Abstract
Context: Glutathione reductase (GR, NADPH:oxidized glutathione oxidoreductase, E.C 1.6.4.2) is a flavoprotein that catalyzes the NADPH-dependent reduction of oxidized glutathione (GSSG). GR is a crucial enzyme in the antioxidant system by maintaining reduced glutathione (GSH). Glucose 6-phosphate dehydrogenase (G6PD, glucose 6-phosphate (G6P):NADP+ oxidoreductase, EC 1.1.1.49) is the key regulatory enzyme of the pentose phosphate pathway and maintains NADPH for reductive reactions.
Objective: Rosmarinic acid (RA; α-O-caffeoyl-3,4-dihydroxyphenyl lactic acid) is an ester of caffeic acid (CA) and 3,4-dihydroxyphenyllactic acid. It has a number of interesting biological activities. The inhibiting activities of the RA on GR and G6PD are investigated here for the first time.
Materials and methods: GR and G6PD were purified from tissues, then the effects of RA are investigated.
Results: This study reports that RA, which was isolated from Echium vulgare L. (Boraginaceae), inhibits purified GR and G6PD in a concentration-dependent manner. Kinetic characterizations and inhibition constants are investigated.
Discussion and conclusion: Because of their importance in the antioxidative defense system, investigation of the inhibitors of these enzymes is important for drug development.
Introduction
Rosmarinic acid (RA) is an ester of caffeic acid (CA) and 3,4-dihydroxyphenyllactic acid. It has medicinal uses (e.g. antioxidant, anti-inflammatory, astringent, antimutagen, antibacterial, and antiviral) and is mainly found in species of the Boraginaceae and Lamiaceae. The genus Echium vulgare L. (Boraginaceae) contains pyrrolizidine alkaloids, flavonoids, phenol carboxylic acids, sterones, and naphthoquinones (CitationPardo et al., 2000; CitationMitkov et al., 2002). Notably, E. vulgare L. has a high concentration of RA. We have isolated four compounds; RA, 3-(3′,4′-dihydroxyphenyl)-(2R)-lactic acid, kaempferol 3-O-neohesperidoside, and uridine, from E. vulgare. These isolated four compounds have been identified by using 1H NMR, 13C NMR, COSY, HMQC, HMBC, and ESI MS (CitationKuruüzüm-Uz et al., 2004).
Glutathione reductase (GR) is an ubiquitous enzyme that catalyzes the NADPH-dependent reduction of oxidized glutathione (GSSG) to reduced glutathione (CitationRana et al., 2002). GR is a homodimeric flavoenzyme of known geometry. In consideration of the amino acids at the catalytic cite, cysteine, histidine, and tyrosine are critical amino acids for the reaction of GR (CitationUntucht-Grau et al., 1981; CitationDeonarain et al., 1989; CitationArscott et al., 2000). GR is known to be sensitive to chemical modification of the redox-active thiol groups. The redox properties of this enzyme can be inhibited or activated by several chemicals, drugs, or natural substrates (CitationBauer et al., 2006).
Glucose 6-phosphate dehydrogenase (G6PD) is the first and rate-limiting enzyme of the pentose phosphate pathway and the principal intracellular source of the reduced form of NADPH (CitationLeopold et al., 2007). Increase in G6PD gene expression has been associated with increasing GSH levels and resistance to oxidative stress (CitationWakao et al., 2008). Particular amino acids in substrate and coenzyme-binding site of G6PD have been investigated; cysteine, histidine, arginine, and lysine residues are involved in NADP+ binding and essential for catalysis of G6PD (CitationBautista et al., 1995; CitationWenderoth et al., 1997; CitationVought et al., 2000).
