Abstract
Context: Archachatina marginata Swainson (Achatinidae) is found in Nigeria, West Africa. Its hemolymph is applied as a disinfectant to blades and fresh cuts of circumcision in Yorubaland. The hemolymph is also used in traditional medicine practice. Investigation into its anti-endotoxin response is being studied for the first time.
Objective: This study determined whether endotoxin causes measurable and concentration-dependent protein coagulation in the separate hemolymph fractions and in hemocyte lysate (HL)/plasma mixtures.
Materials and methods: Endotoxin was prepared by inoculating 5% w/v dextrose with locally isolated Escherichia coli cells and incubated for 48 h before sterilization. Pyrogenicity was determined by rabbit test method and use the of LAL kit. Hemolymph fractions were exposed to endotoxin while controls were exposed to endotoxin-free water (0.025 EU/ml). HL/plasma (1:1 v/v) was exposed to varied endotoxin concentrations.
Results: Data indicated significantly higher protein coagulates induced by endotoxin in all the hemolymph fractions (P < 0.05). Maximum protein coagulation in mixture of HL/plasma 1:1 was recorded. Exposure of HL/plasma at optimal ratio to varied endotoxin caused linear protein coagulation up to 1.0 EU/ml, beyond which it dropped significantly and unresponsive to further increase in endotoxin doses.
Discussion and conclusion: There was endotoxin-induced protein coagulation, which is endotoxin concentration-dependent. The optimal coagulation observed for 1:1 HL/plasma mixture suggests stronger interaction between the hemocytes and the plasma in response to endotoxin. There are LPS-binding proteins in the plasma and hemocytes of A. marginata. This finding may be employed in detection and quantification of endotoxin in future.
Introduction
Innate immunity in invertebrates is a nonclonal defense mechanism which depends upon the ability of the organisms to respond to pathogen-associated molecular patterns (PAMPs), through the use of pattern recognition receptors (PRRs) on their hemocyte cell membranes. The recognition of PAMPs leads to generation of responses leading to the destruction of the invading pathogen. Such responses lead to recognition of self and nonself antigens and help protect the organism against pathogens responses elicited sometimes include protein coagulation and/or melanization or synthesis of antimicrobial peptides. PAMPs are generally preserved patterns associated with microorganisms such as the peptidoglycan in all bacteria, lipid A of the lipopolysaccharides of Gram negative bacteria and β-1-3-d-glucans in fungi (CitationMedzhitov and Janeway, 1997).
The invertebrate protein coagulation systems are important in the defense of invertebrates against micro organisms. In crustaceans for example, processes of defense involve agglutination, encapsulation, phagocytosis, glycoproteins and clottable proteins. All are induced by carbohydrate-driven recognition patterns (CitationPereyra et al., 2009; CitationVazquez et al., 2009). The invertebrate coagulation systems are well studied in the Limulus crab (CitationTamura et al., 1996). The Limulus endotoxin-induced coagulation is the basis of limulus amebocyte lysate (LAL) test of bacterial endotoxin in medical preparations in use to date.
Archachatina marginata Swainson (Achatinidae) is a gastropod found in the forest and savannah areas of West Africa (CitationRaut and Barker, 2002). It is a good source of protein, eaten in soups and stew in Nigeria. A. marginata’s meat has 12–60% protein, 0.05–0.8% fat and 45–50 mg/kg iron (CitationCobbinah, 1997). Its hemolymph is made up of 5.6 g/dl proteins. Triglycerides and cholesterol have been detected in the hemolymph. Alkaline phosphatase (ALP), alanine aminotransferase (ALT) and aspartate transaminase are some enzymes with activity found in the snail’s hemolymph. Na+, K+, Ca2+, Mg2+, Zn2+ and bicarbonate ions are some of the minerals found in the hemolymph of both the wild and captive snails. The hemolymph biochemical parameters have been reported to contain, albumin/globulin ratios similar to those of some mammals like the pangolin and sheep (CitationOgunsanmi et al., 2003).
The snail’s hemolymph is used in folk medicine. From orally given information, it is used in the traditional management of hypertension. The fresh hemolymph is swallowed in tablespoonfuls by the hypertensives. During circumcision, fresh hemolymph obtained by apical cracking of the shells of the snails is applied to fresh cut of the foreskin. This was believed to aid quick healing and prevent infection of the wound. However, no scientific evidence has been shown to support this practice.
