Abstract
Context: Artemisia annua L. (Asteraceae), commonly known as sweet wormwood or Qinghao, is an annual herb/shrub native of Asia. The plant grows broadly in Caspian Sea shores in North of Iran. In China, the aerial parts of this plant are source of artemisinin, which is an antimalarial compound.
Objective: This study aimed to establish the scientific basis of reported ethnomedicinal use of A. annua as sedative agent.
Material and methods: The plants were gathered from Gilan Province in Iran. Plant aerial parts were extracted with methanol and concentrated in vacuum. Methanol extract was partitioned into chloroform, petroleum ether, and ethyl acetate. Each fraction was administered intraperitoneally (i.p.) in male mice with different concentrations (50, 100, and 200 mg/kg), and for evaluation of sedative activity, immobility time was determined. In effort to clarify the mechanism of action, flumazenil (3 mg/kg, i.p.) as a benzodiazepine (BZD) receptor antagonist was injected 15 min before chloroform fraction (200 mg/kg, i.p.).
Results: Compared with control group (saline-treated mice), the chloroform fraction significantly increased immobility time in a dose-dependent manner. Flumazenil decreased immobility time induced by chloroform fraction significantly.
Discussion and conclusion: The results of the present study suggest that A. annua growing in Iran has sedative effects, which are probably mediated via BZD receptors pathways.
Introduction
The identification of biologically active molecules from 250,000 higher plant species (CitationBalouchnejad Mojarad et al., 2005) used as remedies in traditional folk medicine can be important to find their mechanism(s) of action in biological studies. Artemisia annua L. (Asteraceae) commonly known as sweet wormwood or Qinghao is an annual herb/shrub native of Asia (CitationWright, 2002). A. annua growing broadly in north of Iran has been used traditionally for infants as an antispasmodic, carminative, or sedative remedy. The plant grows wild in Europe and America and on a large scale in China, Iran, Turkey, Afghanistan, and Australia (CitationBhakuni et al., 2001). A. annua essential oil with antioxidant (CitationJuteau et al., 2002), antibacterial, and antifungal effects (CitationWoerdenbag et al., 1993) has been used in cosmetics, perfumery, and aromatherapy. The essential oil of this plant is generally between 0.3% and 1.4% (v/w) and principle constituents are camphor, germacrene D, artemisia ketone, artemisia alcohol, linalool, cineol, p-cymene, and thujone (CitationWoerdenbag et al., 1993; CitationJuteau et al., 2002; CitationPerazzo et al., 2003).
The aerial parts of A. annua are source of artemisinin that has potent antimalarial activity against chloroquine and quinine-resistant Plasmodium falciparum and other malaria-causing parasites. More than 3000 malarial patients infected with P. falciparum and P. vivax were clinically cured by artemisinin and its derivatives. These compounds are also effective in cerebral malaria (CitationBhakuni et al., 2001).
Beside antimalarial effects, A. annua has biological activities such as antibacterial, anti-inflammatory, angiotensin-converting enzyme inhibitory, cytokinin-like and antitumor effects (CitationBhakuni et al., 2001). These activities related to the secondary metabolites such as sesquiterpenoids, flavonoids, coumarins, phenolics, purines, triterpenoids, steroid, and monoterpenoids (CitationBhakuni et al., 2001).
Artemisinic acid is a well-known precursor for semisynthesis of artemisinin, which has shown antibacterial activity (CitationRoth and Acton, 1989; CitationBhakuni et al., 2001).