Reactive oxygen species (ROS) and various electrophiles are involved in the etiology of many diseases varying from cancer to cardiovascular and pulmonary disorders. The human body is protected against damaging effects of these compounds by antioxidative system. GR and G6PD are crucial enzymes in the antioxidative system and alterations in the activities of these enzymes may affect cellular defense system. The aim of the present study was to investigate the effects of RA on bovine kidney cortex, liver and yeast GR, and sheep brain cortex G6PD. We have found that RA inhibits these enzymes in a concentration-dependent manner.
Materials and methods
Materials
Sheep brain, bovine kidney and liver obtained from a local slaughterhouse was kept in ice and processed within 2–3 h of death.
Glucose 6-phosphate (G6P), nicotinamide adenine dinucleotide phosphate (NADP+), NADPH, GSSG, Tris [Tris(hydroxymethyl)aminomethane], DEAE Sepharose Fast Flow and Type VI Baker’s yeast GR were obtained from Sigma Chemical Co., St. Louis, MO. The 2′,5′-ADP-Sepharose 4B was obtained from Pharmacia Fine Chemicals, Uppsala, Sweden. bovine serum albumin (BSA) was obtained from British Drug Houses Ltd., England.
All other chemicals were of analytical grade and were obtained from Sigma Chemical Co.
Purification of G6PD
We have previously described a purification method for G6PD from bovine lens and sheep kidney cortex (CitationUlusu et al., 1999; CitationUlusu & Tandogan, 2006). We have used the same method for the purification of G6PD from sheep brain cortex. We have preferred to purify the enzyme by 2′,5′-ADP-Sepharose 4B affinity and DEAE Sepharose Fast Flow ion-exchange chromatography columns in two purification step orders. The purified enzyme was ~9400-fold and has a specific activity of 50 U/mg protein (CitationSengezer & Ulusu, 2007).
Purification of GR
Bovine kidney cortex and liver GR is purified by chromatography consisting of two steps after ultracentrifugation by Beckman L7-80 ultracentrifuge and heat denaturation: 2′,5′-ADP Sepharose 4B affinity and DEAE Sepharose Fast Flow anion exchange chromatography (CitationUlusu & Tandogan, 2007; CitationTandogan & Ulusu, 2010). Bovine liver GR was purified 28,028-fold with a final yield of 43%. Bovine kidney cortex GR was purified 34,806-fold with a final yield of 85%.
Assay of G6PD
Enzyme activities were determined spectrophotometrically using an Ultrospec 2100 pro(UV/visible) spectrophotometer, by monitoring the NADPH production at 340 nm and at 37°C (ϵ340 = 6.22 per mM/cm). The assay mixture contained 10 mM MgCl2, 0.2 mM NADP+, and 0.6 mM G6P in 100 mM Tris/HCl buffer, pH 8.0 (CitationBetke et al., 1967). Assays were carried out in triplicates and the activities were followed for 60 sec. The reaction was linear during this time period.
One unit (U) of activity is the amount of enzyme required to reduce 1 μmol of NADP+ per min under the assay conditions. Specific activity is defined as units per mg of protein.
Assay of GR
GR activity was determined according to a modified version of Stall’s method (CitationAcan & Tezcan, 1989). The incubation mixture contained 100 mM sodium phosphate buffer, pH 7.4; 1 mM GSSG; and 0.2 mM NADPH. Decrease in the absorbance of NADPH at 340 nm was monitored spectrophotometrically at 37°C. Assays were carried out in triplicates.
A unit of activity (U) was defined as the amount of enzyme that catalyzes the oxidation of 1 μmol of NADPH in 1 min under these conditions. Specific activity is defined as units per mg of protein.
Protein determination
Protein concentrations in column fractions were determined by measuring the absorbance at 280 nm and protein concentrations of the purification steps were determined as described by CitationBradford (1976) using BSA as standard.
Inhibition studies
Activities are measured by adding different concentrations of RA in the assay mixture given above for GR and G6PD measurement. Assays of GR and G6PD in the presence of RA performed in the system without enzyme-inhibitor pre-incubation in which the reactions are initiated by adding enzyme to a substrate–inhibitor mixture.
