Abstract
Context: Emblica officinalis (Euphorbiaceae), commonly known as amla, is traditionally used for central nervous system (CNS) disorders.
Objective: In the present study, the effect of standardized hydroalcoholic extract of E. officinalis fruit (HAEEO), an Indian medicinal plant with potent antioxidant activity, was studied against kainic acid (KA)-induced seizures, cognitive deficits and on markers of oxidative stress.
Materials and methods: Rats were administered KA (10 mg/kg, i.p.) and observed for behavioral changes, incidence, and latency of convulsions over 4 h. The rats were thereafter sacrificed for estimation of oxidative stress parameters: thiobarbituric acid-reactive substances (TBARS) and glutathione (GSH). The proinflammatory cytokine tumor necrosis factor alpha (TNF-α) was also determined in the rat brain.
Results: Pretreatment with HAEEO (500 and 700 mg/kg, i.p.) significantly (P < 0.001) increased the latency of seizures as compared with the vehicle-treated KA group. HAEEO significantly prevented the increase in TBARS levels and ameliorated the fall in GSH. Furthermore, HAEEO dose-dependently attenuated the KA-induced increase in the TNF-α level in the brain. HAEEO also significantly improved the cognitive deficit induced by KA, as evidenced by increased latency in passive avoidance task.
Discussion and conclusion: HAEEO at the dose of 700 mg/kg, i.p., was most effective in suppressing KA-induced seizures, cognitive decline, and oxidative stress in the brain. These neuroprotective effects may be due to the antioxidant and anti-inflammatory effects of HAEEO.
Keywords::
Introduction
Epilepsy, one of the most common neurological disorders, shows major clinical syndromes such as spontaneous recurrent seizures (SRS). In adults with temporal lobe epilepsy (TLE), complex partial seizures have the poorest prognosis of all types of seizures, with about 60–70% of all patients having intractable seizures (CitationBertram, 2009). In addition, memory problems are more common in human TLE (CitationCarreño et al., 2008). The underlying mechanism is however not clear. Kainic acid (KA) is a structural analog of glutamate, an excitatory amino acid neurotransmitter (CitationSchmidt et al., 1976). A single systemic injection of a convulsive dose of KA results in limbic status epilepticus (SE), which is followed by long-term SRS, as well as spatial learning impairments (CitationMohamad et al., 2001). KA-induced epilepsy model has been used widely to study human TLE (CitationSchwarzer & Sperk, 1995). It has been proposed that activation of excitatory amino acid receptor can trigger the formation of reactive oxygen species (ROS) (CitationGupta et al., 2009). These increased ROS in turn may further release glutamate thus forming a loop. This “vicious” cycle not only causes a long-lasting seizure formation, but if not arrested may also lead to the neuronal death (CitationSaid et al., 2000; CitationAtlante et al., 2001). SE is an emergency condition where seizures last for a long time and if not controlled neuronal injury occurs (CitationCherlyee & Thomas, 1995; CitationChen et al., 2010). KA-induced SE following systemic or intracerebroventricular injection of the drug initiates neuronal injury and death in different parts of the limbic systems (CitationMontgomery et al., 1999). The mechanism involved in the pathogenesis appears to be linked to oxidative stress (CitationZaja-Milatovic et al., 2008). In various experimental studies, it has been demonstrated that antioxidants can prevent the excitotoxicity induced by agents such as glutamate and KA (CitationSilva et al., 2008; CitationDong et al., 2009; CitationWu et al., 2009).
Thus, antioxidants may have a potential role in preventing excitotoxicity-induced seizure genesis. In this respect, scavenging of free radicals by nonenzymatic/exogenous antioxidants seems to be the most practical approach. The anticonvulsant effect of several agents having antioxidant property such as curcumin, vineatrol, transresveratrol, melatonin, adenosine, and α-lipoic acid has been demonstrated in our laboratory (CitationGupta & Briyal, 2006).
Recent findings in experimental models and in the clinical setting suggest the possible role of inflammatory processes in the etiopathogenesis of seizures (CitationVezzani & Granata, 2005). Proinflammatory cytokines such as interleukin (IL)-1β, tumor necrosis factor alpha (TNF-α), and IL-6 have been shown to be overexpressed in experimental models of seizures in brain areas of seizure generation and propagation (CitationRao et al., 2009). Therefore, possible strategies that involve the pharmacological manipulation of inflammation can lead to the development of novel approaches for the treatment of epilepsy. Emblica officinalis (Euphorbiaceae) is commonly known as amla. It has been used in traditional system of medicine for the treatment of diseases of nervous system, epilepsy, for enhancement of memory and intelligence, hemiplegia, fever, and headache (CitationKirtikar & Basu, 1996). The fruits are also of culinary use and contain thermostable vitamin C, minerals, and amino acids. It has high antioxidant activity on account of tannoids, tannins, vitamin C, and flavonoids (CitationPozharitskaya et al., 2007). The pharmacological studies on fruit of E. officinalis has revealed that it has good antioxidant, cytoprotective and immunomodulatory, cardioprotective, antiulcerogenic, antimutagenic, and hepatoprotective activity (CitationKhan & Khan, 2009; CitationSharma et al., 2009; CitationKim et al., 2010). Different clinical studies have also reported that E. officinalis has hypolipidemic, antibacterial, and antidiabetic activity (CitationJacob et al., 1988; CitationBiswas et al., 2001; CitationChandra et al., 2004).