There is dearth of information about the coagulation system in molluscs. This is the first report of the presence of LPS-binding protein in A. marginata. A study of the mollusc’s hemolymph coagulation responses to Escherichia coli endotoxin may provide information on whether its hemolymph may serve as an alternative test material for endotoxin. This study was designed to examine the presence of endotoxin-induced protein coagulation reactions in A. marginata hemolymph fractions and the endotoxin concentration dependence of the reaction.
Methods
Snails collection and identification
Twenty A. marginata snails were purchased from Ipata Market. Ilorin, Kwara State, Nigeria, weighing 435 ± 35 g each with shell lengths of 165 ± 10 mm and largest whorl circumference of 145 ± 15 mm. A sample of the snails was submitted to and identified by Mr. Bolarinwa on October 3, 2008 and issued a collection number in the Department of Zoology, University of Ilorin. The sample was deposited with the department as reference sample. Dextrose monohydrate powder and E. coli laboratory isolates were obtained from the Quality Control/Assurance Department of Biomedical Limited, Ilorin, Nigeria. All chemicals used were of analytical grade and obtained from the chemical store of the Department of Biochemistry, University of Ilorin, Ilorin, Nigeria. The use of animals was approved by the Ethics Committee of the Department of Biochemistry, University of Ilorin, Ilorin, Nigeria.
Preparation of endotoxin from E. coli
E. coli endotoxin stock was prepared as reported earlier (CitationSalawu et al., 2010). Briefly, 5% weight per volume (w/v) dextrose solution was prepared using sterilized endotoxin-free distilled water and dextrose monohydrate powder. The solution was inoculated with a strain of laboratory isolate of E. coli and incubated at 37°C for 48 h and then autoclaved for 35 min. A 5% w/v dextrose control solution was prepared, but sterilized immediately. The microbial load of both solutions was determined by using the pour plate methods. The pyrogen (endotoxin) content was determined using the standard rabbit test method as described in the (CitationRadhakrishna, 2010). The dextrose solution inoculated with E. coli and sterilized by autoclaving after 48 h and tested for pyrogen. In anticipation of high endotoxin content, the solution was diluted with pyrogen-free 0.9% w/v sodium chloride (1:9). The control dextrose solution that was sterilized immediately was also tested for pyrogen using the rabbit test method (Precautions taken: The animals used for the tests have not failed a product in the previous 2 weeks. Animals used maintained their initial body temperatures to within 0.2°C within the 60 min before the injection of the dextose solutions, three animals per sample were used weighing not less than 1.5 kg each).
Endotoxin handling
Pyrogenic stock solution was handled carefully with sterile surgical gloves. All other reagents used were common laboratory analytical reagents. The Material Safety Data sheets of the common reagents used are available from literature. None of the material/chemicals used required additional information in terms of its toxicity/pathological side effects.
Extraction of hemolymph
The snails were washed in distilled water to remove dirt from their shells and foot by gently rubbing with hands. The hemolymphs were obtained by apical cracking method (CitationOgunsanmi et al., 2003). The fresh hemolymphs obtained per animal was between 20 and 25 ml in volume and pooled together. Equal volumes of 10 mM EDTA in 10 mM phosphate buffer pH 7.0 containing 0.142 M NaCl and 0.18 M glucose were added and centrifuged at room temperature, 800g for 10 min (CitationKopacek et al., 1993). The supernatant was collected as A. marginata plasma. The pellets were washed in 10 mM phosphate buffer pH 7.0 containing 0.1 M CaCl2 and centrifuged at 800g for 10 min. The supernatants were discarded and the pellets harvested as hemocytes and then homogenized in a 10-mM phosphate buffer pH 7.0 containing 10 mM EDTA. The homogenate was suspended in the same buffer and adjusted to the initial actual volume of the hemolymph that was processed. The homogenate was the hemocyte lysate (HL). HL (10 ml) was centrifuged at 800g for 20 min and the supernatant collected as HL supernatant (HLS). The pellet cytosol free hemocyte lysate debris (HLD) contained the hemocyte membranes were washed twice in 1 ml 10-mM phosphate buffer pH 7.0 and suspended in the same buffer made up to the initial hemolymph volume that yielded the HLD. The fractions were stored frozen.
