Abstract
Context: Traditional Herbal Medicine (THM) has many advantages that make it a promising choice for the treatment of ischemic heart disease (IHD). To study the mechanism of IHDs or pharmacological actions of THM, many hypoxia-induced cardiomyocyte injury models have been established. Radix Salvia miltorrhiza (Danshen) was used as a representative of THM. Danshen is a famous medicinal herb widely applied in Asia to relieve ischemic cardiovascular diseases.
Objective: To investigate the effects of various hypoxic conditions and discuss a suitable hypoxia model, cell viability, apoptosis, release of myocardial injury markers, and mRNA levels of target genes were tested for the first time.
Materials and methods: Radix Salvia miltorrhiza (Danshen) was purchased from a GMP-compliant producer and both its preparation method and quality control were standardized. Cellular status, such as cell viability, apoptosis, releases of myocardial injury markers, and the mRNA level of target gene were tested by 3-[4,5-dimethylthiazol-2-yl]-2,5-diphenyltetrazolium bromide (MTT) method, biochemical analyzer, flow cytometry, Hoechst 33258 staining, and real-time PCR, respectively.
Results: Based on our data, we found a treppe response of cardiomyocyte in the hypoxic condition and suggested that 8 h in 2% O2 might be a suitable condition for in vitro pharmacological study of cardiomyocytes.
Discussion and conclusions: Our findings outlined more extended and in-depth capability of cardiomyocyte suffering from hypoxia, and might be of particular interest due to the high prevalence of THM pharmacological study.
Introduction
Recently, ischemic heart disease (IHD) has become one of the great threats to human health. It is well demonstrated that hypoxia-induced cardiomyocyte death is involved in IHD. To simulate the pathological hypoxic condition and further study the pharmacological action of Traditional Herbal Medicine (THM), many hypoxia models have been established.
As reported in recent related studies, the most prevalent oxygen concentration is 0% or 1%, and hypoxia time varies from 1 h to 24 h (CitationKulisz et al., 2002; CitationSun et al., 2005; CitationWang et al., 2009; CitationZhang et al., 2009). Actually, the differences between hypoxic models are not as simple as it appears, and usually the expense of hypoxia model establishment (nitrogen consumption, etc.) is a great burden for many researchers, especially when they are from a developing country where most natural products are processed. And most importantly, different hypoxia models show different effects on cellular status, such as hormone secretion (CitationGoetze et al., 2004), growth curve (CitationD’Ippolito et al., 2006), apoptosis (CitationMuschel et al., 1995), and so on. Even though almost all the hypoxia models show damage to cardiomyocytes, details and real-time actions are still poorly understood. Therefore, in this study, we established many physical hypoxia models (including all the popular models) to determine the effects of various hypoxic conditions on cardiomyocyte with the cellular parameters prevalent in pharmacological study, and tried to find a suitable condition based on our data.
To study whether our condition would suit natural-product study, we used Radix Salvia miltorrhiza (Danshen) as a representative of THM. Danshen is a famous medicinal herb widely applied in Asia to relieve ischemic cardiovascular diseases, and its protection for ischemic myocardium has been corroborated in both clinical and laboratory studies (CitationZhu et al., 2004).
Materials and methods
Materials
The Danshen injection (DSI) was purchased from Sanchine-Sunnyhope Pharmaceutical Co., Ltd. (Chengdu, Sichuan, China). Its preparation was approved by the State Food and Drug Administration (#Z51021303) of China. In detail, the air-dried root of Danshen (Danshen was planted in Sichuan in March and harvested in November of the same year) was prepared in a powdered form and sifted through a 20-mesh sieve (aperture 0.94 mm). Dry powder of Danshen (1.5 kg) was extracted with hot water for three times (2 h for the first time, and 1.5 h for each of the latter two times), and the condensate was extracted through decompression till 750 mL was left. Then, the product was precipitated twice with ethanol solution (concentration: 75% for the first time and 85% for the second time). The preparation was then stored overnight under cold condition and then filtered. The filtrate of the extract was concentrated, dried, and then dissolved in 650 mL water for injection. Then, the preparation was stored overnight in cold and filtered again until the pH value was adjusted to 6.8. The preparation was boiled for 0.5 h, and the extract was filtered, fixed to 1000 mL, and sterilized. The product was named DSI and stored in a dark and cool place.