Since E. officinalis has very high antioxidant activity and the primary underlying pathology of SE is attributable to increased oxidative stress, it was considered worthwhile to evaluate the anticonvulsant effect of hydroalcoholic extract of E. officinalis (HAEEO) in the KA model of epilepsy. Thus, the aim of this investigation was to investigate the effect of HAEEO in KA-induced seizures, oxidative stress, and cognitive impairment in rats. Furthermore, we have also evaluated the effect of HAEEO on TNF-α, a proinflammatory cytokine.
Materials and methods
Animals
This study was carried out using male Wistar rats weighing 150–200 g. They were obtained from the central animal house facility of All India Institute of Medical Sciences, New Delhi and stock bred in the Departmental animal house. The rats were group-housed in polyacrylic cages (38 × 23 × 10 cm) with not more than four animals per cage and were maintained under standard laboratory conditions with natural dark and light cycles (~14 h light–10 h dark cycle) and a room temperature of 25 ± 1°C. They were allowed free access to standard dry diet (Golden Feeds, New Delhi, Delhi, India) and tap water ad libitum. All the behavioral procedures were carried out between 0900 and 1300 h. All procedures described were reviewed and approved by the Institutional Committee for Ethical Use of Animals and care of animals was taken as per guidelines of CPCSEA, Ministry of Forests and Environment, Government of India.
Plant extract and phytochemical analysis
The standardized lyophilized HAEEO was procured from Sanat Products Pvt. Limited, New Delhi, India (A WHO-GMP and ISO 9001 Accredited Herbal Extract Company). The identity of E. officinalis was authenticated by Dr. Santosh Kumari, Division of Plant Physiology, Indian Agriculture Research Institute, New Delhi, India. The voucher specimen of lyophilized extract of the fruits of E. officinalis (No. EO 0114) was deposited at Department of Pharmacology, All India Institute of Medical Sciences, New Delhi, India. The phytochemical analysis was done by using HPLC (Waters, Milford, MA). The extract obtained was of the highest purity with 28.26% w/w of hydrolyzable tannins emblicanin A and emblicanin B on dried weight basis.
Drugs and chemicals
KA, radioimmunoprecipitation assay (RIPA) lysis buffer, and dimethyl sulfoxide (DMSO) were purchased from Sigma Chemical Co. (St. Louis, MO). The protease inhibitors cocktail was purchased from Roche Applied Science (Mannheim, Germany). Enzyme-linked immunosorbent assay (ELISA) kits were from Pierce Biotechnology, Inc. (Rockford, IL). KA was dissolved in distilled water. HAEEO was prepared freshly by dissolving in normal saline. All other materials were of the highest grade available.
KA-induced SE
Experimental seizure model
Rats were administered KA at a dose 10 mg/kg intraperitoneally (i.p.). The pH of KA solution was adjusted to 7.2 ± 0.1. Following administration of KA, animals were observed for behavioral changes (grooming, rearing, hind limb scratching, urination, defecation, wet dog shakes, jaw movements, salivation, head nodding), incidence and latency of convulsions and mortality over the total period of 4 h (CitationGupta & Briyal, 2006).
Experimental design
Rats were divided into five groups and each group consisted of a minimum of six animals. Separate animals were used for each experiment.
Group I: It represented the control group. The rats were injected with vehicle normal saline i.p. for 7 days (this group was used in biochemical and behavioral studies).
Group II: It represented the vehicle-treated KA group. Vehicle was administered for seven consecutive days. KA (10 mg/kg, i.p.) was administered on the seventh day 30 min after the administration of vehicle and animals were observed over a period of 4 h for the change in behavioral parameters, incidence and latency of convulsions.
Groups III, IV, and V: HAEEO (300, 500, and 700 mg/kg) was administered i.p. for 7 days. After 30 min of administration of last dose of HAEEO on the seventh day, KA (10 mg/kg, i.p.) was administered and rats were observed over a period of 4 h for the change in behavioral parameters, incidence and latency of convulsions.
In groups I–V before KA administration, initial latency (IL) was noted and after 24 h, that is, on the ninth day retention latency (RL) was noted using one trial passive avoidance task. This was done to assess the effect of KA on learning and memory. The rats were thereafter sacrificed for estimation of markers of oxidative stress (thiobarbituric acid-reactive substances [TBARS] and glutathione [GSH]) and determination of brain levels of TNF-α.