Plant material
In this study, RA was isolated from E. vulgare, which was collected from Tortum-Erzurum, Turkey (alt. 1730 m). A voucher specimen, identified by Dr. Yusuf Kaya, has been deposited in Department of Biology (ATA 9732), Atatürk University, Erzurum, Turkey.
Extraction and isolation
For isolation of RA, dried and powdered flowering aerial parts of E. vulgare (340 g) were used. Flowered parts of the plants were extracted with methanol (MeOH) (3 × 3.5 L, 45°C) and the combined extracts were evaporated to yield 29.9 g residue. The MeOH extract was dissolved in H2O and partitioned with CHCl3 (8.4 g). Aqueous phase (20 g) was fractionated on a silica gel column eluting with a gradient solvent system (CHCl3–MeOH) and from this chromatography five main fractions were obtained. The fraction V was further chromatographed over reverse-phase silica gel and Sephadex LH-20 columns using MeOH led to the isolation of compound 1 (73 mg) (CitationKuruüzüm-Uz et al., 2004). The molecular formula of compound 1 was established as C18H16O8 on the basis of NMR data and the peak of [M+H]+ at m/z = 360 in ESI MS.
Statistical analysis of kinetic data
The data are analyzed and the kinetic constants are calculated with following equations (CitationSegel, 1975) by means of a nonlinear curve-fitting program of the Statistica.
Results
As a result of the serial chromatographic separations, RA were isolated and its structure elucidated based on spectroscopic data (1H NMR, 13C NMR, COSY, HMQC, HMBC, and ESI MS). The NMR data of compound 1 were found similar to previously reported data (CitationLu & Foo, 1999) ().
In this study, we have investigated the in vitro effects of RA on GR from bovine kidney cortex, liver, and yeast. We have purified GR from bovine liver and kidney cortex and then analyzed the effect of RA on the purified enzyme (CitationUlusu & Tandogan, 2007; CitationTandogan & Ulusu, 2010). Baker’s yeast GR is obtained from Sigma and the specific activity of the enzyme is 1.25 U/mg. To investigate the in vitro effect of RA on the activity of G6PD, we primarily have purified G6PD from sheep brain cortex (CitationSengezer & Ulusu, 2007).
Inhibition of bovine kidney cortex GR by RA
In the inhibition of bovine kidney cortex GR by RA, we used RA at different concentrations (0–1 mM) as an inhibitor. It is found that RA inhibits the GR in a concentration-dependent manner and the obtained IC50 value of RA is 0.2 ± 0.003 mM.
Kinetics of RA inhibition of bovine kidney cortex GR
Kinetic studies on the inhibition of bovine kidney cortex GR by RA have been investigated by using Lineweaver–Burk double reciprocal plot and initial velocity data is analyzed.
When GSSG is varied substrate, at constant and unsaturating NADPH concentration (0.1 mM), different fixed concentrations of RA (0.1–0.4 mM) are added into the assay mixture and initial velocities are measured. It is seen that RA acts as a non-competitive inhibitor with respect to GSSG () and from “Statistica Program” Ki is calculated as (KiGSSG 0.158 ± 0.009 mM).
Figure 2. Inhibition kinetics of bovine kidney cortex glutathione reductase (GR). Lineweaver–Burk double reciprocal plot of initial velocity against oxidized glutathione (GSSG) as varied substrate and rosmarinic acid (RA) (0.1–0.4 mM) as inhibitor at different fixed NADPH (0.1 mM) concentrations.