Scopoletin is a coumarin isolated from A. annua, which has been reported to possess anti-inflammatory activity (CitationHuang et al., 1993; CitationBhakuni et al., 2001). Artemisinin, dihydroartemisinin, and arteether have been found to exhibit marked suppression of humeral responses in mice at high dose. The significant cytotoxic activity of artemisinin and quercetagetin-6,7,3′,4′-tetramethylether has been reported against some tumor cells (CitationZheng, 1994). The flavonoids of fisetin and patuletin-3,7-dirhamnoside, isolated from A. annua, were found to be nonpeptide angiotensin-converting enzyme inhibitors (CitationLin et al., 1994). The use of A. annua in the treatment of cerebral malaria raises the prospect of other possible central nervous system (CNS) effects. The medicines that are used for the treatment of cerebral malaria have to pass the blood–brain barrier freely (CitationGenovese et al., 1998). CitationAmos et al. (2003) showed that artemisinin in A. annua increased pentobarbital-induced hypnosis in rats and decreased exploratory behavior in mice. In addition, artemisinin reduced spontaneous motor activity in mice and decreased bromocripine-induced hyperactivity in reserpinized mice (CitationAmos et al., 2003). Some evidence indicated that sedative property of artemisinin may be mediated via postsynaptic dopamine D2 receptor antagonism in the brain (CitationAmos et al., 2003). CitationPerazzo et al. (2003) in another study concluded that both essential oil and crude ethanol extract of A. annua have a depressant activity. The involvement of cholinergic and dopaminergic systems for essential oil and ethanol extract of A. annua has been indicated in CitationPerazzo et al. (2003).
The present study was therefore designed to evaluate the sedative effects and its possible pharmacological mechanism(s) of fractions obtained from aerial parts of A. annua growing in north of Iran.
Materials and methods
Animals
Male albino mice weighing 25–30 g were purchased from Pasteur Institute (Tehran, Iran) and used in this study. The mice bred in the Faculty of Pharmacy, Tehran University of Medical Sciences (TUMS), placed in individual stainless-steel cages, handled daily, and given pellet food and tap water ad libitum. A 12 h light/dark cycle was maintained, and the animals were tested during the light cycle. The study was conducted in accordance with the recommendations and approval principles of the Ethics Committee on Animal Experiments of TUMS.
Chemicals
The chemicals used for the experiments in this study are: diazepam (Tamin Pharmaceutical Co., Rasht, Iran), flumazenil (Sigma, St. Louis, MO), Tween 80 and DMSO (Merck Chemical Co., Darmstadt, Germany).
Plant material
The aerial parts of sweet wormwood were collected in July 2007 before blooming time from an area around Rasht city in north of Iran. Voucher specimen (number TEH-6692) was identified and deposited by Dr. G. Amin at the Herbarium of Faculty of Pharmacy, TUMS, Tehran, Iran.
Preparation of extract
The dried aerial parts (745 g) of A. annua was powdered and extracted by Soxhlet apparatus with methanol. The extract concentrated with evaporator yielded 193.7 g (26%) dried material. This extract was fractionated with petroleum ether, chloroform, and ethyl acetate, which yielded 91, 31, and 27 g of related fractions, respectively, and a 44 g of methanol soluble residue.
Pharmacological tests
Each fraction was administered intraperitoneally (i.p.) to each group of mice in different doses (50, 100, and 200 mg/kg). Diazepam was injected in another group as a positive control.
The sedative effect was determined by direct observation of mice immobility time in open field system. More specifically, the animal behavior in the test box was recorded by a video-tracking system (Ethovision version 3.1; Noldus Information Technology, Wageningen, The Netherlands), and the accumulative immobility time was assessed during 60 min. Measuring the immobility time was stopped immediately during any movement of the animals. Since the immobility was the predominant observed behavior in mice, thus, this effect of the fractions was evaluated in our study. The sedative effects of the fractions were measured and statistically compared with control.
Animals were divided into six groups and the following solutions were injected i.p. in each group (n = 7):
NS/DMSO: 20% dimethyl sulfoxide (DMSO) in normal saline (NS) plus a few drops of Tween 80 as vehicle of fractions (control animals).
Diazepam (3 mg/kg, i.p.) as positive control (CitationHelton et al., 1996; CitationNogueria and Vassilieff, 2000; CitationAkanmu et al., 2005; CitationRakhshandah et al., 2007).
Petroleum ether fraction with three concentrations (50, 100, and 200 mg/kg).
Chloroform fraction (50, 100, and 200 mg/kg).
Ethyl acetate fraction (200 mg/kg).
Methanol fraction (200 mg/kg).
To describe the pharmacological sedative mechanism of A. annua, flumazenil (3 mg/kg) (CitationAkanmu et al., 2005) as a benzodiazepine (BZD) receptor antagonist was administered i.p. 15 min before chloroform fraction (200 mg/kg) as the most effective fraction, for studying the role of GABA receptor pathways in sedative effects of fractions.