Coagulation reactions in the HL, HLS, HLD and plasma
Borosilicate glass tubes and pipettes previously depyrogenated by dry heating in an oven at 250°C for 30 min (CitationRadhakrishna, 2010) were used for this experiment. 0.1 EU in 0.1 ml distilled water was added to 0.8 ml of HL and 0.1 ml of 0.1 M CaCl2. Same procedure was repeated using HLS, HLD and plasma, respectively. Four replicates were prepared for each hemolymph fraction. A control was also prepared in quadruplicates for each fraction using endotoxin-free water (≤0.025 EU/ml). The reaction mixtures were incubated at 37°C for 1 h. Coagulants were observed in each tube and then fixed to the bottom of the tubes by centrifugation (CitationVazquez et al., 2009) while supernatants were discarded. The pellets were then dissolved in 0.9% w/v sodium chloride solution.
Determination of protein content in the coagulants obtained from HL, HLS, HLD, plasma and the controls
The total protein contents of each fraction and its control were determined using biuret reaction (CitationGornall et al., 1949). The characterization of the protein coagulates was intended for future studies.
Determination of the optimum HL:plasma ratio required for endotoxin-induced coagulation in A. marginata hemolymph fractions
HL and plasma were mixed in various ratios and exposed to a final endotoxin concentration of 0.1 endotoxin unit per milliliter (EU/ml). The total volume of reaction mixture was 1.0 ml containing 0.1 ml 0.1 M CaCl2. A control was prepared for each reaction mixture prepared in quadruplicates. The samples were then incubated at 37°C for 1 h centrifuged at 800g to fix the coagulants to bottom of test tubes. The supernatants were discarded and the coagulants dissolved in 1 ml 0.9% w/v NaCl and the protein content estimated by biuret reaction method (CitationGornall et al., 1949).
Determination of concentration dependence of endotoxin-induced protein coagulation using HL:plasma mixture
After the determination of the optimal ratio of HL:plasma, the ratio that gave maximum protein coagulation was utilized to study the concentration dependence of the protein coagulation in A. marginata hemolymph. A reaction mixture containing equal volume of HL and plasma, 0.1 ml CaCl2 and 0.1 ml of endotoxin was used (endotoxin concentrations of 0.0, 0.125, 0.25, 0.50, 0.75, 1.0, 1.5, 2.0, 2.5, 3.0, 3.5, 4.0, 4.5, and 5.0 EU/ml). A blank was prepared containing endotoxin-free water (endotoxin concentration ≤0.025 EU/ml). Reaction mixtures were prepared in quadruplicates for 1 h, centrifuged at 800g and supernatants discarded. The coagulants were dissolved in 0.9% w/v NaCl and protein content estimated by biuret reaction (CitationGornall et al., 1949). Values were corrected with blanks prepared with endotoxin-free water (endotoxin concentration ≤0.025 EU/ml) incubated with each HL:plasma mixture.
Results
The protein contents of the coagulants determined as A 550 nm are shown in . The protein contents obtained with the presence of 1.0 EU/ml in each hemolymph fraction was significantly higher than those of the controls (P < 0.05). The introduction of endotoxin caused higher quantities of protein to coagulate from the hemolymph fractions. There was maximum protein coagulation at HL:plasma ratio of 1:1. Therefore, maximum anti-endotoxin response in terms of protein coagulation was obtained as an additive increase between the HL and the plasma (). There was progressive increase in protein coagulation from endotoxin contents of 0–0.1 EU per reaction mixture corresponding to concentrations of 0–1.0 EU/ml solution of endotoxin incubated with the HL:plasma mixture. At higher concentrations, there was a drop in coagulation and a loss of concentration-dependent response ().
Figure 1. Endotoxin-induced protein coagulation in Archachatina marginata hemolymph fractions. Values are Mean ± SEM of 4 determinations. Different superscripts on the test and control for each hemolymph fraction indicate significantly different values at p <0.05.