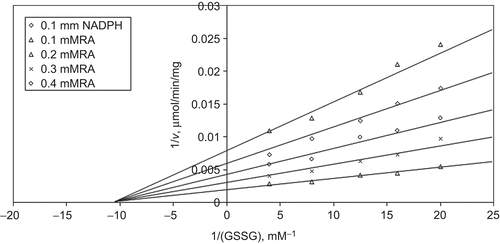
When NADPH is varied substrate, at constant and unsaturating GSSG concentration (0.7 mM), different fixed concentrations of RA (0.1–0.6 mM) are added into the assay mixture. It is shown that RA acts as a non-competitive inhibitor with respect to NADPH, and Ki is calculated as 0.443 ± 0.03 mM ().
Inhibition of bovine liver GR by RA
In the inhibition of bovine liver GR by RA, we used RA at different concentrations (0–1 mM) as an inhibitor. It is found that RA inhibits the GR in a concentration-dependent manner and the obtained IC50 value of RA is 0.104 ± 0.02 mM.
Kinetics of RA inhibition of bovine liver GR
Kinetic studies on the inhibition of bovine liver GR by RA have been investigated by using Lineweaver–Burk double reciprocal plot and initial velocity data is analyzed.
When GSSG is varied substrate, at constant and unsaturating NADPH concentration (0.1 mM), different fixed concentrations of RA (0.1–0.4 mM) are added into the assay mixture and initial velocities are measured. It is seen that RA acts as a non-competitive inhibitor with respect to GSSG () and from “Statistica Program” Ki is calculated as (KiGSSG 0.124 ± 0.009 mM).
Figure 4. Inhibition kinetics of bovine liver glutathione reductase (GR). Inhibition kinetics of Lineweaver–Burk double reciprocal plot of initial velocity against oxidized glutathione (GSSG) as varied substrate and rosmarinic acid (RA) (0.1–0.4 mM) as inhibitor at different fixed NADPH (0.1 mM) concentrations.
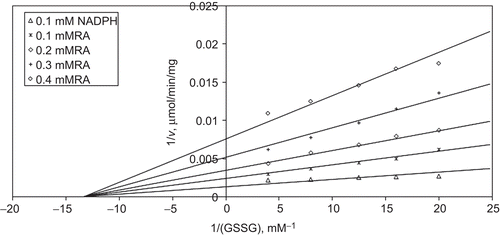
When NADPH is varied substrate, at constant and unsaturating GSSG concentration (0.7 mM), different fixed concentrations of RA (0.1–0.6 mM) are added into the assay mixture. It is shown that RA acts as a non-competitive inhibitor with respect to NADPH, and Ki is calculated as 0.556 ± 0.03 mM ().
Inhibition of yeast GR by RA
We have investigated the in vitro effects of RA on Baker’s yeast GR by using different (0–1 mM) concentrations as an inhibitor. The obtained IC50 value of RA is 0.112 ± 0.01 mM.
Kinetics of RA inhibition of yeast GR
Kinetic studies on the inhibition of Baker’s yeast GR by RA have been investigated by using Lineweaver–Burk double reciprocal plot and initial velocity data is analyzed.
When GSSG is varied substrate, at constant and unsaturating NADPH concentration (0.1 mM), different fixed concentrations of RA (0.1–0.4 mM) are added into the assay mixture and initial velocities are measured. It is seen that RA acts as an uncompetitive inhibitor with respect to GSSG () and from “Statistica Program” Ki is calculated as (KiGSSG 0.229 ± 0.01 mM).
Figure 6. Inhibition kinetics of yeast glutathione reductase (GR). Lineweaver–Burk double reciprocal plot of initial velocity against oxidized glutathione (GSSG) as varied substrate and rosmarinic acid (RA) (0.1–0.4 mM) as inhibitor at different fixed NADPH (0.1 mM) concentrations.
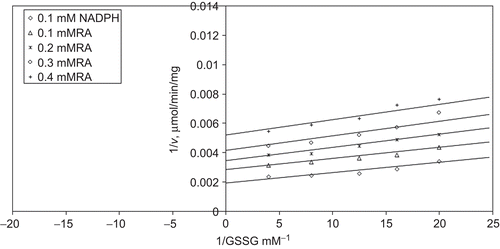
When NADPH is varied substrate, at constant and unsaturating GSSG concentration (0.7 mM), different fixed concentrations of RA (0.1–0.6 mM) are added into the assay mixture. It is shown that RA acts as an uncompetitive inhibitor with respect to NADPH, and Ki is calculated as KiNADPH 0.527 ± 0.04 mM ().