Statistical analysis
All data were expressed as mean ± SEM. Comparison of immobility time in all groups were made using one-way analysis of variance (ANOVA), followed by Newman-Keuls post hoc test. Differences with P < 0.05 were considered statistically significant.
Results
Comparison of the fractions on the sedative effect
Administration of the chloroform fraction (50, 100, and 200 mg/kg) induced significant (***P < 0.001) sedation in comparison with control group. As shown in , the effect of chloroform fraction is dose-dependent and the maximum response was obtained with 200 mg/kg. Injection of diazepam (3 mg/kg, i.p.) caused more sedative effect (***P < 0.001) than chloroform fraction-treated mice ().
Figure 1. Effect of the chloroform fraction of A. annua extract (50, 100, and 200 mg/kg, i.p.) on duration of immobility. Administration of chloroform fraction increased immobility time as sedative effect in a dose-dependent manner. Treatment of animals with diazepam (3 mg/kg, i.p.) as positive control induced sedation significantly (***P < 0.001). Data are presented as mean ± SEM of seven mice. ***P < 0.001 shows significant difference with control group. †P < 0.001 compared the statistical difference with diazepam-treated mice (positive control).
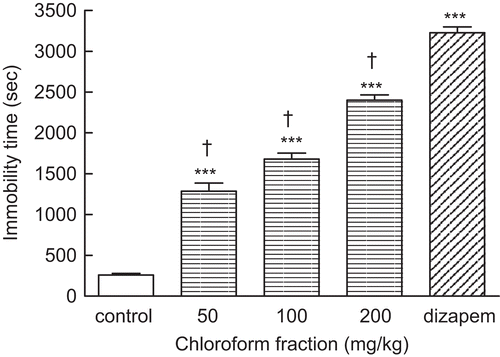
The immobility time of animal groups receiving 50, 100, and 200 mg/kg of the petroleum ether fraction was significantly greater than control mice (***P < 0.001) (). The dose-dependent effect of petroleum ether fraction is shown in .
Figure 2. Effect of the petroleum fraction of A. annua extract (50, 100, and 200 mg/kg, i.p.) on duration of immobility. Administration of petroleum fraction increased immobility time as sedative effect. Treatment of animals with diazepam (3 mg/kg, i.p.) as positive control induced sedation significantly (***P < 0.001). Data are presented as mean ± SEM of seven mice. ***P < 0.001 shows significant difference with control group. †P < 0.001 compared the statistical difference with diazepam-treated mice (positive control).
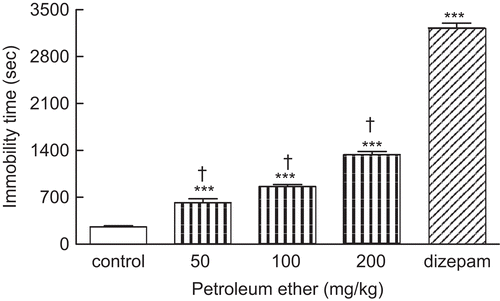
Intraperitoneal administration of either ethyl acetate or methanol fractions with dose of 200 mg/kg did not cause any significant effect compared with control group (). Injections of lower doses (50 and 100 mg/kg) also did not induce any significant changes (data not shown).
Figure 3. Time course effects of chloroform, petroleum ether, methanol, and ethyl acetate fractions of A. annua extract on immobility duration in mice. Immobility time was recorded as sedation during four quarters (15 min). The maximum response was obtained in first quarter and with chloroform fraction. Data are presented as mean ± SEM of seven mice. ***P < 0.001 shows significant difference with respective control group.
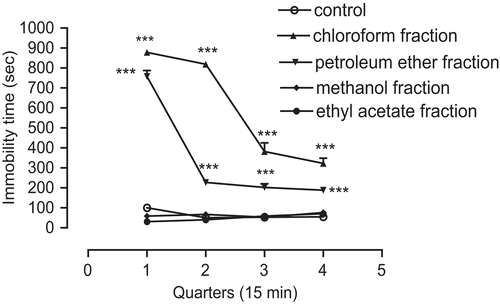
Time course effects of high concentrations of all fractions are compared in . The chloroform fraction shows significant difference (***P < 0.001) during the four quarters (15 min) compared with control animals ().
