Abstract
Context: Pain-relieving plaster (PRP) is a traditional Chinese medicine (TCM) that has been widely used with satisfactory results in the treatment of some diseases related to inflammation, such as bruises, chronic arthritis.
Objective: The mechanisms underlying the anti-inflammatory actions of PRP are investigated in this study for the first time.
Materials and methods: The anti-inflammatory effects of PRP extracts were evaluated in lipopolysaccharide (LPS) or calcium ionophore A23187-treated murine peritoneal macrophages (PMs). Tumor necrosis factor-α (TNF-α), interleukin-1β (IL-1β), prostaglandin E2 (PGE2), and leukotrienes B4 (LTB4) were evaluated by ELISA assays. Reverse transcriptase-polymerase chain reaction (RT-PCR) and western blot analysis were used to detect the expression of cyclooxygenase-2 (COX-2) and 5-lipoxygenase (5-LOX). Nuclear factor-kappa B (NF-κB)–DNA-binding activity was determined by gel mobility shift assay.
Results: PRP extracts were found to inhibit the production of TNF-α, IL-1β, and PGE2, reduce the expressions of COX-2 at the mRNA and protein levels induced by LPS, and reduced the production of LTB4 induced by A23187. Furthermore, PRP extracts significantly attenuated LPS-induced NF-κB–DNA-binding activity.
Discussion and conclusion: The anti-inflammatory effects of PRP possibly are related to reduction of inflammatory cytokines (TNF-α and IL-1β), inducible inflammatory enzyme (COX-2), and its metabolite PGE2 via NF-κB signal pathway. Moreover, PRP extracts also notably inhibited the production of LTB4, indicating that PRP inhibited the 5-LOX pathway, which may be the other mechanism for its anti-inflammatory action.
Introduction
Inflammation is a complex process regulated by various small molecular mediators, cytokines, and growth factors. Macrophages play a crucial role in the initiation, maintenance, and resolution of inflammation and immune responses and are major sources of inflammatory mediators such as tumor necrosis factor-α (TNF-α), interleukin-1β (IL-1β), prostaglandin E2 (PGE2), and so on (CitationShen et al., 2002; CitationHuang et al., 2008).
Among various proinflammatory chemical mediators, prostaglandins (PGs) and leukotrienes (LTs) synthesized from arachidonic acid (AA) play an important role in many inflammatory disorders. Cyclooxygenase (COX), the primary enzyme responsible for the synthesis of PGs, exist in at least three isoforms. COX-1 was recognized as a constitutive expression enzyme, which associated with physiological function, such as protection of gastric mucosa. COX-2 has been suggested to be an inducible isoform closely related to the inflammation (CitationWilloughby et al., 2000). On the other hand, since AA metabolizes through both the COX and 5-lipoxygenase (5-LOX) pathways, long-term inhibition of COX-2 could lead to a shunt to the 5-LOX pathway to generate LTs, as observed in vitro with OA osteoblasts (CitationMaxis et al., 2006), which can induce gastric lesions and bronchoconstriction. Thus, during recent years, combined 5-LOX/COX inhibition, interfering with the biosynthesis of both PGs and LTs, may be better than either COX inhibition or 5-LOX inhibition (CitationLeone et al., 2007; CitationYu et al., 2010).
It is well-known that nuclear factor-kappa B (NF-κB), a major transcription factor in the development of atherosclerotic injury and other inflammatory diseases, plays a pivotal role in the regulation of expression of proinflammatory cytokines, chemokines, adhesion molecules, and inducible enzymes such as inducible nitric oxide synthase (iNOS) and COX-2. Blocking the NF-κB transcriptional activity in the macrophages is conducive to suppress the expression of iNOS, COX-2, 5-LOX, and other inflammatory cytokines (CitationKim et al., 2007; CitationLee et al., 2010).
Recently, the rising interest in traditional Chinese medicines (TCMs) and their potential therapeutic effects on various inflammatory diseases has led to increased attention to their potential safety and efficacy. Among these widely used TCMs, pain-relieving plaster (PRP) is commonly used to treat bruises, sprains, and strains on muscles or joints, and relieve chronic pain such as rheumatic pains, stiff neck and backaches, arthritis (CitationZhen et al., 2006). It is composed of herbs from China Tibetan Plateau, such as Lamiophlomis rotata Kudo (Labiatae), Curcuma longa Linn. (Zingiberaceae), Oxytropis falcata Bung (Leguminosae), and Myricaria bracteata Royle (Tamaricaceae), which have been used clinically for hundreds of years in China to remove stasis and relieve pain (CitationLi et al., 2004; CitationNotoya et al., 2006). The quality control of PRP mainly focuses on the analysis of the active constituent, luteolin, contained in L. rotata, by high-performance liquid chromatography (HPLC). Recent clinical research has shown that PRP is helpful for soft tissue injury, omarthritis, and osteoarthritis by virtue of relieving pain and inhibiting inflammatory edema of diseased region (CitationDu, 2007; CitationYan & Ai, 2008; CitationZhao, 2010). Pharmacodynamic studies have shown that PRP has good anti-inflammatory effect on acute arthritis induced by carrageenan in mice and adjuvant chronic arthritis in rats, and it can reduce serum IL-1β level of acute soft tissue injury rabbits (CitationXu et al., 2004; CitationHe et al., 2008). However, the anti-inflammatory mechanism of PRP is rarely reported. The present study was designed to explore the potential anti-inflammatory mechanism of the extracts of PRP by investigating the influence on inflammatory cytokines production in macrophages stimulated by lipopolysaccharide (LPS) or A23187.