Inhibition of sheep brain cortex G6PD
In the inhibition of sheep brain cortex G6PD by RA, we used RA at different concentrations (0–3 mM) as an inhibitor. It is found that RA inhibits sheep brain cortex G6PD in a concentration-dependent manner and the obtained IC50 value of RA is 1.50 ± 0.0005 mM.
Kinetics of RA inhibition of sheep brain cortex G6PD
Kinetic studies on the inhibition of sheep brain cortex G6PD by RA have been investigated by using Lineweaver–Burk double reciprocal plot and initial velocity data is analyzed.
When G6P is varied substrate, at constant and unsaturating NADP+ concentration (0.1 mM), different fixed concentrations of RA (0–2 mM) are added into the assay mixture and initial velocities are measured. It is seen that RA acts as a competitive inhibitor with respect to G6P () and from “Statistica Program” Ki is calculated as (KiG6P 1.12 ± 0.002 mM).
Figure 8. Inhibition kinetics of sheep brain cortex glucose 6-phosphate dehydrogenase (G6PD). Lineweaver–Burk double reciprocal plot of initial velocity against glucose 6-phosphate (G6P) as varied substrate and rosmarinic acid (RA) (0–2 mM) as inhibitor at different fixed NADP+ (0.1 mM) concentrations.
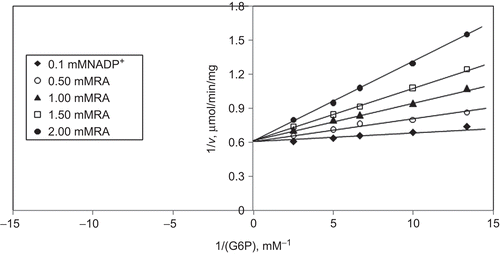
When NADP+ is varied substrate, at constant and unsaturating G6P concentration (0.4 mM), different fixed concentrations of RA (0–2 mM) are added into the assay mixture. It is shown that RA acts as a competitive inhibitor with respect to NADP+, and Ki is calculated as 0.67 ± 0.003 mM ().
Figure 9. Inhibition kinetics of sheep brain cortex glucose-6-phosphate dehydrogenase (G6PD). Lineweaver–Burk double reciprocal plot of initial velocity against NADP+ as varied substrate and rosmarinic acid (RA) (0–2 mM) as inhibitor at different fixed glucose 6-phosphate (G6P) (0.4 mM) concentrations.
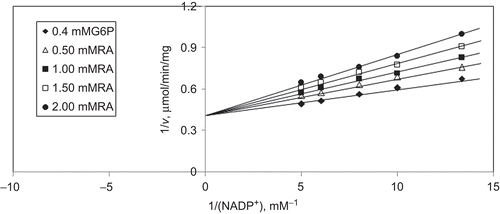
Discussion
RA has antioxidant, anti-inflammatory, astringent, antimutagen, antibacterial, and antiviral properties and it is rapidly eliminated from the blood circulation after intravenous application and shows a very low toxicity (LD50 in mice of 561 mg/kg after intravenous application) (CitationParnham & Kesselring, 1985). Phenolic compounds like RA can provide protection against cancer and it contributes to the antioxidant activity of plants used in the cosmetic industry (CitationD’Amelio, 1999). The studies on the activity of RA confirm that caffeoyl esters show the highest antioxidant activity. RA has been reported to exhibit antioxidant properties and is protective against peroxidative damage (CitationLiu et al., 1992). It prevents the oxidation of LDL, which is compatible with anti-inflammatory and anti-atherosclerotic role in pathophysiological conditions (CitationCartron et al., 2001). However, RA inhibits amylase activity, the extent of amylase inhibition correlated with increased concentration of RA (CitationMcCue & Shetty, 2004) Similarly, in a concentration-dependent manner, aldose reductase, phospholipase A(2) from different sources, and carboxypeptidase A are inhibited with RA (CitationKusano et al., 1998; Citationda Silva et al., 2009; CitationKoukoulitsa et al., 2010). Overdoses of protocatechuic acid, which is a phenolic antioxidant, can disturb the detoxification of other electrophilic toxicants (CitationNakamura et al., 2001).