Behavioral tests
Single-trial passive avoidance test
Memory retention deficit was evaluated by step through passive avoidance apparatus. The apparatus consist of equal size light and dark compartments (30 × 20 × 30 cm). A 40-W lamp was fixed 30 cm above its floor in the center of the light compartment. The floor consisted of metal grid connected to a shock scrambler. The two compartments were separated by a trap door that could be raised to 10 cm. Before the administration of KA, rats were placed in the light compartment and the time lapse before each animal entered the dark compartment and had all four paws inside it was measured in seconds and termed as IL. Immediately after the rat entered the dark chamber with all the four paws inside the dark chamber, the trap door was closed and an electric foot shock (50 V a.c.) was delivered for 3 sec. Five seconds later, the rat was removed from the dark chamber and returned to its home cage. Twenty-four hours after the IL, the latency time was again measured in the same way as in the acquisition trial and was termed as the RL. However, during the retention trial, no foot shock was delivered, and the latency time was recorded to a maximum of 600 sec. To improve the reliability and validity of the foot shock avoidance test, the grid as well as the rat paw was moistened with water before foot shock as this is known to reduce the wide inter animal variability in paw skin resistance of the rats (CitationStubley-Weatherly et al., 1996).
Biochemical tests
Tissue preparation
Brain tissue samples were thawed and homogenized with 10 times (w/v) ice-cold 0.1 M phosphate buffer (pH 7.4). Aliquots of homogenates from rat brain were used to determine lipid peroxidation and GSH.
Measurement of lipid peroxidation
TBARS, a measure of lipid peroxidation, were measured as described by CitationOhkawa et al. (1979). The reagents 1.5 mL acetic acid (20%), pH 3.5, 1.5 mL thiobarbituric acid (0.8%), and 0.2 mL sodium dodecyl sulfate (SDS; 8.1%) were added to 0.1 mL of processed tissue samples, and then heated at 100°C for 60 min. The mixture was cooled with tap water and 5 mL of n-butanol/pyridine (15:1), and 1 mL of distilled water was added. The mixture was vortexed vigorously. After centrifugation at 4000 rpm for 10 min, the organic layer was separated and absorbance was measured at 532 nm using a spectrophotometer. The concentration of TBARS was expressed as nmol/g tissue.
Measurement of reduced GSH
GSH was measured according to the method of CitationEllman (1959). The equal quantity of homogenate was mixed with 10% trichloroacetic acid and centrifuged to separate the proteins. To 0.01 mL of this supernatant, 2 mL of phosphate buffer (pH 8.4), 0.5 mL of 5,5′-dithiobis(2-nitrobenzoic acid), and 0.4 mL of double distilled water were added. The mixture was vortexed and the absorbance read at 412 nm within 15 min. The concentration of reduced GSH was expressed as µg/g tissue.
TNF-α assay in rat brain by ELISA
For the ELISA of TNF-α, the brain was homogenized in 1 mL of ice-cold lysis buffer (RIPA) containing 50 mM Tris–HCl (pH 8.0), 150 mM sodium chloride, 1.0% Iepal CA-630 (NP-40), 0.5% sodium deoxycholate, 0.1% SDS, 1% phosphatase inhibitor cocktail, and a protease inhibitor cocktail. The lysate was centrifuged (15,000 g, 4°C) for 15 min, and the supernatant was added to 96-well ELISA plates. The TNF-α concentration was then determined by reading the ELISA plate.
Statistical analysis of data
Data obtained from experiments were expressed as mean ± SEM. Statistical differences between the treatment and the control groups were evaluated by one-way ANOVA followed by Tukey-Kramer post test. The P-value <0.05 was considered to be significant.
Results
Effect of KA on behavioral symptoms and convulsions
KA, when administered in vehicle-treated group at the dose of 10 mg/kg, i.p., exhibited behavioral signs, that is, grooming, rearing, hind limb scratching, urination, defecation, wet dog shakes, jaw movements, salivation, head nodding in all the rats within 5 min. All of the rats exhibited convulsions with the mean latency of 42.54 ± 3.64 min.
Effect of HAEEO on KA-induced SE
HAEEO was administered at doses of 300, 500, and 700 mg/kg, i.p., to the different groups. All the animals exhibited behavioral signs and seizures but there was an increase in the latency of occurrence of behavioral signs and seizures at each dose tested. Latency of occurrence of behavioral signs significantly increased to 13.43 ± 1.57 (P < 0.01), 23.32 ± 1.78, and 27.50 ± 1.69 min (P < 0.001) as compared with about 5 min in KA per se group and latency of convulsion was also significantly increased to 64.01 ± 2.64, 79.25 ± 2.60, and 99.85 ± 2.64 min, respectively, with 300, 500, and 700 mg/kg dose of HAEEO as compared with that in vehicle-treated KA group (42.54 ± 3.64 min) (P < 0.001) ().
