < 0.01), and 1.49-fold in the stomach. The basal reduced glutathione level was increased 1.63-fold (P < 0.001) in the liver, 1.54-fold (P < 0.01) in the lung, and 1.59-fold (P < 0.001) with the CE-treated group of mice (group 6). Mice that were treated with KE (group 7, 200 mg/kg bw) revealed significant elevation in reduced glutathione level. This increase was 1.36-fold (P < 0.05) in the liver, 1.39-fold (P < 0.01) in the lung, and 1.49-fold (P < 0.01) in the stomach. In BHA-treated mice (group 2), the glutathione level was elevated 1.48-fold (P < 0.01) in liver, 1.94-fold (P < 0.001) in the lung, and 1.41-fold (P < 0.05) in the stomach compared with group I ().
Discussion
Murine-type tumors are common malignant tumors, and primary therapy for these tumors includes surgery, RT, and chemotherapy. The success of these therapies is greatly reduced due to their side effects, and there is needed not only to explore new molecules but also for adjuvant therapy that can reduce these side effects. Reduction in TV and increase in survival rate are two important parameters for any anticancer therapy. The oral administration of the different species of Ocimum extract showed a significant reduction in TV, tumor incidence, and a significant increase in the survival rate. However, it was observed that SE has more chemopreventive and radioprotective potential than GE, BE, CE, and KE at same concentration in B16F10 melanoma skin cancer in C57BL mice. In the SE-treated group, more reduction in tumor incidence and TV was observed than other species of Ocimum. The survival rate was significantly increased for the SE-treated group. These effects could be associated with the low tumor burden as a result of the inhibitory effect of Ocimum extracts on carcinogenesis.
To reduce side effects, radioprotection is required among cancer patients receiving RT. The main radioprotective class is the thiol synthetic compounds such as amifostine. Amifostine is a powerful radioprotective agent compared with other agents, but this drug is limited in its use in clinical practice due to its own side effects and toxicity (CitationUsseinimehr et al., 2001, Citation2002). The search for less toxic radiation protectors has spurred interest in natural products. The oral administration of OE at a dose of 200 mg/kg bw was found to reduce chromosomal aberrations induced by γ-radiation significantly. Radiation-induced DNA damage is expressed as single-strand breaks (SSBs), double-strand breaks (DSBs), base/liberation, base damage, inter-strand cross-links, and DNA–protein cross-links (CitationWard, 1975). DSBs are widely believed to be biologically more relevant than SSBs, and they have been implicated as the critical event in radiation-induced cell killing (CitationRadford, 1985; CitationWard, 1986). The DNA DSBs induced by X-rays are repaired by the time these cells reach the first post-irradiation mitosis. However, the repair is error-prone, leading to structural chromosomal aberrations (CitationLliakis et al., 1987). Experimental evidence suggests that DNA DSBs are among the primary lesions responsible for the induction of chromosome aberrations and cell death in mammalian cells (CitationBryant, 1985, Citation1988). Various types of chromosomal damage such as DCs, r, ACA, PVZ, M, frg were observed at dose 12 Gy. OE pretreatment significantly reduced the exchange and multiple aberrations in comparison with RT-alone-exposed groups, demonstrating protection against DSBs and multiple lesions. SE showed maximum protection in terms of chromosomal aberration followed by BE, GE, CE, and KE. The decrease is proportional to the radiation damage at a given dose suggesting that OE protection may be at the primary level of induction of DNA lesion. The radioprotection activity of OE may be attributed to free radical scavenging and repair of radiation damage via GSH. The maximum bone marrow protection in term of chromosomal aberration is shown by SE followed by BE, GE, CE, and KE. Since significant protection is obtained at a nontoxic dose (200 mg/kg bw), the extract may have an advantage over the known radioprotectors available so far.