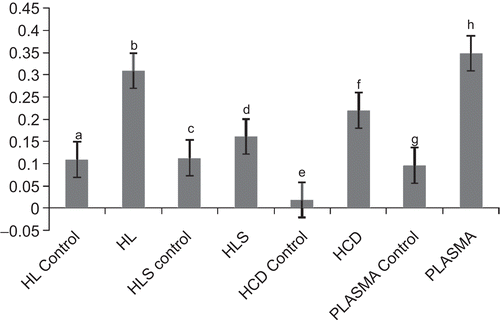
Discussion
Protein coagulation systems in invertebrates serve as an important process for limiting infection by pathogenic organisms. In crustaceans (crabs and crayfish), coagulogen and coagulating enzymes (transglutaminases), which exist normally as zymogens, are involved in a cascade of reactions leading to protein coagulation, in order to protect the integrity of the organisms (CitationKawabata et al., 1996; CitationVazquez et al., 2009). The prophenoloxidase cascade also leads to melanization in protein meshwork in insects and other invertebrates at the sites of injury. It was associated with cuticle formation in crustaceans such as Pacifastacus leniusculus Dana (Astacidae) (CitationKopacek et al., 1993). The presence of opsonin-related proteins was discovered in the freshwater crayfish (CitationLee and Söderhäll, 2001) which helps in clearance of E. coli from the crayfish hemolymph in vivo.
In this study, A. marginata hemolymph fractions: the HL, HLS, HLD and the plasma were exposed to endotoxin and distilled water (endotoxin content ≤0.025 EU/ml), respectively. The fractions after incubation produced protein coagulates that were significantly different in quantity (P < 0.05). The fractions exposed to endotoxin actually produced higher quantity of endotoxin than those exposed to distilled water (≤0.025 EU/ml). A combination of various ratios of HL/plasma was also exposed to equal quantity of endotoxin. The 1:1 HL/plasma mixture produced optimal quantity of endotoxin. The exposure of optimal HL:plasma mixture to endotoxin ranging from 0 EU/ml to 5.0 EU/ml produced a linearly increased coagulation up to endotoxin concentration of 1.0 EU/ml, beyond which there was a drop in response.
The data generated suggest that LPS-binding proteins were present in the hemolymph fractions of the snail—the plasma, HLS, HLD (). The protein coagulation induced by endotoxin is a joint response by the LPS-binding proteins in the plasma as well as those produced by the hemocytes as shown in the response in the HL. A synergistic response was produced by the HL and the plasma when mixed in equal ratio (). There was a progressive increase in protein coagulation in a mixture of HL/plasma until equal volume mixture of the fractions was attained (). This suggests that there is a cellular response in terms of protein degranulation as well as LPS-binding of some plasma proteins simultaneously in response to the presence of endotoxin in the hemolymph. This binding and coagulation may serve a purpose in encapsulating and restricting an invading Gram negative pathogen, prior to hemocyte engulfing of the bacteria and endocytosis. Exposure of HL/plasma mixture at optimal ratio to varying concentrations of endotoxin causes quantitative response in terms of protein coagulation to E. coli endotoxin (). There was progressive increase in the quantity of protein coagulates as endotoxin concentration increased from 0 to 1.0 EU/ml. At higher concentrations, there was a drop in the quantities of protein coagulated and then no further significant change with increasing concentration of endotoxin.
The coagulated proteins are likely to be lectins. Such proteins were discovered in Achatina fulica; achatinin-H (CitationSen and Mandal, 1995) and achatinin (CitationBiswas, 2000). In A. fulica, a related gastropod to A. marginata, achatinin-H was isolated and characterized (CitationSen and Mandal, 1995), achatinin was also isolated from the hemolymph of the same gastropod and characterized (CitationBiswas, 2000). The two proteins were lectins. On SDS-PAGE electrophoresis, achatinin showed 16 subunits each 15 kdal. Achatinin-H was shown to be homologous to that of achatinin. Both lectins were shown to be of importance in the innate immunity of A. fulica as they respectively bind (E. coli O55: B5) lipopolysaccharide’s 9-O-acetyl sialic acid and coagulate out of solution. This reaction explains why E. coli cells are agglutinated by these lectins in A. fulica.