In this study, we have found the in vitro effects of RA on GR and G6PD in a concentration-dependent manner. According to our results; RA inhibits both of these antioxidant enzymes in high concentration. Our results indicate that RA inhibition may be due to the interaction of RA with sensitive SH groups present in G6PD. G6PD activity is thought to depend on the intactness of SH groups; inhibitory effect of RA may be due to binding thiol groups in the enzyme. In consideration of the amino acid residue in the substrate and NADP+-binding site or catalytic site, cysteine residues were found, which play an essential role for the activation of G6PD (CitationWenderoth et al., 1997).
RA inhibits bovine kidney cortex and liver GR non-competitively with respect to both GSSG and NADPH. While a non-competitive inhibitor may bind to a non-substrate site on an enzyme and distort it to the point of non-functionality, an uncompetitive inhibitor binds only to the enzyme–substrate complex (CitationSegel, 1975). Yeast GR is inhibited uncompetitive with respect to both GSSG and NADPH. RA may bind to yeast GR substrate complex and leads to uncompetitive inhibition. Differences in amino acid composition in catalytic site of enzymes may be the cause of different inhibitory effects between bovine tissue and yeast GR. It has been shown that comparison with Escherichia coli and human GR sequences reveals 49.8% amino acid identity (CitationCollinson & Dawes, 1995). Despite that the overall structure and amino acid homology in GR enzymes, the sequence of amino aid residues around the active site in yeast GR shows diversity. For instance, GR from the cyanobacterium Anabaena PCC 7120 contains a pyridine nucleotide-binding motif differing from that of the enzyme from other sources and an insertion of 10 amino acid residues. Enzyme might show different coenzyme specificity than GR from other sources (CitationDanielson et al., 1999).
Notably, although RA has antioxidant properties, high concentrations of RA inhibit both G6PD and GR activity. GR inhibition may influence total intracellular GSH levels and lowers cellular defense against oxidative stress. Because of G6PD is an important enzyme in many reductive biosynthesis and GSH regeneration, its inhibition by RA may induce oxidative damage that causes hemolytic anemia in G6PD-deficient individuals.
Inhibition of G6PD and GR activities has many key physiological and biochemical importance for all of the organisms because of the crucial roles in pathways and antioxidant impacts. However, both of these enzymes are involved in antioxidant system and inhibition of these enzymes may be cascade effect in increasing oxidative stress. And, as a result, inhibition of these enzymes may be harmful to the organism because of the failure in antioxidant defense mechanism. Inhibition of G6PD and GR may be beneficial if these enzymes are overexpressed from causative of a disease. Pharmacodynamics, toxicology, and clinic studies should be done for explaining the fate and the pathophysiological effects of this natural product in organisms. Also, one point should not be forgotten that G6PD deficiency is the most common enzymopathy. Further investigations must be done for various diseases and disorders by new inhibitors or activators for new drug proposals. Finding or developing a new enzyme inhibitor will be helpful in future drug discovery for various diseases.
Declaration of interest
This work is a part of a project and (09D05101001 and 0701101011) supported by Hacettepe University Scientific Research Unit.
References
- Acan NL, Tezcan EF. (1989). Sheep brain glutathione reductase: purification and general properties. FEBS Lett, 250, 72–74.
