Abstract
Context: Monosialotetrahexosylganglioside (GM1) prepared from the brain of pig or bovine is an effective clinical drug in the treatment of different nervous system diseases. Generally, polysialogangliosides are transformed into GM1 by enzymic or chemical hydrolysis due to the very poor level of natural GM1.
Objective: To continuously obtain GM1 by cell immobilization in a packed-bed reactor.
Materials and methods: Brevibacterium casei, which is Gram-positive bacteria belonging to the order Actinomyces and family Brevibacteriaceae, can produce high-activity sialidase, are encapsulated in silk fibroin hydrogel, and subsequently packed into a reactor. The crude ganglioside is pumped into the reactor and continuously turned to GM1.
Results: The optimal silk fibroin concentration for hydrogel preparation is 6.0% (w/v). The optimal initial biomass for immobilization is ~12% (wet weight). The optimal conversion conditions are 35°C and 6 mL/min of flow rate. Under above conditions, the maximum GM1 productive strength and conversion ratio can reach to 4.2 g/L·h and 313.5%, respectively.
Discussion: Silk fibroin is a promising material for cell immobilization because it has predominant characteristics of higher permeability and intensity. Cell immobilization for continuous GM1 transformation could eliminate the asialo GM1 and decrease the foreign matter from transfer medium and metabolism product.
Conclusion: In the packed-bed reactor, continuous production of GM1 had been under effective running at least for 15 days indicating a potential for industrial production. It is significant that this is a first report on cell immobilization for GM1 production.
Introduction
Monosialotetrahexosylganglioside (GM1) is a component of gangliosides, which are rich in mammalian cerebral and nervous tissues, and may have applications in the treatment of Alzheimer’s disease, Parkinson’s disease, spinal-cord injury, and stroke (CitationArgentino et al., 1989; CitationGeisler et al., 1991; CitationSchneider et al., 1995; CitationSvennerholm et al., 2002). GM1 is usually prepared by hydrolyzing crude gangliosides using acid or alkali from pig or bovine’s brain in industrial scale. However, these acid or alkali treatments under high temperature generally produced by-products, mainly asialo GM1 (CitationKanfer, 1969; CitationTatsuro et al., 1989). Some studies have reported that sialidase produced by different nonpathogenic microorganisms could specially cut sialic acids from polysialogangliosides to produce GM1 (CitationFukano & Ito, 1997; CitationPeng et al., 2007). In this study, a cell immobilization technique was developed to produce GM1 in a packed-bed reactor continuously. The cell-immobilized method with microorganism producing sialidase is suitable for the preparation of GM1 in large quantities due to the characteristics of high yield and low cost.
Materials and methods
Microorganism and culture conditions
Brevibacterium casei no. 747, which is a mutant strain, produces high-activity sialidase and is restored in our center. It was cultivated in a 15-L stirred reactor containing medium of 1.0% glucose, 0.5% peptone, 0.3% yeast extract, and 0.2% K2HPO4 at 28°C, origin pH 7.5, and 0.6 vvm (air volume/culture volume/minute) of aeration.
Materials and chemicals
Silkworm cocoon used for preparation of silk fibroin hydrogel was purchased from Linyi Silk Company of China. Crude gangliosides were prepared from fresh pig brain as the methods described by CitationLin et al. (2004) and CitationKato and Hatanaka (2008). 4-Methylumbelliferyl-α-d-N-acetylneuraminic acid (4-MU-NeuAc) was purchased from Sigma Company (Santa Clara, CA). Precoated silica gel 60 thin-layer chromatography (TLC) plates were obtained from Hailang of Silica Company of Qingdao (Qingdao, China).
Whole cell immobilization with silk fibroin
Silk cocoons were shattered and then degelatinized twice in boiling water containing 0.5% (w/v) Na2CO3 for 1 h. The degelatinized silk fibroin was washed with the distilled water. The clean silk fibroin was dissolved and boiled in 50% (w/v) CaCl2 at 100°C for 10 min. The cooled silk fibroin solution dialyzed for 72 h to remove salt using 8 kDa dialysis membrane. Finally, the silk fibroin was filtered through film (φ 5 µm; Pharmacia, Piscataway, NJ) and subsequently became achroic transparent liquor. For making silk fibroin hydrogel the sterilized silk fibroin remained at 45°C and was mixed with B. casei cells. The mixture gel subsequently was added into the phosphate buffer (pH 7.0) containing 0.1% (w/v) NaCl and stirred under 8°C for 10 min. The concretionary gel was first washed with 2% (w/v) KCl and incised into block about 3–5 mm size (CitationKim et al., 2004; CitationMatsumoto et al., 2006; CitationWang et al., 2008).
Cell leakage
Cells leakage from the hydrogel matrix was determined by measuring the optical density of conversion buffer at 600 nm.