Materials and methods
Chemicals
LPS (Escherichia coli 055:B5), calcium ionophore A23187, Brewer thioglycollate medium, and RNase inhibitor were from Sigma-Aldrich (St. Louis, MO). RPMI-1640 was from GIBCO-BRL (Gaithersburg, MD). Trizol reagent was from Invitrogen (Carlsbad, CA). M-MLV reverse transcriptase, GoTaq® Green Master Mix, random hexamers, dNTPs mixture were from Promega (Madison, WI). Newborn calf serum was from PAA (Pasching, Austria). Mouse TNF-α ELISA kit and IL-1β ELISA kit were from eBioscience (San Diego, CA). PGE2 EIA kit, LTB4 EIA kit, and 5-LOX polyclonal antibody were from Cayman Chemical Company (Ann Arbor, MI). COX-1, COX-2, and glyceraldehyde 3-phosphate dehyrogenase (GAPDH) polyclonal antibody were from Santa Cruz (Santa Crux, CA). LightShift Chemiluminescent electrophoretic mobility shift assay (EMSA) kit and NE-PER® Nuclear and Cytoplasmic Extraction Reagents were from Pierce (Appleton, WI). Nondenaturing Lysis Buffer was from Applygen Technologies Inc. (Beijing, China). Methanol, HPLC grade, was purchased from Merck Co. Ltd. (Whitehouse Station, NJ). The other chemicals were of analytical purity from Beijing Chemical Reagents Company (Beijing, China).
Drugs
Diclofenac sodium (DS) was obtained from Jinan Dongfeng Pharmaceutical Factory Ltd. (purity>98%) (Shangdong, China), and was dissolved in dimethyl sulfoxide (DMSO) with initial concentration of 0.1 M and diluted with serum-free RPMI-1640 to appropriate concentrations. Ethanol extracts of PRP were offered by the Tibet Linzhi Cheezheng Tibetan Medicine Factory (Lanzhou, China) as a dark brown semisolid mass. The yield of the extracts was ~25% of plant powder. The lot number was No. 070428. PRP extracts were dissolved in DMSO with initial concentration equal to 1.25 g/mL crude drug and diluted with serum-free RPMI-1640 to appropriate concentrations for pharmacological experiments. For HPLC identification, ethanol extracts of PRP were offered as ethanol solution with the same lot number, and after the freeze-drying process, the residue of 10 mL PRP ethanol solution was dissolved in 25 mL methanol solution containing 2.5 mol/L hydrochloric acid and the weight was recorded. Then after 30 min of recirculation, the weight loss was supplemented by methanol. The solution was then filtered through a 0.45-µm membrane filter for HPLC analysis. PRP ethanol solution without L. rotata in the prescription was also prepared as negative control using the same method as above. Luteolin were purchased from National Institute for the Control of Pharmaceutical and Biological Products (Beijing, China). After drying by phosphoric anhydride overnight, luteolin was dissolved in methanol with final concentration 20 µg/mL as positive control.
Animals
Specific pathogen-free inbred male C57BL/6 mice were purchased from Experimental Animal Center, Institute of Laboratory Animal Science, Chinese Academy of Medical Sciences (CAMS) and Peking Union Medical College (PUMC) (Beijing, China). Mice were obtained at 8–12 weeks of age. Mice were housed in the experimental Animal center of Institute of Materia Medica Chinese Academy of Medical Sciences and Peking Union Medical College, which is fully accredited by Beijing Administration Office of Laboratory Animal, where they were fed standard animal chow and tap water ad libitum. The study complied with the Declaration of Helsinki, and followed a protocol approved by the Animal Care Committee of the local Institutional Review Board.
HPLC apparatus and operating conditions
HPLC analysis was performed on the Agilent 1100 system, equipped with a variable-wavelength UV detector (VWD), a thermostated column compartment, G1314A isocratic pump, and Agilent Chemstation software. Chromatographic separation was achieved on the Agilent ZORBAX XDB-C18 (4.6 × 250 mm, 5 µm). The samples were separated using a mobile phase of acetonitrile–tetrahydrofuran–0.5% phosphoric acid (20:6.4:73.6, v/v/v) at a flow rate of 1.0 mL/min, column temperature of 40°C. The injection volume of samples was 10 µL and detection was carried out at wavelength of 350 nm.
Isolation and culture of macrophages
Peritoneal macrophages (PMs) were harvested from the peritoneal cells of mice 4 days after the intraperitoneal injection of brewer thioglycollate medium (50 mL/kg body weight) as described previously (CitationShen et al., 2002). The cells were suspended in RPMI-1640 supplemented with 100 international units/mL penicillin, 100 μg/mL streptomycin (Roche Molecular Biochemicals, Indianapolis, IN), and 5% heat-inactivated fetal bovine serum. They were seeded in 48-well plates (1 × 106 cells/well) or 60-mm tissue culture dishes (4 × 106 cells/well) in 5% CO2 at 37°C. After 3 h incubation, nonadherent cells were removed by gently washing three times with phosphate-buffered saline (PBS). Cells were incubated with or without different concentration of drugs for 30 min in 5% CO2 at 37°C. Here, DS culture group was used as positive control group, and its final concentration was always 10 μM. Then 1 μg/mL (final concentration) LPS was added except for blank control group, and incubated in 5% CO2 at 37°C for another 18 h.
TNF-α, IL-1β, and PGE2 determination
After incubating for 18 h as above, the media were collected to measure TNF-α, IL-1β, and PGE2 concentrations by ELISA assay according to manufacturer’s instructions. In addition, cell viability was assessed by the MTT assay to detect whether the vehicle or drugs have cytotoxic activities.