Figure 1. Effect of 7 days pretreatment with HAEEO on KA-induced seizures in rats. Each value represents the mean ± SEM. for six rats. aP < 0.001 compared with vehicle-treated KA (ANOVA followed by Tukey-Kramer post test). KA represents kainic acid and HAEEO represents hydroalcoholic extract of Emblica officinalis.
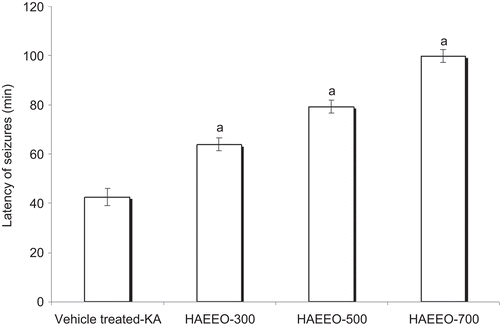
Effect of HAEEO on the levels of TBARS in KA-induced SE in rats
The brain levels of TBARS were significantly raised after KA administration (361.29 ± 10.79 nmol/g wet tissue) as compared with the control group rats (184.86 ± 3.99 nmol/g wet tissue) (P < 0.001). In the rats treated with HAEEO (300, 500, and 700 mg/kg, i.p.), the values of TBARS were 262.54 ± 5.74, 220.42 ± 5.16, and 196.56 ± 3.83 nmol/g wet tissue, respectively. The HAEEO dose-dependently and significantly decreased the levels of TBARS as compared with the vehicle-treated KA group by virtue of its potent antioxidant activity ().
Figure 2. Effect of 7 day pretreatment with HAEEO on levels of TBARS in KA-induced seizures in rats. Each value represents the mean ± SEM for six rats. aP < 0.001 compared with control, bP < 0.001, cP < 0.01 compared with vehicle-treated KA (ANOVA followed by Tukey-Kramer post test). KA represents kainic acid and HAEEO represents hydroalcoholic extract of Emblica officinalis.
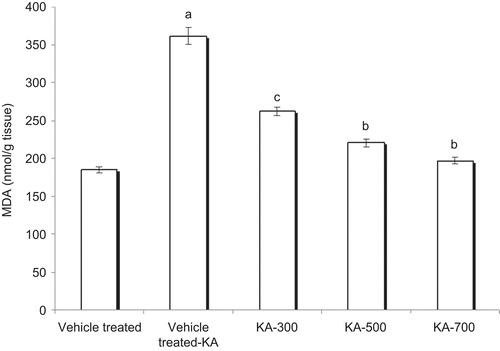
Effect of HAEEO on the levels of GSH in KA-induced SE in rats
The brain GSH levels were estimated in control, vehicle-treated KA rats and HAEEO-treated rats. The brain levels of GSH showed significant (P < 0.001) decrease in vehicle-treated KA group (50.0 ± 2.54 μg/g wet tissues) as compared with the control group rats (100.83 ± 4.10 μg/g wet tissues). In the rats treated with HAEEO (300, 500, and 700 mg/kg, i.p.), the values of GSH were 60.00 ± 2.37, 71.66 ± 2.10, and 87.70 ± 1.42 μg/g wet tissue, respectively. The values were significantly (P < 0.001) higher at the dose of 500 and 700 mg/kg, i.p., than the vehicle-treated KA group ().
Figure 3. Effect of 7 day pretreatment with HAEEO on levels of glutathione (GSH) in KA-induced seizures in rats. Each value represents the mean ± SEM for six rats. aP < 0.001 compared with control, bP < 0.001, cP < 0.01 compared with vehicle-treated KA (ANOVA followed by Tukey-Kramer post test). KA represents kainic acid and HAEEO represents hydroalcoholic extract of Emblica officinalis.
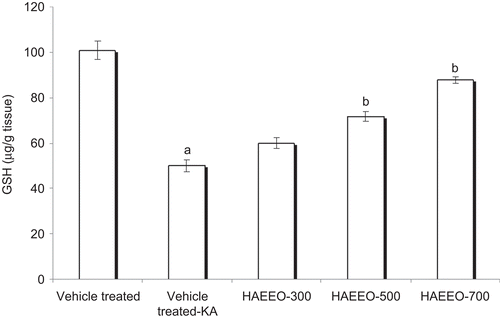
Effect of HAEEO on the levels of TNF-α in KA-induced SE in rats
The brain levels of TNF-α were significantly raised after KA administration (794.16 ± 25.98 pg/mL) as compared with the control group rats (476.29 ± 21.53 pg/mL) (P < 0.001). HAEEO dose-dependently attenuated the raised brain levels of TNF-α induced by KA (). The maximum inhibition was observed at the dose of 700 mg/kg of HAEEO (P < 0.001).