Continuous production of GM1
Cell-immobilized silk fibroin hydrogel that weighed about 5000 g (containing about 1800 g wet weight cell) were filled into two tandem glass columns (ID 100 × 1000 mm) with conversion buffer containing 0.5% glucose, 0.25% yeast extract, 0.1% K2HPO4, and 0.05% MgSO4 (pH 6.5). The schematic diagram of the packed-bed reactor is shown in . The column was maintained at 35°C with thermoexchanger. The crude polysialogangliosides were dissolved in conversion buffer and pumped into the packed-bed reactor at certain flow rate ranging from 4 to 6 mL/min.
Description of production capability and conversion ratio
To investigate the production capability of cell-immobilized technique and GM1 conversion ratio, Equations (1) and (2) were defined as follows:
QP, productive strength; YP/S, conversion ratio; S0 (g/L), initial concentration of GM1; St (g/L), final concentration of GM1 out the column; VR (L), value of packed-bed reactor; t (h), retent time of substrate; and q (L/h), flow rate.
Enzyme assay of sialidase
Sialidase activity was measured by using 4-methylumbelliferyl-N-acetylneuraminic (4-MU-NeuAc) as the substrate. The reaction mixture contained 10 µL 1 mM of 4-MU-NeuAc and a 10 µL conversion solution with 80 µL 200 mM sodium acetate buffer (pH 5). Following incubation at 37°C for 30 min, the reaction was stopped by adding 100 µL 1 M glycine–NaOH buffer (pH 10). Sialidase activity was measured by determination of absorbance at 325 nm. The control experiment was conducted with the heat-inactivated enzyme. One unit of the enzyme was defined as the amount that catalyzes the release of 1 mol of 4-MU per minute from 4-MU-NeuAc under the conditions described above (CitationTatsuro et al., 1989).
HPLC assay of GM1
The quantity of GM1 was analyzed by HPLC (Agilent 1100, Merck LichroCART-NH2 column: 5 μm 4.6 × 250 mm) method as described by CitationGandini et al. (1990). The mobile phase consisted of 66/34/8 (v/v) of acetonitrile, 0.1% phosphoric acid, and tetrahydrofuran. The constant flow rate was 1 mL/min and gangliosides and GM1 were detected with a UV detector at 210 nm.
Results and discussion
Optimization of immobilization in silk fibroin
To prepare the silk fibroin hydrogel with proper permeability and rigidity, the important parameters of silk fibroin concentration and initial biomass were investigated. The effects of silk fibroin concentration on sialidase production and cell leakage are shown in . The highest sialidase yield was obtained when the hydrogel was prepared using 6% (w/v) silk fibroin. At higher concentration of silk fibroin, the hydrogel rigidity was improved but the sialidase yield decreased due to the diffusion limitation of the transfer of nutrients into the gel and sialidase out of the gel into the conversion buffer. On the other hand, the hydrogel prepared using lower concentration of silk fibroin was fragile, which resulted in the higher cell leakage. Therefore, the optimal concentration of silk fibroin was determined by the enzyme viability and diffusion.
The influences of initial biomass on the sialidase yield and cell leakage are shown in . Higher concentration initial biomass could produce higher sialidase yield, and however as the cell amount rose, the cell leakage increased correspondingly. The sialidase yield increased when the initial cell concentration ranged from 2 to 12 g wet weight. Previous reports had revealed that high cell densities resulted in higher product yield. Yet in the present study the decreased enzyme yield was triggered by the increase of initial biomass. The reason might be the competition between cells due to no enough nutrients available in conversion buffer for optimal growth.
Optimization of conversion conditions for GM1 production in batch operation
Previous studies have reported that sialidase has high activity from 24°C to 32°C and from pH 6.0 to 9.0. Also, the effect of temperature on sialidase production is more important than pH. So, the role of temperature on the GM1 production was investigated. As shown in , GM1 conversion ratio increased with temperature increasing. However, GM1 conversion was inhibited strongly up to 40°C implying that the cells were damaged. Maximum GM1 conversion ratio (305%) was at 35°C.
Figure 4. Effect of temperature on monosialotetrahexosylganglioside (GM1) conversion ratio. GM1 was produced by 5 kg of cell-immobilized gel in tandem packed-bed reactor at pH 7.5 and various feed flow rates.
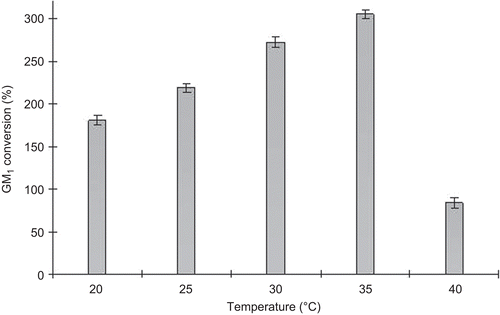
GM1 were continuously produced at optimal conversion conditions. shows the effect of the feed flow rate on the production of GM1. GM1 conversion ratio remarkably decreased when the feed flow rate increased to 6 mL/min. At a higher feed flow rate, gangliosides have no enough retention time in the reactor and not been completely converted to GM1. Although the maximum GM1 conversion ratio was actually obtained at the lowest feed flow rate of 1 mL/min, the optimal feed flow rate was 6 mL/min and determined in view of conversion efficiency.