Measurement of LTB4 production
LTB4 production was measured in cell-free supernatants using a commercially available LTB4 EIA kit as recommended by the manufacturer. In brief, after the pre-incubation of macrophages with drugs or vehicle at 37°C for 10 min, A23187 (final concentration 1 μM) and AA (final concentration 5 μM) were immediately added. The reaction system was incubated with shaking at 37°C for another 10 min, then the reaction was terminated in an ice bath. The conditioned media were harvested and LTB4 was quantified by EIA following the instructions supplied with the kit.
Reverse transcriptase-polymerase chain reaction
After incubating for 18 h as above (4 × 106 cells), the macrophages were washed twice with PBS, then the cells were lysed, and total RNA was extracted using Trizol reagent as previously described (CitationZaitsu et al., 2000). First strain cDNA was synthesized from 2 μg of total RNA with M-MLV reverse transcriptase and random hexamer, and then amplified by polymerase chain reaction (PCR) using GoTaq® Green Master Mix. PCR amplification of COX-1, COX-2, 5-LOX, FLAP, and LTA4 hydrolase (LTA4H) was performed with specific oligonucleotides (). The amplification cycles were 95°C for 45 sec, Tm, for 45 sec, and 72°C for 1 min, and repeated for 30 cycles. PCR products were analyzed by electrophoresis in 1.5% (w/v) agarose gel and visualized by ethidium bromide staining.
Table 1. List of primers for RT-PCR.
Western blot analysis
Polyclonal antibodies to COX-1, COX-2, GAPDH, and 5-LOX were used for western blotting. After washing with PBS twice, the macrophages were separated into nuclear and cytosol fractions using NE-PER® nuclear and cytoplasmic extraction reagents or Nondenaturing Lysis Buffer with a protease inhibitor cocktail. Protein concentrations were determined using a Bradford Protein Assay kit and bovine serum albumin (BSA) was employed as the standard. Then 30 μg proteins were separated on 5%–10% polyacrylamide gels and electrophoretically transferred onto polyvinylidene difluoride membranes. The membranes were blocked in Tris-buffered saline Tween-20 (TBST; 10 mM Tris–HCl, pH 8.0, 150 mM NaCl, 0.1% Tween-20) containing 5% fat-free milk powder overnight at 4°C, and then incubated with primary antibodies for 2 h. After washing three times with shaking, for 10 min each, the bound antibodies were detected by incubation with horseradish peroxidase (HRP)-conjugated secondary goat anti-rabbit or rabbit–goat IgG antibodies. After washing as above, the enzyme protein were visualized by using an enhanced chemiluminescence system, Supersignal Substrate Western Blotting, and photographed by luminescent image analyzer, LAS 1000 (Fuji Film, Tokyo, Japan).
Electrophoretic mobility shift assay
An oligonucleotide probe containing the NF-κB-binding motif (5′-AGT TGA GGG GAC TTT CCC AGG C-3′) was prepared and 5′-end labeled with biotin by Integrated DNA Technologies, Inc. (Coralville, IA). Detection of NF-κB–oligonucleotide complex was performed using a LightShift chemiluminescent EMSA kit (Pierce, Rockford, IL). In brief, nuclear protein (2 μg) was incubated with 28 fmol of biotin-labeled oligonucleotide for 20 min at room temperature in binding buffer consisting of 10 mM Tris at pH 7.5, 50 mM KCl, 1 mM dithiothreitol (DTT), 4% glycerol, 0.5 mM ethylenediaminetetraacetic acid (EDTA), 1 mM MgCl2, 50 ng Poly(dI·dC), and 0.05% NP-40. The specificity of the binding between NF-κB protein and DNA was determined in competition reactions in which a 200-fold molar excess (56 pmol) of unlabeled oligonucleotide was added to the binding reaction. Products of binding reactions were resolved by electrophoresis on a 6% polyacrylamide gel in 0.5 × TBE buffer (Invitrogen). NF-κB–oligonucleotide complex was electrophoretic transferred to BiodyneB nylon membrane (Pall, East Hills, NY) at 380 mA for 45 min. Then cross-link transferred DNA to membrane with a handheld UV lamp equipped with 254 nm bulbs. After incubation in blocking buffer for 15 min at room temperature, the membrane was incubated with streptavidin–HRP conjugate for 30 min at room temperature. The membrane was incubated with chemiluminescent substrate for 5 min, and allowed to expose to an appropriately equipped CCD camera.
Statistical analysis
Data were expressed as mean ± SD. Statistical analysis was performed using SPSS11.5. One-way ANOVA (for multiple comparisons) with post hoc analysis by the Dunnett’s test compares treatment groups to a specific control group. Significant differences were defined as P < 0.05.
Results
Identification and quantification of PRP extracts
Identification of the tested PRP extracts was carried out by comparing the retention time and on-line UV spectra with those of available standards. A VWD recording at 350 nm was used to detect luteolin. As shown in , the retention time of luteolin was about 19.1 min. The chromatographic profile of the negative control showed that there is no interference in the determination of luteolin, which is main component of L. rotata. Quantification was performed according to an external standard method. The quantitative data were obtained by plotting the concentrations of standard versus peak areas, and the concentration of luteolin in the PRP extracts was about 40 μg/mL.
Figure 1. Typical high-performance liquid chromatograms (HPLC). The content determination of luteolin in pain-relieving plaster (PRP) extracts was performed by HPLC. (A) Positive control with 20 μg/mL luteolin, (B) PRP extracts, (C) negative control of PRP extracts without Lamiophlomis rotata in the prescription. The flow rate of mobile phase was 1.0 mL/min and detection was performed at wavelength of 350 nm.