Figure 4. Effect of 7 day pretreatment with HAEEO on levels of TNF-α in KA-induced seizures in rats. Each value represents the mean ± SEM for six rats. aP < 0.001 compared with control, bP < 0.001, cP < 0.01 compared with vehicle-treated KA (ANOVA followed by Tukey-Kramer post test). KA represents kainic acid and HAEEO represents hydroalcoholic extract of Emblica officinalis.
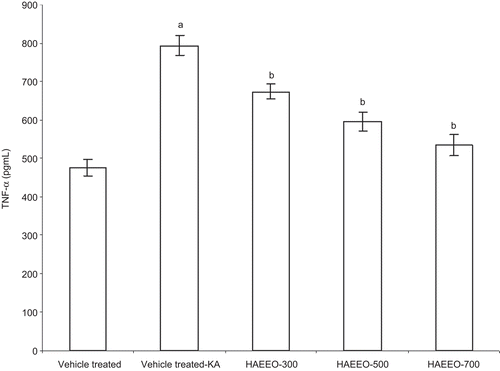
Effect of HAEEO on cognitive impairment induced by KA-induced SE in rats
RL, 48 h after the administration of KA in the vehicle-treated KA group, was significantly less (P < 0.001) compared with the control group rats, which indicates significant cognitive impairment due to KA. The HAEEO (300, 500, and 700 mg/kg, i.p.) + KA-treated groups showed significant reversal of KA-induced cognitive deficit, evidenced by dose-dependent increase in retention latencies (P < 0.05 with 300; P < 0.001 with 500 and 700 mg/kg, i.p.). The mean retention latencies of control, vehicle-treated KA group, and HAEEO (300, 500, and 700 mg/kg, i.p.) + KA groups were 278.35 ± 11.16, 110.2 ± 4.26, 128.6 ± 2.93, 180.7 ± 3.10, and 230.4 ± 4.18 sec respectively ().
Figure 5. Effect of 7 day pretreatment with HAEEO on KA-induced cognitive impairment in rats. Each value represents the mean ± SEM for six rats. aP < 0.001 compared with control, bP < 0.001, cP < 0.01, dP < 0.05 compared with vehicle-treated KA (ANOVA followed by Tukey-Kramer post test). KA represents kainic acid and HAEEO represents hydroalcoholic extract of Emblica officinalis.
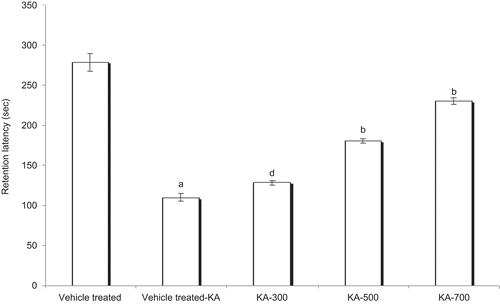
Discussion
Epilepsy is the commonest neurological disorder encountered in clinical practice. Approximately 2 million persons in the United States have epilepsy, and 3% of persons in the general population will have epilepsy at some point in their lives (CitationChang & Lowenstein, 2003). In recent years, important advances have been made in the diagnosis and treatment of seizure disorders. The exact mechanism for the genesis of seizures is not clear. However, it has been proposed that there may be overexcitation of excitatory amino acid receptor and/or inhibition of GABAergic system (CitationKardos, 1999; CitationSierra-Paredes & Sierra-Marcuño, 2007). It has been well-demonstrated that excessive activation of excitatory amino acid receptors can cause prolonged seizures (CitationVincent & Mulle, 2009). The free radicals are generated as a result of overexcitation of excitatory amino acid receptors and thus they play a critical role in seizure genesis (CitationObay et al., 2008). Thus, intervention by antioxidants can be a potential beneficial approach in the treatment of epilepsy.
In the present study, KA produced behavioral changes as well as convulsions in all the animals. HAEEO pretreatment dose-dependently increased the latency of onset of seizures as compared with the vehicle-treated KA group. Moreover, HAEEO (500 and 700 mg/kg, i.p.) pretreatment prevented KA-induced oxidative stress. In the KA per se group, there was a significant increase in the levels of TBARS and a significant decrease in the levels of GSH suggesting thereby the production of oxidative stress. TBARS is an end product of lipid peroxidation, a measure of free radical generation. The significantly less increase in TBARS levels in the groups treated with HAEEO as compared with the vehicle-treated KA group indicates attenuation of lipid peroxidation. Also, there was a simultaneous significant increase in the GSH levels in the HAEEO (500 and 700 mg/kg, i.p.) group as compared with vehicle-treated KA group. GSH is the most abundant intracellular thiol and low-molecular-weight tripeptide found in living cells (CitationForman et al., 2009). It reacts with the free radicals and can protect cells from singlet oxygen, hydroxyl radical, and superoxide radical damage.