Effect of flumazenil on immobility time induced by chloroform fraction
As it is presented in , flumazenil (3 mg/kg, i.p.) decreased the sedation time induced by chloroform fraction significantly (*P < 0.05). Pretreatment of animals with flumazenil (3 mg/kg, i.p.) also caused significant (**P < 0.01) reduction in sedation induced by diazepam (3 mg/kg, i.p.).
Figure 4. Effect of flumazenil (3 mg/kg, i.p.) on chloroform fraction-induced immobility. Pretreatment of animals with flumazenil (3 mg/kg, 15 min, i.p.) caused significant (#P < 0.05) reduction in immobility time induced by chloroform fraction (200 mg/kg, i.p.). Administration of flumazenil (3 mg/kg, i.p.) also decreased sedation induced by diazepam (3 mg/kg, i.p.) significantly (†P < 0.01). Data are presented as mean ± SEM of seven animals in each group. The abbreviations used are as follows: Dz: diazepam, Cl: chloroform fraction, Fl: flumazenil. ***P < 0.001 shows significant difference with control group. †P < 0.01 compared the statistical difference with diazepam-treated mice (positive control). #P < 0.05 indicates the difference with chloroform fraction.
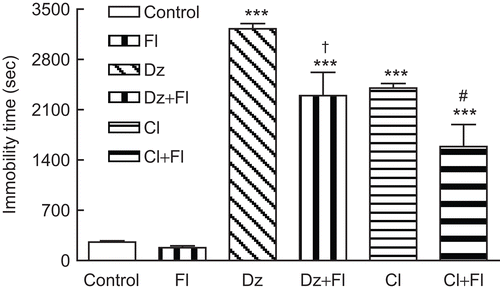
Discussion
BZDs, which are the most commonly consumed medicines for the management of sleep and related problems, facilitate sleep throughout the night with no effect by the following morning. Despite advances in the development of newer hypnotics in modern medicine, a significant proportion of patients with insomnia use herbal hypnotic regularly (CitationAncoli-Israel & Roth, 1999; CitationKumar & Kalonia, 2008). Hence, finding a candidate molecule for the better management of sleep could be rewarding.
Searching for novel pharmacotherapy from medicinal plants for psychiatric problems has progressed significantly in the past decade. A noticeable number of herbal components whose behavioral and pharmacological effects have been well-characterized may be good candidates for more investigations that may ultimately lead to clinical use. There are an increasing number of herbal products, which have been introduced into psychiatric practice in the past decade (CitationEun et al., 2006; CitationMa et al., 2009).
The data of the present work showed that there was a prolongation of the immobility time that indicated significant inhibition on CNS activity in groups who were treated by chloroform and petroleum ether fractions of A. annua.
Our findings are in accordance with previous studies showing that essential oil and the ethanol extract from A. annua caused strong depressant activity on the CNS (CitationPerazzo et al., 2003, Citation2008). In our investigation, all three doses of both chloroform and petroleum ether fractions of A. annua (50, 100, 200 mg/kg) significantly caused immobility, and the effects of high dose of chloroform fraction were predominant. Compared with the diazepam as a sedative agent, both chloroform and petroleum ether fractions induced sedative response but not as potent as diazepam. Regarding the depressant activities of both extracts, especially chloroform fraction, it is possible that the sedative effects are mediated through interaction with inhibitory neurotransmitter receptors. Moreover, the present results suggest that both fractions may contain different potent compounds that induce sedation via various signaling pathways. Regarding previously published reports, the central inhibitory effects of A. annua may be related to direct or indirect interaction with cholinergic or dopaminergic receptors in the brain (CitationAmos et al., 2003; CitationPerazzo et al., 2003, CitationPerazzo et al., 2008).
One of the major findings of the current work is that pretreatment of animals with flumazenil as BZD receptors antagonist attenuated chloroform fraction-induced sedation significantly (P < 0.05).
Considering the pharmacological mechanism of flumazenil on blockade of GABA receptors, it is reasonable to deduce that the depressant effects of chloroform fraction are probably mediated by interactions with the BZD-signaling mechanism. It is suggested that the phytoconstituents that are present in non-polar fraction of A. annua can be responsible for its sedative activity. It is also possible that chloroform fraction contains BZD-like compounds that act via GABA pathway.