Continuous production of GM1 in a packed-bed reactor
Continuous production of GM1 using immobilized cells was studied over a period of 30 days, which corresponded to 15 cycles of conversion. Every conversion cycle took about 40 h. The result of GM1 productive strength in conversion process is shown in . The immobilized cells could be reused effectively for GM1 conversion at higher productive strength over 3 g/L·h till the 15th day. During the residue period GM1 productive strength obviously decreased due to the loss of cell viability. Therefore, the cell-immobilized gel would maintain high sialidase activity and GM1 productive strength for about 15 days and 10 conversion cycles.
Figure 6. The continuous monosialotetrahexosylganglioside (GM1) productive strength in packed-bed reactor at the optimized conditions.
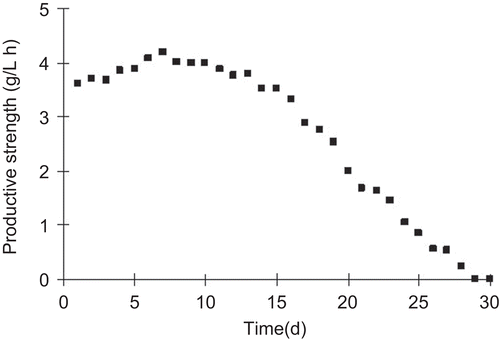
The process curve of GM1 conversion ratio shown in indicated GM1 conversion ratio sustained higher level during the former 15 days. The maximum GM1 conversion ratio was up to 313%. These data demonstrated that the GM1 conversion with long term of 15 days was successfully conducted in the packed-bed reactor. Under the feed rate of 6 mL/min of gangliosides, GM1 productive strength was maintained above 2 g/L·h for over 20 days. It decreased to zero at the 30th day. This cell-immobilized technique took obvious advantages when compared with the GM1 conversion using free cell. This continuous operation was found to have potential for industrial-scale GM1 production.
Declaration of interest
The support of the Executive Director Dr. Zhong Liu made it available for the authors to carry out the present research in the Biotechnology Center of Shandong, P. R. China.
References
- Argentino C, Sacchetti ML, Toni D, Savoini G, D’Arcangelo E, Erminio F, Federico F, Milone FF, Gallai V, Gambi D. (1989). GM1 ganglioside therapy in acute ischemic stroke. Italian Acute Stroke Study—hemodilution + drug. Stroke, 20, 1143–1149.
- Fukano Y, Ito M. (1997). Preparation of GM1 ganglioside with sialidase-producing marine bacteria as a microbial biocatalyst. Appl Environ Microbiol, 63, 1861–1865.
- Gandini C, Kitsos M, Massolini G, de Lorenzi E, Soldi A, Caccialanza G, Kirschner G. (1990). Determination of gangliosides in parenteral dosage form by high-performance liquid chromatography. J Pharm Biomed Anal, 8, 1063–1066.
- Geisler FH, Dorsey FC, Coleman WP. (1991). Recovery of motor function after spinal-cord injury—a randomized, placebo-controlled trial with GM-1 ganglioside. N Engl J Med, 324, 1829–1838.
- Kanfer JN. (1969). Preparation of gangliosides. Methods Enzymol, 14, 660–664.
- Kato T, Hatanaka K. (2008). Purification of gangliosides by liquid–liquid partition chromatography. J Lipid Res, 49, 2474–2478.
- Kim UJ, Park J, Li C, Jin HJ, Valluzzi R, Kaplan DL. (2004). Structure and properties of silk hydrogels. Biomacromolecules, 5, 786–792.
- Lin JH, Liu LY, Yang MH, Lee MH. (2004). Ethyl acetate/ethyl alcohol mixtures as an alternative to Folch reagent for extracting animal lipids. J Agric Food Chem, 52, 4984–4986.
- Matsumoto A, Chen J, Collette AL, Kim UJ, Altman GH, Cebe P, Kaplan DL. (2006). Mechanisms of silk fibroin sol–gel transitions. J Phys Chem B, 110, 21630–21638.
- Peng YF, Wang XD, Wei DZ. (2007). Development of a large scale process for the conversion of polysialogangliosides to monosialotetrahexosylganglioside with a novel strain of Brevibacterium casei producing sialidase. Biotechnol Lett, 29, 885–889.
- Schneider JS, Roeltgen DP, Rothblat DS, Chapas-Crilly J, Seraydarian L, Rao J. (1995). GM1 ganglioside treatment of Parkinson’s disease: An open pilot study of safety and efficacy. Neurology, 45, 1149–1154.
- Svennerholm L, Bråne G, Karlsson I, Lekman A, Ramström I, Wikkelsö C. (2002). Alzheimer disease—effect of continuous intracerebroventricular treatment with GM1 ganglioside and a systematic activation programme. Dement Geriatr Cogn Disord, 14, 128–136.
- Tatsuro Y, Haruki M, Masanobu A, Atsushi K. (1989). Preparation of monosialoganglioside. US Patent 4,868,292.
- Wang X, Kluge JA, Leisk GG, Kaplan DL. (2008). Sonication-induced gelation of silk fibroin for cell encapsulation. Biomaterials, 29, 1054–1064.