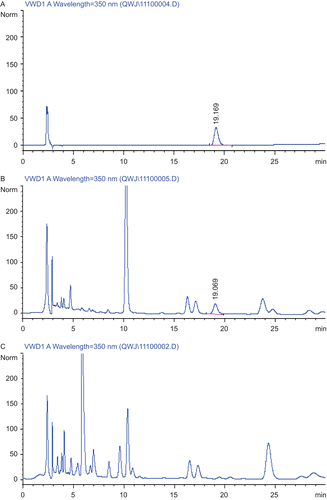
Effects of PRP extracts on the production of proinflammatory cytokines in LPS-stimulated PMs
To investigate the anti-inflammatory effects of PRP extracts on LPS-stimulated PMs, the production of proinflammatory cytokines was evaluated by ELISA. TNF-α () and IL-1β () levels were notably increased in the culture media of LPS-stimulated PMs, and these increases were significantly inhibited in a concentration-dependent manner by treatment with PRP extracts. The vehicle, 10−4 (v/v) DMSO, diluted in RPMI-1640 medium, did not affect the TNF-α, and IL-1β levels increased by LPS.
Figure 2. Pain-relieving plaster (PRP) extracts inhibited the production of lipopolysaccharide (LPS)-induced proinflammatory cytokines in peritoneal macrophages (PMs). PMs pretreated with PRP extracts (125, 62, 31 μg/mL) for 30 min, and then stimulated with LPS (1 μg/mL). Culture media were collected at 18 h in order to measure TNF-α (A) and IL-1β (B) concentrations using ELISA. Data are presented as means ± SD (n = 6). *P < 0.05, **P < 0.01, ***P < 0.001 vs. the LPS-treated group.
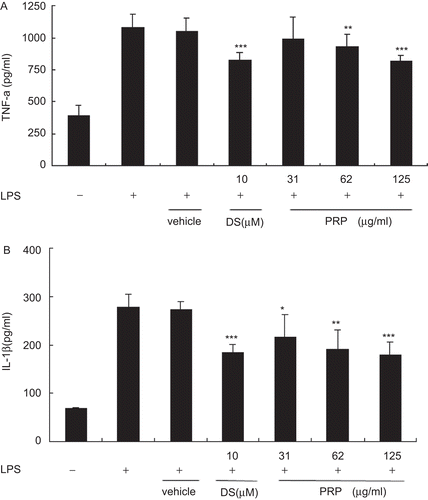
PRP extracts inhibit the production of PGE2 by suppressing COX-2 expression in LPS-stimulated PMs
After being incubated with LPS (1 μg/mL) for 18 h, the PMs produce large amounts of PGE2 (). As shown in the experiments, the contents of PGE2 increased from control levels of 96.00 ± 15.31 to 234.96 ± 24.02 pg/mL. DS, a well-known nonsteroidal anti-inflammatory drugs (NSAIDs), used as positive control, caused significant reduction of PGE2 (P < 0.01) at concentration of 10 µM. And all concentrations of PRP (from 31 to 125 µg/mL) concentration-dependently inhibited the formation of PGE2 with IC50 values ≈58.71 µg/mL. No significant cytotoxicity was observed in any groups tested in these experiments as measured by MTT assay (data not shown). To investigate whether PRP extracts could affect LPS-induced COX-2 expression, reverse transcriptase-PCR (RT-PCR) and western immunoblot analysis were carried out. Pronounced amounts of COX-2 mRNA and protein expression were induced upon exposure to LPS ( and ). PRP extracts significantly inhibited LPS-induced expression levels of COX-2 mRNA and protein in a dose-dependent manner. The vehicle, 10−4 (v/v) DMSO, diluted in RPMI-1640 medium, did not affect the expression levels of COX-2 mRNA and protein increased by LPS.
Figure 3. Pain-relieving plaster (PRP) extracts inhibited lipopolysaccharide (LPS)-induced prostaglandin E2 (PGE2) production and cyclooxygenase-2 (COX-2) expression in peritoneal macrophages (PMs). (A) PMs were pretreated with PRP extracts (31, 62, and 125 µg/mL) for 30 min, and then stimulated with LPS (1 µg/mL). Culture media were collected at 18 h in order to measure PGE2 concentrations using ELISA. Data are presented as means ± SD (n = 6). **P < 0.01, ***P < 0.001 vs. the LPS-treated group. (B) PMs were pretreated with vehicle, diclofenac sodium (DS), or 31.25, 62.5, and 125 μg/mL of PRP at 37°C for 30 min, then 1 μg/mL LPS (final concentration) was added and the incubation continued for another 18 h. Total RNA was extracted and RT-PCR was performed using specific primers as described in , and then isolated by electrophoresis on 1.5% agarose gels, the typical result of which was shown on the upside of the figure. The figures showed a representative result of three independent experiments. GAPDH expression was used as an internal control for RT-PCR. (C) PMs were pretreated with vehicle, DS, or 31.25, 62.5, and 125 μg/mL of PRP at 37°C for 30 min, then 1 μg/mL LPS (final concentration) was added and the incubation continued for another 18 h. Total protein was extracted, and then equal amounts (30 μg of protein) of protein were subjected to immunoblot analysis for cyclooxygenase-2 (COX-2), as described in “Materials and methods”. Shown are representative graphs of three independent experiments.