The essential oil of this plant, which is a part of non-polar fraction (chloroform and petroleum ether), is mainly composed of camphor, 1,8-cineol, linalool, p-cymene, thujone (CitationWoerdenbag et al., 1993; CitationJuteau et al., 2002; CitationPerazzo et al., 2003), and myrcene (CitationGoel et al., 2007). The central effects of these compounds with high lipid solubility have been investigated in some studies presenting its action on the CNS-related behaviors (CitationRobbers et al., 1996). Linalool, a major monoterpenoid present in aromatic plants, is shown to have sedative, anticonvulsant (CitationElisabetsky et al., 1995), and high depressive activity in animal and human studies (CitationSugawara et al., 1998; CitationElisabetsky et al., 1999). In addition, Lavandula angustifolia, the oil of which contains linalool, is used as a mild sedative and exhibits depressive activity on experimental animals (CitationBlumenthal et al., 2000). There are also some reports indicating a possible inhibitory effect on GABA transaminase by essential oils (CitationKoo et al., 2003). These compounds may be considered as the candidates for the observed effects of A. annua.
In the present work, we assessed the sedative activity of various fractions of methanol extract of A. annua. There are also some reports that show the effects of the essential oil and crude ethanol extract of this plant on cholinergic and dopaminergic pathways in the CNS (CitationAmos et al., 2003; CitationPerazzo et al., 2003). Regarding the different types of extracts, it is possible that the effective compounds of extracts or fractions affect behavioral activities via interactions with various pharmacological mechanism(s).
Further work is presently on going in our laboratory to isolate and identify the active components.
Conclusion
In conclusion, the behavioral results obtained in the current work are in accordance with the ethnopharmacological use of A. annua and support traditional use of this plant as a sedative agent. The non-polar fractions (chloroform and petroleum ether) of A. annua methanol extract increased immobility time as sedative effect. The pharmacological profile of effective components of A. annua might resemble that of BZD receptor agonists such as diazepam. Considering the inhibitory effect of flumazenil on chloroform fraction-induced sedation, the involvement of GABAergic system in their sedative mechanism might be possible.
However, our findings showed the CNS depressant effects of A. annua. Understanding the molecular mechanism(s) of sedative effects of A. annua and its biologically active compounds require more studies, which will be done in our future investigations.
Acknowledgement
The authors would like to thank the authorities of Pharmacy Faculty, TUMS for its financial support.
Declaration of interest
The authors report no declaration of interest.
References
- Akanmu MA, Olayiwola G, Ukponmwan OE, Honda K. (2005). Acute toxicity and sleep-wake EEg analysis of Stachtarpheta cayennensis (Verbenaceae) in rodents. Afr J Tradit Complem, 2, 222–232.
- Amos S, Chindo BA, Abbah J, Vongtau HO, Edmond I, Binda L, Akah PA, Wambebe C, Gamaniel KS. (2003). Postsynaptic dopamine (D(2))-mediated behavioural effects of high acute doses of artemisinin in rodents. Brain Res Bull, 62, 255–260.
- Ancoli-Israel S, Roth T. (1999). Characteristics of insomnia in the United States: Results of the 1991 National Sleep Foundation Survey. I. Sleep, 22 (Suppl 2), S347–S353.
- Balouchnejad Mojarad T, Roghani M, Zare N. (2005). Effect of subchronic administration of aqueous Artemisia annua extract on α1-adrenoceptor agonist-induced contraction of isolated aorta in rat. Iranian Biomed J, 9, 57–62.
- Bhakuni RS, Jain DC, Sharma RP, Kumar S. (2001). Secondary metabolites of Artemisia annua and their biological activity. Curr Sci, 80, 35–48.
- Blumenthal M, Goldberg A, Brinkmann J, eds. (2000). Herbal Medicine. Expanded Commission E Monographs. Newton, MA: Integrative Medicine Communications, pp. 75–77, 227.
- Elisabetsky E, Brum LF, Souza DO. (1999). Anticonvulsant properties of linalool in glutamate-related seizure models. Phytomedicine, 6, 107–113.
- Elisabetsky E, Coelho de Souza GP, dos Santos MAC, Siqueira IR, Amador TA. (1995). Sedative properties of linalool. Fitoterapia, LXV, 407–414.