The increase in levels of GSH by HAEEO indicates its antioxidant property possibly by increasing the endogenous defensive capacity of the brain to combat oxidative stress induced by KA. Experimental studies have reported the potent antioxidant property of the fruit of E. officinalis (CitationYokozawa et al., 2007; CitationSharma et al., 2009). Various phytochemical constituents of the plant such as emblicanins A and B, gallic acid, ellagic acids have been identified as powerful free radical scavengers (CitationPozharitskaya et al., 2007). Moreover, other phytochemicals with NO scavenging properties such as geraniin, corilagin, and furosin have been reported in the E. officinalis fruit extract (CitationKumaran and Karunakaran, 2006). A recent study by CitationSaito et al. (2008) has also reported that the superoxide scavenging properties of E. officinalis extract approximates that of l-ascorbic acid, a well-established antioxidant. It is our contention that the animals will exhibit seizures when a threshold value of excitation is reached after administration of KA. The KA administration stimulates glutamate receptors, which enhances ROS level, which in turn will enhance glutaminergic activity (CitationUeda et al., 2002). It is difficult to predict at what level HAEEO acts. However, it is evident that it prevents the vicious chain, thus decreasing the excitotoxicity and thereby showing the protective effect. Hence, a dual effect of HAEEO acting both as an antioxidant and an anti-excitotoxic agent cannot be ruled out.
Inflammation is known to participate in the mediation of a growing number of acute and chronic neurological disorders. However, the role of inflammatory responses in the pathogenesis of epilepsy and seizure-induced brain damage has been appreciated only recently. Inflammatory processes, including activation of microglia and astrocytes and production of proinflammatory cytokines such as TNF-α, IL-1β, IL-6, and related molecules, have been described in human epilepsy patients as well as in experimental models of epilepsy (CitationVezzani & Granata, 2005; CitationChoi & Koh, 2008). TNF-α, IL-1β, and IL-1ra are highly inducible under different forms of stress, such as excitatory neurotransmitter excess occurring during seizures, in infection and inflammation, and during neurotrauma (CitationBartfai et al., 2007). For these reasons, KA has been adapted for studies of various neurological disorders involving excitotoxicity and inflammation, such as epilepsy, Huntington’s chorea, and Alzheimer’s disease (CitationLee et al., 2008). In the present study, we have observed that KA causes induction of brain levels of TNF-α. HAEEO (500 and 700 mg/kg, i.p.) significantly attenuate the KA-induced rise in the brain level of TNF-α (P < 0.001). Previous study by CitationPramyothin et al. (2006) also reported that E. officinalis extract lowered the ethanol-induced rise in the levels of TNF-α and IL-1β in rats.
COX-2 is the inducible isoform expressed at the injury/inflammation sites and constitutively at the central nervous system, and plays a role in neurodegenerative diseases associated with increased excitatory activity (CitationTeismann et al., 2003). The excitatory neurotransmitter glutamate by activating NMDA receptor leads to increased expression of COX-2 resulting in increased synthesis of prostaglandins (CitationBauer et al., 2008). COX-2 overexpression has been associated with neurotoxicity in acute conditions, such as hypoxia/ischemia and seizures (CitationMinghetti et al., 2007). Moreover, recently in our laboratory we found that HAEEO possesses potent anti-inflammatory activity in both acute and chronic model of inflammation (unpublished observations). This finding is supported by the study of CitationYokozawa et al. (2007) in which ethyl acetate extract of E. officinalis reduced the iNOS and COX-2 expression levels by inhibiting NF-κB activation. In the light of above-mentioned observations, it may be reasonable to assume that the potent antioxidant and anti-inflammatory effects of E. officinalis contributes to the anticonvulsant activity exhibited by HAEEO.
In the present study, it was observed that the administration of KA resulted in seizures that were associated with cognitive impairment as evidenced by reduction of RL in passive avoidance behavior. These results are in conformity with findings of other workers who also demonstrated cognitive impairment after administration of chemoconvulsants (CitationGrecksch et al., 1991; CitationBecker et al., 1995; CitationHomayoun et al., 2001). The administration of HAEEO for seven consecutive days dose-dependently prevented cognitive deficit associated with KA-induced seizures as indicated by increased RL in passive avoidance behavior. All major antiepileptic drugs (AEDs) have been reported to be associated with cognitive side effects, though uncertainty exists regarding the degree of cognitive deficits (CitationHessen et al., 2006).
In the present study, 700 mg/kg, i.p., dose of HAEEO was most effective in attenuating the KA-induced seizures as well as cognitive impairment in rats. Our study also demonstrates that HAEEO can prevent cognitive impairment following SE and may also act as a useful adjuvant drug in addition to the available conventional AEDs in the treatment of SE.
Declaration of interest
The authors report no conflicts of interest. The authors alone are responsible for the content and writing of the article.
References
- Atlante A, Calissano P, Bobba A, Giannattasio S, Marra E, Passarella S. (2001). Glutamate neurotoxicity, oxidative stress and mitochondria. FEBS Lett, 497, 1–5.