- Arscott LD, Veine DM, Williams CH Jr. (2000). Mixed disulfide with glutathione as an intermediate in the reaction catalyzed by glutathione reductase from yeast and as a major form of the enzyme in the cell. Biochemistry, 39, 4711–4721.
- Bauer H, Fritz-Wolf K, Winzer A, Kühner S, Little S, Yardley V, Vezin H, Palfey B, Schirmer RH, Davioud-Charvet E. (2006). A fluoro analogue of the menadione derivative 6-[2′-(3′-methyl)-1′,4′-naphthoquinolyl]hexanoic acid is a suicide substrate of glutathione reductase. Crystal structure of the alkylated human enzyme. J Am Chem Soc, 128, 10784–10794.
- Bautista JM, Mason PJ, Luzzatto L. (1995). Human glucose-6-phosphate dehydrogenase. Lysine 205 is dispensable for substrate binding but essential for catalysis. FEBS Lett, 366, 61–64.
- Betke K, Brewer GJ, Kirkman HN, Luzzato L, Motulsky AG, Ramot B, Siniscalco M. (1967). Standardized method for G-6-PD assay of haemolysates. WHO Tech Rep Ser, 366, 30–32.
- Bradford MM. (1976). A rapid and sensitive method for the quantitation of microgram quantities of protein utilizing the principle of protein–dye binding. Anal Biochem, 72, 248–254.
- Cartron E, Carbonneau MA, Fouret G, Descomps B, Léger CL. (2001). Specific antioxidant activity of caffeoyl derivatives and other natural phenolic compounds: LDL protection against oxidation and decrease in the proinflammatory lysophosphatidylcholine production. J Nat Prod, 64, 480–486.
- Collinson LP, Dawes IW. (1995). Isolation, characterization and overexpression of the yeast gene, GLR1, encoding glutathione reductase. Gene, 156, 123–127.
- D’Amelio FS. (1999). Botanicals, A Phytocosmetic Desk Reference. CRC Press, London, p. 361.
- da Silva SL, Calgarotto AK, Maso V, Damico DC, Baldasso P, Veber CL, Villar JA, Oliveira AR, Comar M Jr, Oliveira KM, Marangoni S. (2009). Molecular modeling and inhibition of phospholipase A2 by polyhydroxy phenolic compounds. Eur J Med Chem, 44, 312–321.
- Danielson UH, Jiang F, Hansson LO, Mannervik B. (1999). Probing the kinetic mechanism and coenzyme specificity of glutathione reductase from the cyanobacterium Anabaena PCC 7120 by redesign of the pyridine-nucleotide-binding site. Biochemistry, 38, 9254–9263.
- Deonarain MP, Berry A, Scrutton NS, Perham RN. (1989). Alternative proton donors/acceptors in the catalytic mechanism of the glutathione reductase of Escherichia coli: the role of histidine-439 and tyrosine-99. Biochemistry, 28, 9602–9607.
- Koukoulitsa C, Bailly F, Pegklidou K, Demopoulos VJ, Cotelle P. (2010). Evaluation of aldose reductase inhibition and docking studies of 6′-nitro and 6′,6′′-dinitrorosmarinic acids. Eur J Med Chem, 45, 1663–1666.
- Kuruüzüm-Uz A, Güvenalp Z, Ströch K, Demirezer LÖ, Zeeck A. (2004). Phytochemical and antimicrobial investigation of Echium vulgare growing in Turkey. Biochem Syst Ecol, 32, 833–836.
- Kusano G, Takahira M, Shibano M, Kusano A, Okamoto Y, Tsujibo H, Numata A, Inamori Y. (1998). Studies on inhibitory activities of fukiic acid esters on germination, alpha-amylase and carboxypeptidase A. Biol Pharm Bull, 21, 997–999.