- Eun JS, Kwon HN, Hong JT, Oh KW. (2006). Inhibitory effects of (−)-epigallocatechin gallate on morphine-induced locomotor sensitization and conditional place preference in mice. J Appl Pharmacol, 14, 125–131.
- Genovese RF, Newman DB, Petras JM, Brewer TG. (1998). Behavioral and neural toxicity of arteether in rats. Pharmacol Biochem Behav, 60, 449–458.
- Goel D, Singh V, Ali M, Mallavarupu MR, Kumar S. (2007). Essential oils of petal, leaf and stem of the antimalarial plant Artemisia annua. J Nat Med, 61, 187–191.
- Helton DR, Berger JE, Czachura JF, Rasmussen K, Kallman MJ. (1996). Central nervous system characterization of the new cholecystokinin B antagonist LY288513. Pharmacol Biochem Behav, 53, 493–502.
- Huang L, Liu JF, Liu LX, Li DF, Zhang Y, Nui HZ, Song HY, Zhang CY. (1993). [Antipyretic and anti-inflammatory effects of Artemisia annua L]. Zhongguo Zhong Yao Za Zhi, 18, 44–48, 63.
- Juteau F, Masotti V, Bessière JM, Dherbomez M, Viano J. (2002). Antibacterial and antioxidant activities of Artemisia annua essential oil. Fitoterapia, 73, 532–535.
- Koo BS, Park KS, Ha JH, Park JH, Lim JC, Lee DU. (2003). Inhibitory effects of the fragrance inhalation of essential oil from Acorus gramineus on central nervous system. Biol Pharm Bull, 26, 978–982.
- Kumar A, Kalonia H. (2008). Effect of Withania somnifera on sleep–wake cycle in sleep-disturbed rats: Possible GABAergic 22echanism. Indian J Pharm Sci, 70, 806–810.
- Lin JY, Chen TS, Chen CS. (1994). Flavonoids as nonpeptide angiotensin-converting enzyme inhibitors for hypertension treatment. Jpn-Kokai Tokkyo Koho Jp, Patent No. 06135830.
- Ma Y, Ma H, Eun JS, Nam SY, Kim YB, Hong JT, Lee MK, Oh KW. (2009). Methanol extract of Longanae arillus augments pentobarbital-induced sleep behaviors through the modification of GABAergic systems. J Ethnopharmacol, 122, 245–250.
- Nogueria E, Vassilieff VS. (2000). Hypnotic, anticonvulsant and muscle relaxant effects of Rubus brasiliensis. Involvement of GABAA system. J Ethnopharmacol, 70, 275–280.
- Perazzo FF, Carvalho JC, Carvalho JE, Rehder VL. (2003). Central properties of the essential oil and the crude ethanol extract from aerial parts of Artemisia annua L. Pharmacol Res, 48, 497–502.
- Perazzo FF, Lima LM, Maistro EL, Carvalho JE, Rehder VLG, Carvalho JCT. (2008). Effect of Artemisia annua L. leaves essential oil and ethanol extract on behavioral assays. Rev Bras Farmacogn, 18, 686–689.
- Rakhshandah H, Shakeriand MT, Ghasemzadeh MR. (2007). Comparative hypnotic effect of Rosa damascena fractions and diazepam in mice. Iranian J Pharm Res, 6, 193–197.
- Robbers JE, Speedie MK, Tyler VE. (1996). Pharmacognosy and Pharmacobiotechnology. Baltimore, MD: Williams & Wilkins.
- Roth RJ, Acton N. (1989). A simple conversion of artemisinic acid into artemisinin. J Nat Prod, 52, 1183–1185.
- Sugawara Y, Hara C, Tamura K, Fujii T, Nakamura T, Aoki T. (1998).Sedative effect on humans of inhalation of essential oil of linalool: sensory evaluation and physiological measurement using optically active linalool. Anal Chim Acta, 365, 293–299.
- Woerdenbag HJ, Bos R, Salomons MC, Hendriks H, Pras N, Malingré TM. (1993). Volatile constituents of Artemisia annua L. (Asteraceae). Flavour Frag J, 8, 131–137.
- Wright CW. (2002). Artemisia. London, UK: Taylor & Francis, pp. 107–117.
- Zheng GQ. (1994). Cytotoxic terpenoids and flavonoids from Artemisia annua. Planta Med, 60, 54–57.