Many plant materials are known to influence the hepatic biotransformation enzyme profiles that are involved in activation and detoxification of xenobiotic chemicals including chemical carcinogens (CitationWattenberg, 1983; CitationColditz et al., 1985). The CDNB was used as a nonspecific substrate for assaying total cytosolic GST, and it was noticed that feeding the extract of different species of Ocimum leaf through gastric intubation for 15 days significantly enhanced the GST activity in the liver, lung, and stomach of mice. The Swiss albino mice also responded to the induction of glutathione S-transferase by BHA, a synthetic antioxidant, which has been shown to have protective effect against tumor induction by chemical carcinogens of diverse nature (CitationHocman, 1988). The elevation of cytosolic GST implies a protective role against the cytotoxic or carcinogenic effect of many compounds, which are activated by the biotransformation enzymes of the endoplasmic reticulum, by scavenging the electrophilic metabolites (CitationChasseud, 1979; CitationColes & Ketterer, 1990; CitationDeflora & Ramel, 1998). The various extracts of Ocimum (SE, GE, BE, CE, and KE) induced increase in GST activity, which may provide protection against carcinogenicity or genotoxicity mediated by electrophilic metabolites. The protective effect of BHA has been attributed to lowering of the intracellular concentration of biologically reactive intermediates through elevation of glutathione S-transferase enzyme activity and intracellular glutathione concentration (CitationBenson et al., 1978; CitationCha & Beuding, 1979). Similar to BHA, the present study also demonstrates that feeding of Ocimum extract could elevate significantly the level of SH group in the hepatic and extrahepatic tissues of mice. Cellular acid soluble SH content, which almost entirely consists of glutathione, functions in protecting the cells against free radicals generated during oxidative metabolism as well as from cellular lethality following exposure to drugs or radiation and acting as acceptor of electrophilic molecules involved in cancer initiation (CitationOrrneius & Moldeus, 1984; CitationKetterer, 1998). Thus, Ocimum leaf extract presumably renders the mice resistant to harmful oxygen species and chemical carcinogenic action. Hence, from a mechanistic point of view, it is possible that Ocimum extract may be accelerating detoxification of carcinogens in the hepatic and extrahepatic tissues of mice by increasing the GST activity. The different species of Ocimum increased the reduced glutathione level and increased glutathione S-transferase. The increased glutathione reductase level plays a significant role in the reduction of oxidized glutathione to reduced glutathione at the expense of NADPH and regulates the GSH–GSSG cycle in the cell (CitationGonzales et al., 1984). GSH has been endowed with an important function in maintaining the reduced state of cellular environment, in addition to its conjugating ability owing to nucleophilic center and its involvement in detoxification of xenobiotics that cause toxicity and carcinogenicity.
Conclusion
The alcoholic aqueous extract of various species of Ocimum showed marked decrease in TV and incidence against B16F10, metastatic melanoma cell-induced metastasis in C57BL mice. Modulatory influence of OE was also observed against lethal irradiation dose of gamma radiation in terms of radiation-induced chromosomal damage. The radioprotection activity of OE may be attributed to free radical scavenging and repair of radiation damage via GSH. The results suggest further research on bioactive components of this plant and in-depth mechanism study.
Acknowledgements
The authors are grateful to Shri Madan Mohan Joshi, Chairman of the Jawaharlal Nehru Cancer Hospital and Research Center, Bhopal, for providing facilities to carry out the work. Sanjeev Vashistha and Nancy, Department of Radiation Biology and Radioprotection, are acknowledged gratefully for irradiation facilities and dosimetric calculations.
Declaration of interest
The authors report no conflicts of interest. The authors alone are responsible for the content and writing of the paper.
References
- Benson AM, Batzinger RP, Ou SL, Bueding E, Cha YN, Talalay P. (1978). Elevation of hepatic glutathione-S-transferase activities and protection against mutagenic metabolites of benzo(a)pyrene by dietary antioxidants. Cancer Res, 38, 4486–4495.
- Bhargava KP, Singh N. (1981). Anti-stress activity of Ocimum sanctum Linn. Indian J Med Res, 73, 443–451.
- Bradford MM. (1976). A rapid and sensitive method for the quantification of microgram quantities of protein utilizing the principle of protein–dye binding. Anal Biochem, 72, 248–254.
- Bryant PE. (1985). Enzymatic restriction of mammalian cells DNA: evidence for the double-strand breaks as potentially lethal lesions. Int J Radiat Biol, 48, 55–60.
- Bryant PE. (1988). Use of restriction endonucleases to study relationship between DNA double-strand breaks, chromosomal aberrations and other end points in mammalian cells. Int J Radiat Biol, 54, 869–890.
- Cha YN, Beuding E. (1979). Effect of 2(3)-tert-butyl-4-hydroxyanisole administration on the activities of several hepatic microsomal and cytoplasmic enzymes in mice. Biochem Pharmacol, 28, 1917–1921.
- Chasseud, LF. (1979). The role of glutathione and glutathione-S-transferases in the metabolism of chemical carcinogen and other electrophilic agents. Adv Cancer Res, 29, 175–274.
- Colditz GA, Branch LG, Lipnick RJ, Willett WC, Rosner B, Posner BM, Hennekens CH. (1985). Increased green and yellow vegetable intake and lowered cancer deaths in an elderly population. Am J Clin Nutr, 41, 32–36.
- Coles B, Ketterer B. (1990). The role of glutathione and glutathione transferases in chemical carcinogenesis. Biochem Mol Biol Int, 25, 47–70.
- Deflora S, Ramel C. (1998). Mechanisms of inhibitors of mutagenesis and carcinogenesis: Classification and overview. Mutat Res, 202, 285–306.
- El-said F, Sofowora EA, Malcolm SA, Hofer A. (1969). An investigation into the efficacy of Ocimum gratissimum as used in Nigerian native medicine. Planta Med, 17, 195–200.
- Fidler IJ. (1973). Selection of successive tumor lines for metastasis. Nature New Biol, 242, 148–149.
- Frykholm G, Glimelius B, Påhlman L. (1989). Preoperative irradiation with and without chemotherapy (MFL) in the treatment of primary non-resettable adenocarcinoma of the rectum: results from two consecutive studies. Eur J Cancer Clin Oncol, 25, 1535–1541.
- Ganasoundari A, Uma Devi P, Rao MNA. (1997). Protection against radiation-induced chromosome damage in mouse bone marrow by Ocimum sanctum. Mutat Res, 373, 271–276.