Further studies on the endotoxin-induced protein coagulates from A. marginata hemolymph fractions may provide information on the constituent proteins and their structure.
Conclusions
From this study, we conclude that lipopolysaccharide-binding proteins likely exist in the heamolymphs of A. marginata. There is a quantitative response to exposure to endotoxin at concentration range between 0 and 1.0 EU/ml by hemolymph fractions of A. marginata. This can be employed in the future for detection and quantification of bacterial endotoxin in medications meant for parenteral use.
Acknowledgement
We acknowledge the contributions of the Management of Biomedical Limited, Ilorin, Nigeria; Mrs. A. Amupitan and Miss Arinola Busari for the microbiological aspect of this work.
Declarations of interest
The authors report no declarations of interest. The authors alone are responsible for the content and writing of the article.
References
- Biswas SM. (2000). Investigation on the interaction of achatinin a 9-O-acetyl sialic acid binding lectin with lipopolysaccharide in the innate immunity of Achatina fulica snails. Mol Immunol, 37, 745–754.
- Cobbinah JR. (1997). Snail Farming in West Africa: A Practical Guide. Technical Centre for Agricultural and Rural Cooperation (CTA) Wageningen, Netherland pp. 1–16.
- Gornall AG, Bardawill CJ, David MM. (1949). Determination of serum protein by means of Biuret reaction. J Biol Chem, 177, 751–756.
- Kawabata S, Tokunaga F, Kugi Y, Motoyama S, Miura Y, Hirata M, Iwanaga S. (1996). Limulus factor D, a 43-kDa protein isolated from horseshoe crab hemocytes, is a serine protease homologue with antimicrobial activity. FEBS Lett, 398, 146–150.
- Kopacek P, Hall M, Söderhäll K. (1993). Characterization of clotting protein, isolated from plasma of the freshwater crayfish Pacifastacus leniuscus. Eur Biochem, 213, 591–597.
- Lee SY, Söderhäll K. (2001). Characterization of a pattern recognition protein, a masquerade-like protein, in the freshwater crayfish Pacifastacus leniusculus. J Immunol, 166, 7319–7326.
- Medzhitov R, Janeway CA Jr. (1997). Innate immunity: the virtues of a nonclonal system of recognition. Cell, 91, 295–298.
- Ogunsanmi AO, Taiwo VO, Akintomide TO. (2003). Haemolymph biochemical parameters of the African giant snail (Achatina achatina) and the big black snail (Achachatina marginata). Trop Vet, 21, 43–48.
- Pereyra A, Agundis C, Barrera B, Alpuche J, Sierra C, Zenteno R, Zenteno E, Vazquez L. (2009). The use of monoclonal antibodies anti-lectin from freshwater prawn Macrobrachium rosenbergii (DeMan, 1879) in the recognition of protein with lectin activity in Decapod’s hemolymph. Prep Biochem Biotechnol, 39, 308–322.
- Radhakrishna ST. (2010). Rabbit Pyrogen test; United States Pharmacopoeia XXIX, USP 29-NF24 p. 2546; available online at http://www.pharmacopeia.cn/v29240/usp29nf24s0_c151.html searched on 13th September, 2010.
- Raut SK, Barker GM. (2002). Achatina fulica Bodwich and other Achatinidae as pests in tropical agriculture In: Molluscs as Crop Pests. CAB International.
- Salawu MO, Oloyede OB, Oladiji AT, Yakubu MT, Atata RF. (2010). Effect of delayed sterilization on the production of intravenuous infusion (parenterals). Afr J Biotech 9, 6948–6951.
- Sen G, Mandal C. (1995). The specificity of the binding site of achatinin-H, a sialic-acid binding lectin from Achatina fulica. Carb Res, 268, 115–125.
- Tamura H, Tanaka S, Oda T, Uemura Y, Aketagawa J, Hashimoto Y. (1996). Purification and characterization of a (1-3)-β-d-glucan-binding protein from horseshoe crab (Tachypleus tridentatus) amoebocytes. Carb Res, 295, 103–111.
- Vazquez L, Alpuche J, Maldonado G, Agundis C, Pereyra-Morales A, Zenteno E. (2009). Review: Immunity mechanisms in crustaceans. Innate Immun, 15, 179–188.