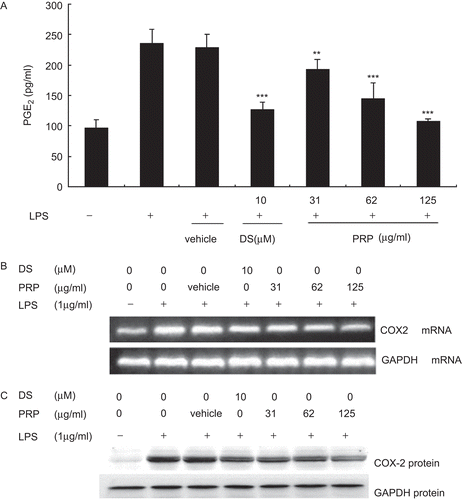
Effects of PRP extracts on the production of LTB4 and the enzymes related with 5-LOX pathway in PMs stimulated by ionophore A23187
Upon activation with A23187, PMs produced great amounts of LTB4. As shown in our experiments (), the concentrations of LTB4 increased from control levels of 12.13 ± 2.02 to 324.40 ± 100.05 pg/mL. Although DS caused significant reduction of PGE2 (P < 0.01), it had weak effect on LTB4 production at concentration of 10 µM. However, PRP extracts could significantly inhibited the production of LTB4 at the concentration of 62 and 125 µg/mL, with IC50 values ≈58.71 µg/mL. LTB4 is a dihydroxy fatty acid derived from AA through the 5-LOX pathway. Is it possible that PRP extracts have effect on the expression of LTB4 metabolizing enzymes, such as 5-LOX, LTA4H, and a specific helper 5-LOX-activating protein (FLAP), which is involved in converting AA to LTB4. As shown in , after incubating for 10 min, A23187 had no effect on the expression of 5-LOX, FLAP, and LTA4H mRNA in PMs, and PRP extracts also showed no influence on them. Moreover, the western blotting assay showed that after stimulation by A23187, all of the 5-LOX protein expressions were unchanged in comparison with resting PMs (). However, the content of 5-LOX protein in nuclear fractions showed a prominent augmentation, and in the meanwhile, 5-LOX protein in cytosol fractions were reduced. In other words, although A23187 had no effect on 5-LOX protein expression level, it caused a notable change on distribution of 5-LOX in cytosol and nuclear fractions. But PRP extracts showed negligible effect on the distribution of 5-LOX protein (). The vehicle, 10−4 (v/v) DMSO, diluted in RPMI-1640 medium, neither inhibited LTB4 formation nor affected the expression levels of 5-LOX, FLAP, and LTA4H and the distribution of 5-LOX.
Figure 4. The effects of pain-relieving plaster (PRP) extracts on the production of LTB4 and the enzymes related with 5-lipoxygenase pathway in peritoneal macrophages (PMs) stimulated by ionophore A23187. (A) PMs were treated with vehicle, diclofenac sodium (DS), or 31.25, 62.5 and 125 μg/mL of PRP at 37°C for 10 min, 1 μM A23187, and 5 μM AA for another 10 min. Culture media were collected to measure the concentration of LTB4 using ELISA. Data are presented as means ± SD (n = 5). *P < 0.05, **P < 0.01 vs. the lipopolysaccharide (LPS)-treated group. (B) PMs were treated with vehicle, DS, or 31.25, 62.5, and 125 μg/mL of PRP at 37°C for 10 min, 1 μM A23187, and 5 μM AA for another 10 min. Total RNA was extracted and RT-PCR was performed using specific primers as described in , and then isolated by electrophoresis on 1.5% agarose gels. The typical result of which was shown in the middle of the figure. GAPDH expression was used as an internal control for RT-PCR. (C) PMs were treated with vehicle, DS, or 31.25, 62.5, and 125 μg/mL of PRP at 37°C for 10 min, 1 μM A23187, and 5 μM AA for another 10 min. Total protein was extracted, and then equal amounts (30 μg of protein) of protein were subjected to immunoblot analysis for the enzymes related with 5-lipoxygenase (5-LOX) pathway, as described in “Materials and methods”. The typical results of which were shown in the figure.
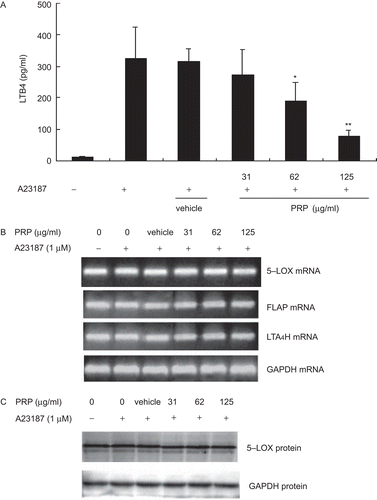
Figure 5. Effect of pain-relieving plaster (PRP) extracts on the subcellular distribution of immunoreactive 5-lipoxygenase (5-LOX) induced by A23187 in peritoneal macrophages (PMs). PMs were treated with vehicle, diclofenac sodium (DS), or 31.25, 62.5, and 125 μg/mL of PRP at 37°C for 10 min, 1 μM A23187, and 5 μM AA were added, the incubation continued for another 10 min. Total protein, cytosolic protein, or nuclear protein were extracted, respectively, and equal amounts (30 μg of protein) of each protein extracts were subjected to immunoblot analysis for 5-LOX, as described in “Materials and methods”. The typical results were from three independent experiments.
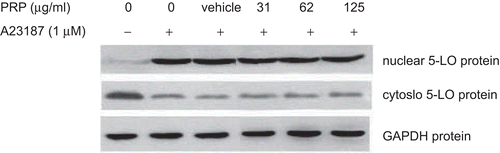
The effect of PRP extracts on LPS-induced NF-κB–DNA-binding activity
The effect of PRP extracts on LPS-induced NF-κB–DNA-binding activity was measured by EMSA assay. As shown in , in comparison with unstimulated PMs, the NF-κB–DNA-binding activity of LPS-stimulated PMs markedly increased. The test system of the NF-κB nuclear protein DNA binding was verified by control assay (lanes 1, 2, and 3). When 200-fold excess of unlabeled consensus NF-κB oligonucleotide (lane 3) were added with or without nuclear extracts (lane 1) in the binding reaction system, no binding bands appeared. Moreover, when both the probe and nuclear extracts were added (lane 2), the NF-κB-DNA binding band emerged. Pretreatment of cells with DS and various concentrations of PRP extracts suppressed the activity of NF-κB binding to its consensus DNA sequences. The vehicle, 10−4 (v/v) DMSO, diluted in RPMI-1640 medium, had no effect on the activity of NF-κB binding to its consensus DNA sequences.