- Godhwani S, Godhwani JL, Vyas DS. (1987). Ocimum sanctum an experimental study evaluating its anti-inflammatory, analgesic and antipyretic activity in animals. J Ethnopharmacol, 21, 153–163.
- Gonzales R, Auclain C, Voisin E, Gautero H, Dhermy D, Boivin P. (1984). Superoxide dismutase, catalase, and glutathione peroxidase in red blood cells from patients with malignant diseases. Cancer Res, 44, 4137–4139.
- Gunilla JF, Pahlman L, Glimelius B. (2003). Combined chemo- and radiotherapy versus radiotherapy alone in the treatment of primary, non-resettable adenocarcinoma of the rectum. Int J Radiat Oncol Biol Phys, 50, 427–434.
- Habig WH, Pabst MJ, Jakoby WB. (1974). The first enzymatic step in mercapturic acid formation. J Biol Chem, 249, 7130–7139.
- Hocman G. (1988). Chemoprevention of cancer: Phenolic antioxidants (BHT, BHA). Int J Biochem, 7, 639–651.
- Ketterer B. (1998). Glutathione-S-transferase and prevention of cellular free radical damage. Free Radic Res, 28, 647–658.
- Lliakis G, Pantelias GE, Okayasu R, Seaner R. (1987). 125IdUrd-induced chromosome fragments, assayed by premature chromosome condensation and DNA double-strand breaks have similar repair kinetics in G1-phase CHO cells. Int J Radiat Biol, 52, 705–722.
- Moron MS, Depierre JW, Mannervik B. (1979). Levels of glutathione, glutathione reductase and glutathione-S-transferase activities in rat lung and liver. Biochim Biophys Acta, 582, 67–78.
- Orrneius S, Moldeus P. (1984). The multiple roles of glutathione in drug metabolism. Trends Pharmacol Sci, 5, 432–435.
- Pooja D, Arvind I, Gude P. (2007). Suramin augment the antimelanoma and metastatic activity of pentoxifylline in B16F10 melanoma. Int J Cancer, 121, 1600–1608.
- Prashar R, Kumar A, Banerjee S, Rao AR. (1994). Chemopreventive action by an extract from Ocimum sanctum on mouse skin papillomagenesis and its enhancement of skin glutathione-S-transferase activity and acid soluble sulfahydryl level. Anticancer Drugs, 5, 567–572.
- Radford IR. (1985). The level of induced DNA double-strand breakage correlates with cell killing after X-irradiation. Int J Radiat Biol, 48, 45–54.
- Rigel DS, Carucci JA. (2000). Malignant melanoma: prevention, early detection, and treatment in the 21st century. CA Cancer J Clin, 50, 215–236.
- Sabatino MA, Colombo T, Geroni C, Marchini S, Broggini M. (2003). Enhancement of in vivo antimelanoma activity of classical anticancer agents by combination with the new, glutathione-interacting DNA minor groove-binder, brostallicin. Clin Cancer Res, 9, 5402–5408.
- Satzger I, Meier A, Schenck F, Kapp A, Hauschild A, Gutzmer R. (2007). Autoimmunity as a prognostic factor in melanoma patients treated with adjuvant low-dose interferon alpha. Int J Cancer, 121, 2562–2566.
- Smalley KSM, Herlyn M. (2009). Integrating tumor-initiating cells into the paradigm for melanoma targeted therapy. Int J Cancer, 124, 1245–1250.
- Somashekar S, Saraswati U, Laxminarayana U, Nagabhushana S. (2006). Wound healing activity of Ocimum sanctum Linn with supportive role of antioxidant enzymes. Indian J Physiol and Pharmacol, 50, 163–168.
- Thompson JF, Scolyer RA, Kefford RF. (2005). Cutaneous melanoma. Lancet, 365, 687–701.
- Uma Devi P, Ganasoundari A, Vrinda B, Srinivasanb KK, Unnikrishnanb MK. (2000). Radiation protection by the Ocimum flavonoids orientin and vicenin: mechanisms of action. Radiat Res, 154, 455–460.
- Usseinimehr SJ, Shafiee A, Mozdarani H, Akhlagpour S. (2001). Radioprotective effects of 2-iminothiazolidine derivatives against lethal dose of gamma radiation in mice. J Radiat Res, 42, 401–408.
- Usseinimehr SJ, Shafiee A, Mozdarani H, Akhlagpour S, Froughizadeh M. (2002). Radioprotective effects of 2-imino-3[(chromone-2-yl) carbonyl] thiazolidine against gamma irradiation in mice. J Radiat Res, 43, 293–300.
- Ward JF. (1986). Mechanisms of DNA repair and their potential modification for radiotherapy. Int J Radiat Oncol Biol Phys, 12, 1027–1032.
- Ward JF. (1975). Molecular mechanisms of radiation induced damage to nucleic acids. Adv Radiat boil, 5, 182–239.
- Wattenberg LW. (1983). Inhibition of neoplasia by minor dietary constituents. Cancer Res, 43, 2448–2453.