- Leopold JA, Dam A, Maron BA, Scribner AW, Liao R, Handy DE, Stanton RC, Pitt B, Loscalzo J. (2007). Aldosterone impairs vascular reactivity by decreasing glucose-6-phosphate dehydrogenase activity. Nat Med, 13, 189–197.
- Liu GT, Zhang TM, Wang BE, Wang YW. (1992). Protective action of seven natural phenolic compounds against peroxidative damage to biomembranes. Biochem Pharmacol, 43, 147–152.
- Lu Y, Foo LY. (1999). Rosmarinic acid derivatives from Salvia officinalis. Phytochemistry, 51, 91–94.
- McCue PP, Shetty K. (2004). Inhibitory effects of rosmarinic acid extracts on porcine pancreatic amylase in vitro. Asia Pac J Clin Nutr, 13, 101–106.
- Mitkov S, Obreshkova D, Ilieva I, Pangarova T, Pencheva I. (2002). Phenolcarboxylic acids in Echium vulgare L. Acta Pharm Turcica, 44, 43–48.
- Nakamura Y, Torikai K, Ohigashi H. (2001). Toxic dose of a simple phenolic antioxidant, protocatechuic acid, attenuates the glutathione level in ICR mouse liver and kidney. J Agric Food Chem, 49, 5674–5678.
- Pardo F, Perich F, Torres R, Delle Monache F. (2000). Stigmast-4-ene-3,6-dione an unusual phytotoxic sterone from the roots of Echium vulgare L. Biochem Syst Ecol, 28, 911–913.
- Parnham MJ, Kesselring K. (1985). Rosmarinic acid. Drugs Future, 10, 756–757.
- Rana SV, Allen T, Singh R. (2002). Inevitable glutathione, then and now. Indian J Exp Biol, 40, 706–716.
- Segel IH. (1975). Enzyme Kinetics: Behavior and Analysis of Rapid Equilibrium and Steady-State Enzyme Systems. John Wiley & Sons, New York.
- Sengezer C, Ulusu NN. (2007). Three different purification protocols in purification of G6PD from sheep brain cortex. FABAD J. Pharm. Sci, 32, 65–72.
- Tandogan B, Ulusu NN. (2010). Purification and kinetics of bovine kidney cortex glutathione reductase. Protein Pept Lett, 17, 667–674.
- Ulusu NN, Kus MS, Acan NL, Tezcan EF. (1999). A rapid method for the purification of glucose-6-phosphate dehydrogenase from bovine lens. Int J Biochem Cell Biol, 31, 787–796.
- Ulusu NN, Tandogan B. (2007). Purification and kinetic properties of glutathione reductase from bovine liver. Mol Cell Biochem, 303, 45–51.
- Ulusu NN, Tandogan B. (2006). Purification and kinetics of sheep kidney cortex glucose-6-phosphate dehydrogenase. Comp Biochem Physiol B, Biochem Mol Biol, 143, 249–255.
- Untucht-Grau R, Schirmer RH, Schirmer I, Krauth-Siegel RL. (1981). Glutathione reductase from human erythrocytes: amino-sequence of the structurally known FAD-binding domain. Eur J Biochem, 120, 407–419.
- Vought V, Ciccone T, Davino MH, Fairbairn L, Lin Y, Cosgrove MS, Adams MJ, Levy HR. (2000). Delineation of the roles of amino acids involved in the catalytic functions of Leuconostoc mesenteroides glucose 6-phosphate dehydrogenase. Biochemistry, 39, 15012–15021.
- Wakao S, Andre C, Benning C. (2008). Functional analyses of cytosolic glucose-6-phosphate dehydrogenases and their contribution to seed oil accumulation in Arabidopsis. Plant Physiol, 146, 277–288.
- Wenderoth I, Scheibe R, von Schaewen A. (1997). Identification of the cysteine residues involved in redox modification of plant plastidic glucose-6-phosphate dehydrogenase. J Biol Chem, 272, 26985–26990.