Figure 6. Effect of pain-relieving plaster (PRP) extracts on the NF-κB–DNA-binding activity in peritoneal macrophages (PMs) induced by lipopolysaccharide (LPS). After incubation for 45 min, nuclear extracts were prepared from PMs and an electrophoretic mobility shift assay (EMSA) was performed, using a consensus biotin-labeled NF-κB-binding oligonucleotide. Results are representative of two independent experiments performed in duplicate. Lane 1: Probe alone, lane 2: probe with nuclear extracts from LPS-stimulated PMs (positive control), lane 3: 200-fold excess of unlabeled consensus NF-κB oligonucleotide was added, lanes 4–10: PMs were treated with none (control), LPS (1 µg/mL), LPS + vehicle; LPS + diclofenac sodium (DS) (10 µM), or LPS + PRP extracts (31.25, 62.5, and 125 µg/mL), respectively.
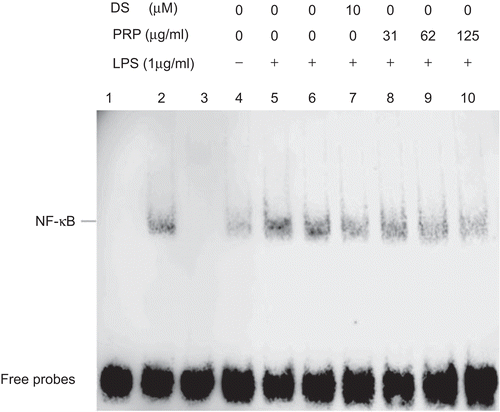
Discussion
PRP have been proven to have potent anti-inflammatory effects in vitro and in vivo (CitationWang et al., 2008; CitationBai et al., 2009). In our previous study, we found that extracts of PRP effectively decreased NO production in LPS-stimulated PMs, which attributed to the inhibition of the transcription of iNOS mRNA (CitationLiu et al., 2009). In this research, the effects of PRP extracts on proinflammatory cytokines, such as TNF-α and IL-1β, and COX-2 and 5-LOX pathways in macrophages were investigated.
Macrophages are extraordinarily versatile cells as sentinels of the immune system. They detect pathogenic substances through pattern-recognition receptors and subsequently initiate and regulate inflammatory response by releasing proinflammatory cytokines, such as TNF-α, IL-1β, and IL-6, which have various proinflammatory effects in chromic inflammatory diseases such as rheumatoid arthritis and atherosclerosis (CitationAndreakos et al., 2004), and other inflammatory mediators as NO and PGE2, which recruit additional immune cells to sites of infection or tissue injury (CitationBoscá et al., 2005). In this study, LPS was used as the prototypical inflammatory stimulus because of its ability to initiate a range of proinflammatory mediators. PRP extracts significantly inhibited LPS-induced TNF-α and IL-1β production in PMs in a dose-dependent manner, which demonstrated that PRP extracts exerted anti-inflammatory effects via inhibiting the generation of proinflammatory cytokines that are paramount in generation of an inflammatory response in activated macrophages.
In general, PGE2 is considered the key inflammatory mediator generated from AA via COX-2, which is primarily limited in immune and nervous systems, where it is induced in response to a variety of inflammatory stimuli, and contributes to the development of many chronic inflammatory diseases, such as cardiovascular disease, cancer, and rheumatoid arthritis (CitationWilloughby et al., 2000; CitationWang et al., 2007). In contrast, COX-1 is usually regarded as constitutive expression and responsible for “housekeeping” functions, such as gastric cytoprotection and regulation of platelet aggregation. Therefore, drugs inhibiting COX-1 or COX-2, such as NSAIDs and selective COX-2 inhibitors, can prevent the production of PGs. Conversely, drugs restraining the generation of PGs should influence COX. In this study, it was first validated that PRP extracts could inhibit the production of PGE2. Following results showed that LPS notably increased the levels of COX-2 mRNA and protein expression after 18 h incubation. Moreover, the results also showed that DS notably inhibited PGE2 production, but had negligible influence on mRNA and protein expressions of COX-2. These consequences suggested that DS may prefer to inhibit the activity of COX rather than inhibit the expression of COX. Differently, PRP extracts could not only inhibit PGE2 production, but also reduce COX-2 mRNA and protein expression significantly in PMs stimulated by LPS. And the anti-inflammatory effects may be due to the main active constituent contained in PRP, luteolin, which has been shown to have inhibitory effects on COX-2 protein expression and PGE2 formation (CitationHarris et al., 2006).
On the other hand, AA can be metabolized by 5-LOX. Among them, 5-LOX is the most important. 5-LOX exists in cells, such as neutrophils, macrophages, and basophils, which are involved in inflammatory response. The liberated free AA is oxygenated at C-5 and subsequently dehydrated by 5-LOX, yielding the intermediate LTA4, which then be hydrolyzed by LTA4 hydrolase (LTA4H) to LTB4. LTB4 has potent chemotactic and activating effects for leukocytes, and exhibits strong proinflammatory activity in cardiovascular tissues and enhances vascular permeability and endothelial dysfunction. Early studies revealed that among the 5-LOX family, 5-LOX had the unique ability to translocate to nuclear membranes following activated by Ca2+, fMLP, PAF, and so on (CitationFlamand et al., 2006). The translocation is related to the action of FLAP, located in the nuclear membrane. In this study, the result showed that challenge of PMs with Ca2+ ionophore A23187 conduced to the translocation of 5-LOX protein to nuclear and enhanced the activity of 5-LOX, which resulted in the increase of LTB4 production, but had no influence on the total 5-LOX protein expression. Moreover, A23187 had no effect on the expression of 5-LOX, FLAP, and LTA4H mRNA. In the meanwhile, PRP extracts showed no influence not only on the gene expression mentioned above, but also on total 5-LOX protein expression and the translocation of 5-LOX. So it is possible that PRP may inhibit the production of LTB4 via interfering on the activity of 5-LOX instead of on the expression of 5-LOX.