- Bartfai T, Sanchez-Alavez M, Andell-Jonsson S, Schultzberg M, Vezzani A, Danielsson E, Conti B. (2007). Interleukin-1 system in CNS stress: Seizures, fever, and neurotrauma. Ann N Y Acad Sci, 1113, 173–177.
- Bauer B, Hartz AM, Pekcec A, Toellner K, Miller DS, Potschka H. (2008). Seizure-induced up-regulation of P-glycoprotein at the blood–brain barrier through glutamate and cyclooxygenase-2 signaling. Mol Pharmacol, 73, 1444–1453.
- Becker A, Grecksch G, Brosz M. (1995). Antiepileptic drugs—their effects on kindled seizures and kindling-induced learning impairments. Pharmacol Biochem Behav, 52, 453–459.
- Bertram EH. (2009). Temporal lobe epilepsy: Where do the seizures really begin? Epilepsy Behav, 14 (Suppl 1), 32–37.
- Biswas NR, Gupta SK, Das GK, Kumar N, Mongre PK, Haldar D, Beri S. (2001). Evaluation of Ophthacare eye drops—a herbal formulation in the management of various ophthalmic disorders. Phytother Res, 15, 618–620.
- Carreño M, Donaire A, Sánchez-Carpintero R. (2008). Cognitive disorders associated with epilepsy: Diagnosis and treatment. Neurologist, 14, S26–S34.
- Chandra RH, Veeresham C, Asres K. (2004). Effect of Emblica officinalis commercial formulation in type 2 diabetic patients. Indian J Pharma Sci, 66, 735–738.
- Chang BS, Lowenstein DH. (2003). Epilepsy. N Engl J Med, 349, 1257–1266.
- Chen J, Quan QY, Yang F, Wang Y, Wang JC, Zhao G, Jiang W. (2010). Effects of lamotrigine and topiramate on hippocampal neurogenesis in experimental temporal-lobe epilepsy. Brain Res, 1313, 270–282.
- Cherlyee CWJ, Thomas PB. (1995). Status epilepticus. Neurol Clin, 13, 529–548.
- Choi J, Koh S. (2008). Role of brain inflammation in epileptogenesis. Yonsei Med J, 49, 1–18.
- Dong XX, Wang Y, Qin ZH. (2009). Molecular mechanisms of excitotoxicity and their relevance to pathogenesis of neurodegenerative diseases. Acta Pharmacol Sin, 30, 379–387.
- Ellman GL. (1959). Tissue sulfhydryl groups. Arch Biochem Biophys, 82, 70–77.
- Forman HJ, Zhang H, Rinna A. (2009). Glutathione: Overview of its protective roles, measurement, and biosynthesis. Mol Aspects Med, 30, 1–12.
- Grecksch G, Becker A, Gadau C, Matthies H. (1991). Gangliosides improve a memory deficit in pentylenetetrazol-kindled rats. Pharmacol Biochem Behav, 39, 825–828.
- Gupta YK, Briyal S. (2006). Protective effect of vineatrol against kainic acid induced seizures, oxidative stress and on the expression of heat shock proteins in rats. Eur Neuropsychopharmacol, 16, 85–91.
- Gupta YK, Briyal S, Sharma M. (2009). Protective effect of curcumin against kainic acid induced seizures and oxidative stress in rats. Indian J Physiol Pharmacol, 53, 39–46.
- Hessen E, Lossius MI, Reinvang I, Gjerstad L. (2006). Predictors of neuropsychological impairment in seizure-free epilepsy patients. Epilepsia, 47, 1870–1878.
- Homayoun H, Khavandgar S, Zarrindast MR. (2001). Effects of adenosine receptor agonists and antagonists on pentylenetetrazole-induced amnesia. Eur J Pharmacol, 430, 289–294.
- Jacob A, Pandey M, Kapoor S, Saroja R. (1988). Effect of the Indian gooseberry (amla) on serum cholesterol levels in men aged 35–55 years. Eur J Clin Nutr, 42, 939–944.
- Kardos J. (1999). Recent advances in GABA research. Neurochem Int, 34, 353–358.
- Khan KH, Khan KH. (2009). Roles of Emblica officinalis in medicine—a review. Bot Res Int, 2, 218–228.
- Kim HY, Okubo T, Juneja LR, Yokozawa T. (2010). The protective role of amla (Emblica officinalis Gaertn.) against fructose-induced metabolic syndrome in a rat model. Br J Nutr, 103, 502–512.
- Kirtikar KR, Basu BD. (1996). Indian Medicinal Plants. Dehradun, India: International Book Distributor.
- Kumaran A, Karunakaran RJ. (2006). Nitric oxide radical scavenging active components from Phyllanthus emblica L. Plant Foods Hum Nutr, 61, 1–5.
- Lee JK, Won JS, Singh AK, Singh I. (2008). Statin inhibits kainic acid-induced seizure and associated inflammation and hippocampal cell death. Neurosci Lett, 440, 260–264.
- Minghetti L. (2007). Role of COX-2 in inflammatory and degenerative brain diseases. Subcell Biochem, 42, 127–141.