Most inflammatory mediators or their metabolizing enzymes, such as IL-1β, TNF-α, COX-2, and iNOS, are mainly regulated at the transcriptional level. In macrophages, LPS activates NF-κB, which leads to the expression of many immediate early genes. The stimulation of macrophages by LPS goes through a cascade of events leading to the activation of inhibitor κB (IκB) kinase (IKK), which phosphorylates IκB, following the degradation and translocation of NF-κB to the nucleus (CitationGriscavage et al., 1996). Once NF-κB translocates to the nucleus, it will induce gene transcription through special cis-acting κB element, which has been demonstrated in COX-2, iNOS, and TNF-α genes. Our previous study has showed that PRP could inhibit NO production by decreasing the expression of iNOS gene and protein, abolish partially the LPS-induced degradation of IκB-α, and inhibit translocation of NF-κB p65 to the nucleus. It was found in this study that PRP could further inhibit LPS-induced DNA-binding activity of NF-κB p65. All of the evidences above suggested that the effects of PRP extracts may be associated with inhibition of NF-κB activation stimulated by LPS.
Based on this study, it is interesting to compare PRP with other anti-inflammatory drugs. Traditional NSAIDs and selective COX-2 inhibitors can inhibit the activity of COX-1 and COX-2 and the following production of PGE2, which can explain their anti-inflammatory mechanisms. However, the inhibition of COX-2 activity and PGE2 production might increase the levels of LTB4, which would affect another inflammatory pathway (CitationMartel-Pelletier et al., 2004). So in recent years, several new dual inhibitors of COX/5-LOX activity such as licofelone have been investigated. This drug can simultaneously inhibit platelet function, PGE2 and LTB4 production associated with COX-1, COX-2, and 5-LOX, respectively (CitationHuang et al., 2004). To date, available clinical data have shown that licofelone has more advantages than conventional NSAIDs (CitationRaynauld et al., 2009). In this study, we found PRP extracts also significantly inhibited the production of LTB4 induced by A23187 and the production of PGE2 induced by LPS. But their mechanisms seem to be different from each other; licofelone inhibited the expression of 5-LOX protein (CitationVidal et al., 2007), whereas PRP decreased the production of LTB4 without interfering with the expression of 5-LOX gene and protein and the translocation of 5-LOX stimulated by A23187. According to our present understanding, this is the first investigation that PRP extracts display dual COX/5-LOX inhibitory effects and markedly inhibit PGE2 and LTB4 production in macrophages. So, the mechanisms of PRP extracts on anti-inflammation not only come from the inhibition on the production of proinflammatory cytokines, iNOS/NO pathway, but also COX-2/PGE2 and 5-LOX/LTB4 pathway. Our findings here provide useful and additional mechanistic explanations for the anti-inflammatory effect of PRP and highlight its pharmaceutical value.
Declaration of interest
This work was supported by a grant (Grant No. 2007BAI31B04) from National Key Technology R&D Program of China.
References
- Andreakos E, Foxwell B, Feldmann M. (2004). Is targeting Toll-like receptors and their signaling pathway a useful therapeutic approach to modulating cytokine-driven inflammation? Immunol Rev, 202, 250–265.
- Bai H, Li Z, Chen WW, Zhong X, Zhang YY. (2009). Effect and mechanism of Qizhengxiaotong Emplastrum in external application for relieving cancer pain. J Beijing Univ Tradit Chin Med, 16, 1–5.
- Boscá L, Zeini M, Través PG, Hortelano S. (2005). Nitric oxide and cell viability in inflammatory cells: A role for NO in macrophage function and fate. Toxicology, 208, 249–258.
- Du ZH. (2007). Clinical application and adverse effects of Cheezheng pain relieving plaster. Mod J Integr Chin Tradit West Med, 16, 1586–1588.
- Flamand N, Lefebvre J, Surette ME, Picard S, Borgeat P. (2006). Arachidonic acid regulates the translocation of 5-lipoxygenase to the nuclear membranes in human neutrophils. J Biol Chem, 281, 129–136.
- Griscavage JM, Wilk S, Ignarro LJ. (1996). Inhibitors of the proteasome pathway interfere with induction of nitric oxide synthase in macrophages by blocking activation of transcription factor NF-kappa B. Proc Natl Acad Sci USA, 93, 3308–3312.
- Harris GK, Qian Y, Leonard SS, Sbarra DC, Shi X. (2006). Luteolin and chrysin differentially inhibit cyclooxygenase-2 expression and scavenge reactive oxygen species but similarly inhibit prostaglandin-E2 formation in RAW 264.7 cells. J Nutr, 136, 1517–1521.
- He CY, Li N, Chen LH, Ma J, Li WD. (2008), Therapeutical effect of Cheezheng pain relieving plaster on acute soft tissue injury. J Tradit Chin Orthop Traumatol, 20, 9–12.
- Huang H, Park PH, McMullen MR, Nagy LE. (2008). Mechanisms for the anti-inflammatory effects of adiponectin in macrophages. J Gastroenterol Hepatol, 23(Suppl. 1), S50–S53.
- Huang L, Zhao A, Wong F, Ayala JM, Struthers M, Ujjainwalla F, Wright SD, Springer MS, Evans J, Cui J. (2004). Leukotriene B4 strongly increases monocyte chemoattractant protein-1 in human monocytes. Arterioscler Thromb Vasc Biol, 24, 1783–1788.
- Kim JB, Han AR, Park EY, Kim JY, Cho W, Lee J, Seo EK, Lee KT. (2007). Inhibition of LPS-induced iNOS, COX-2 and cytokines expression by poncirin through the NF-kappaB inactivation in RAW 264.7 macrophage cells. Biol Pharm Bull, 30, 2345–2351.
- Lee SJ, Kim CE, Seo KW, Kim CD. (2010). HNE-induced 5-LO expression is regulated by NF-κB/ERK and Sp1/p38 MAPK pathways via EGF receptor in murine macrophages. Cardiovasc Res, 88, 352–359.
- Leone S, Ottani A, Bertolini A. (2007). Dual acting anti-inflammatory drugs. Curr Top Med Chem, 7, 265–275.
- Li M, Jia Z, Zhang R. (2004). A review on the study of Lamiophlomis rotata (Benth.) Kudo: An analgesic and hemostatic drug. J Chin Med Mater, 27, 222–224.
- Liu Y, Peng SY, Wang L, Wang WJ. (2009). [Effects of extracts of Cheezheng pain relieving plaster on nitric oxide and iNOS expression in macrophages induced by lipopolysaccharides]. Yao Xue Xue Bao, 44, 863–867.
- Martel-Pelletier J, Mineau F, Fahmi H, Laufer S, Reboul P, Boileau C, Lavigne M, Pelletier JP. (2004). Regulation of the expression of 5-lipoxygenase-activating protein/5-lipoxygenase and the synthesis of leukotriene B(4) in osteoarthritic chondrocytes: Role of transforming growth factor beta and eicosanoids. Arthritis Rheum, 50, 3925–3933.
- Maxis K, Delalandre A, Martel-Pelletier J, Pelletier JP, Duval N, Lajeunesse D. (2006). The shunt from the cyclooxygenase to lipoxygenase pathway in human osteoarthritic subchondral osteoblasts is linked with a variable expression of the 5-lipoxygenase-activating protein. Arthritis Res Ther, 8, R181.
- Notoya M, Nishimura H, Woo JT, Nagai K, Ishihara Y, Hagiwara H. (2006). Curcumin inhibits the proliferation and mineralization of cultured osteoblasts. Eur J Pharmacol, 534, 55–62.
- Raynauld JP, Martel-Pelletier J, Bias P, Laufer S, Haraoui B, Choquette D, Beaulieu AD, Abram F, Dorais M, Vignon E, Pelletier JP; Canadian Licofelone Study Group. (2009). Protective effects of licofelone, a 5-lipoxygenase and cyclo-oxygenase inhibitor, versus naproxen on cartilage loss in knee osteoarthritis: A first multicentre clinical trial using quantitative MRI. Ann Rheum Dis, 68, 938–947.
- Shen F, Bai AP, Guo ZR, Cheng GF. (2002). Inhibitory effect of 3,4-diaryl-3-pyrrolin-2-one derivatives on cyclooxygenase 1 and 2 in murine peritoneal macrophages. Acta Pharmacol Sin, 23, 762–768.
- Vidal C, Gómez-Hernández A, Sánchez-Galán E, González A, Ortega L, Gómez-Gerique JA, Tuñón J, Egido J. (2007). Licofelone, a balanced inhibitor of cyclooxygenase and 5-lipoxygenase, reduces inflammation in a rabbit model of atherosclerosis. J Pharmacol Exp Ther, 320, 108–116.
- Wang MT, Honn KV, Nie D. (2007). Cyclooxygenases, prostanoids, and tumor progression. Cancer Metastasis Rev, 26, 525–534.
- Wang YZ, Guo CY, Zhong HG, Zhang WN, Wang DL, Wang X, Dong FH. (2008). In vivo effects of pain relieving plaster on closed soft tissue injury in rabbit ears. BMC Complement Altern Med, 8, 51.
- Willoughby DA, Moore AR, Colville-Nash PR. (2000). COX-1, COX-2, and COX-3 and the future treatment of chronic inflammatory disease. Lancet, 355, 646–648.
- Xu RM, Zhang SR, Zhang YS. (2004). Study on Qizhengxiaotongtiefen in anti-inflammation and analgesia. Chin J Trad West Med, 5, 12–15.
- Yan ZW, Ai SY. (2008). Clinical observation of triamcinolone acetonide and Cheezheng pain relieving plaster on rheumatoid gonarthritis. Med Innov China, 5, 64–65.
- Yu G, Praveen Rao PN, Chowdhury MA, Abdellatif KR, Dong Y, Das D, Velázquez CA, Suresh MR, Knaus EE. (2010). Synthesis and biological evaluation of N-difluoromethyl-1,2-dihydropyrid-2-one acetic acid regioisomers: Dual inhibitors of cyclooxygenases and 5-lipoxygenase. Bioorg Med Chem Lett, 20, 2168–2173.
- Zaitsu M, Hamasaki Y, Matsuo M, Kukita A, Tsuji K, Miyazaki M, Hayasaki R, Muro E, Yamamoto S, Kobayashi I, Ichimaru T, Kohashi O, Miyazaki S. (2000). New induction of leukotriene A(4) hydrolase by interleukin-4 and interleukin-13 in human polymorphonuclear leukocytes. Blood, 96, 601–609.
- Zhao K. (2010). Clinical observation of Cheezheng pain relieving plaster on osteoarthritis. Cap Med, 14, 49.
- Zhen Y, Cai JY, Jürgen CA. (2006). Comments on China’s Tibetan Medicine. Chin J Integr Trad West Med, 12, 152–153.