- Mohamad A, Mikati MA, Tarif S, Lteif L, Jawad MA. (2001). Time sequence and types of memory deficits after experimental status epilepticus. Epilepsy Res, 43, 97–101.
- Montgomery EM, Bardgett ME, Lall B, Csernansky CA, Csernansky JG. (1999). Delayed neuronal loss after administration of intracerebroventricular kainic acid to preweanling rats. Brain Res Dev Brain Res, 112, 107–116.
- Obay BD, Tasdemir E, Tümer C, Bilgin HM, Atmaca M. (2008). Dose dependent effects of ghrelin on pentylenetetrazole-induced oxidative stress in a rat seizure model. Peptides, 29, 448–455.
- Ohkawa H, Ohishi N, Yagi K. (1979). Assay for lipid peroxides in animal tissues by thiobarbituric acid reaction. Anal Biochem, 95, 351–358.
- Pozharitskaya ON, Ivanova SA, Shikov AN, Makarov VG. (2007). Separation and evaluation of free radical-scavenging activity of phenol components of Emblica officinalis extract by using an HPTLC-DPPH* method. J Sep Sci, 30, 1250–1254.
- Pramyothin P, Samosorn P, Poungshompoo S, Chaichantipyuth C. (2006). The protective effects of Phyllanthus emblica Linn. extract on ethanol induced rat hepatic injury. J Ethnopharmacol, 107, 361–364.
- Rao RS, Prakash A, Medhi B. (2009). Role of different cytokines and seizure susceptibility: A new dimension towards epilepsy research. Indian J Exp Biol, 47, 625–634.
- Said SI, Pakbaz H, Berisha HI, Raza S. (2000). NMDA receptor activation: Critical role in oxidant tissue injury. Free Radic Biol Med, 28, 1300–1302.
- Saito K, Kohno M, Yoshizaki F, Niwano Y. (2008). Extensive screening for edible herbal extracts with potent scavenging activity against superoxide anions. Plant Foods Hum Nutr, 63, 65–70.
- Schmidt MJ, Ryan JJ, Molloy BB. (1976). Effects of kainic acid, a cyclic analogue of glutamic acid, on cyclic nucleotide accumulation in slices of rat cerebellum. Brain Res, 112, 113–126.
- Schwarzer C, Sperk G. (1995). Hippocampal granule cells express glutamic acid decarboxylase-67 after limbic seizures in the rat. Neuroscience, 69, 705–709.
- Sharma A, Sharma MK, Kumar M. (2009). Modulatory role of Emblica officinalis fruit extract against arsenic induced oxidative stress in Swiss albino mice. Chem Biol Interact, 180, 20–30.
- Sierra-Paredes G, Sierra-Marcuño G. (2007). Extrasynaptic GABA and glutamate receptors in epilepsy. CNS Neurol Disord Drug Targets, 6, 288–300.
- Silva B, Oliveira PJ, Dias A, Malva JO. (2008). Quercetin, kaempferol and biapigenin from Hypericum perforatum are neuroprotective against excitotoxic insults. Neurotox Res, 13, 265–279.
- Stubley-Weatherly L, Harding JW, Wright JW. (1996). Effects of discrete kainic acid-induced hippocampal lesions on spatial and contextual learning and memory in rats. Brain Res, 716, 29–38.
- Teismann P, Vila M, Choi DK, Tieu K, Wu DC, Jackson-Lewis V, Przedborski S. (2003). COX-2 and neurodegeneration in Parkinson’s disease. Ann N Y Acad Sci, 991, 272–277.
- Ueda Y, Yokoyama H, Nakajima A, Tokumaru J, Doi T, Mitsuyama Y. (2002). Glutamate excess and free radical formation during and following kainic acid-induced status epilepticus. Exp Brain Res, 147, 219–226.
- Vezzani A, Granata T. (2005). Brain inflammation in epilepsy: Experimental and clinical evidence. Epilepsia, 46, 1724–1743.
- Vincent P, Mulle C. (2009). Kainate receptors in epilepsy and excitotoxicity. Neuroscience, 158, 309–323.
- Wu Z, Xu Q, Zhang L, Kong D, Ma R, Wang L. (2009). Protective effect of resveratrol against kainate-induced temporal lobe epilepsy in rats. Neurochem Res, 34, 1393–1400.
- Yokozawa T, Kim HY, Kim HJ, Okubo T, Chu DC, Juneja LR. (2007). Amla (Emblica officinalis Gaertn.) prevents dyslipidaemia and oxidative stress in the ageing process. Br J Nutr, 97, 1187–1195.
- Zaja-Milatovic S, Gupta RC, Aschner M, Montine TJ, Milatovic D. (2008). Pharmacologic suppression of oxidative damage and dendritic degeneration following kainic acid-induced excitotoxicity in mouse cerebrum. Neurotoxicology, 29, 621–627.