The concentration of Danshen in DSI is 1.5 g/mL. For clinical drip feed, the DSI was diluted in 5% glucose injection (1:10–50), 10 mL DSI qd. For in vitro study, the DSI was diluted in Dulbecco’s modified Eagle’s medium (DMEM) and sonicated. The main components in the extract is as follows (CitationXu et al., 2008; analyzed by high-performance liquid chromatography method; per mL DSI): Danshensu (1232.70 µg); protocatechuic aldehyde (260.36 µg); caffeic acid (18.34 µg); salvianolic acid D (37.57 µg); rosmarinic acid (95.33 µg); salvianolic acid B (62.65 µg); and salvianolic acid A (16.23 µg). In Pharmacopoeia of the People’s Republic of China (2005), Danshensu and protocatechuic aldehyde are used as marker compounds for standardization of the extract.
DMEM, fetal bovine serum (FBS), and trypsin were purchased from GiBCO (Gaithersburg, MD). Antibodies and reagents (sc-58671, sc-2082, sc-2043) used for immunofluorescence in this study were obtained from Santa Cruz Biotechnology, Inc. (Santa Cruz, CA). 3-[4,5-dimethylthiazol-2-yl]-2,5-diphenyltetrazolium bromide (MTT), and collagenase (type 2) were obtained from Sigma (St. Louis, MO). Annexin V-FITC FLOUS staining kit and Roche modular DDP System were obtained from Roche Diagnostics (Indianapolis, IN).
Cell culture and immunofluorescence staining
Primary cultures of neonatal rat cardiomyocytes were prepared as previously described with minor modifications (CitationHoffmann et al., 1995). The hearts were isolated and minced into 1–2 mm3 fragments, and were then enzymatically digested 3–4 times for 5 min each in phosphate-buffered saline (PBS; without Ca2+) containing 0.1% collagenase (type 2) and 0.1% trypsin. The liberated cells from each digestion were collected in DMEM supplemented with 10% FBS and centrifuged, and then resuspended in DMEM with 10% FBS. The cell suspensions were uniformly mixed and plated onto a cell culture flask for 30-min cultivation (37°C in a 5% CO2 incubator) for purification. After that, cells were plated onto 6- and 96-well plates and were maintained in a humidified atmosphere with 5% CO2 at 37°C. 5-Bromo-29-deoxyuridine (BrdU; 100 mM) was added during the first 2 days to inhibit proliferation of nonmyocytes. The cells were used after being cultured for 3 days.
To identify cardiomyocytes, immunofluorescence staining was used. Briefly, cells were fixed in 4% paraformaldehyde for 15 min, after several wash with PBS, and then blocked in 10% goat serum for 1 h, after that the cells were incubated overnight at 4°C with rabbit polyclonal anti-Sarcomeric actin (1:100). After thorough washing, the fluorescein isothiocyanate–conjugated secondary antibody (1:100) was applied for 25 min in a dark place at room temperature. Fluorescent images were captured using a fluorescence microscope (Olympus IX70, Japan).
Hypoxia treatment and experimental grouping
The hypoxic condition was produced by an oxygen-control incubator (Thermo Fisher Scientific Inc, Waltham, MA). Cardiomyocytes were randomly grouped into 9 groups, and then hypoxic-treated for 0, 4, 6, 8, 12, 18, 24, 30, 48 h, respectively. To study the effects of different oxygen concentrations on cell and further explore the suitable hypoxia condition, we also challenged the cells by different oxygen concentrations (1% O2 and 2% O2) for 4, 8, and 30 h. After hypoxia treatment, some cellular parameters that were usually present in the pharmacological study would be tested. To investigate whether our model would be suitable for natural-product pharmacological study, the following groups were set: cells cultured in normal oxygen condition (normal control); cells cultured in 2% O2 for 8 h (hypoxia); cells pretreated with 1.8 mg/mL Danshen and then cultured in 2% O2 for 8 h (hypoxia + Danshen).
Cell viability and LDH release assay
Cell viability was measured by MTT assay. Cells were seeded on 96-well plates and incubated for an indicated time period. Following exposure to hypoxia, MTT solution was added into each well to make a final concentration of 0.5 mg/mL, and then the plates were incubated for an additional 4 h. The medium was removed, 150 µL DMSO was added into each well, and the preparation was oscillate-mixed for 5 min before colorimetric reading at 490 nm by a microplate reader (Molecular Devices, Sunnyvale, CA)
Besides, we also measured the release of lactate dehydrogenase, an inner cell enzyme, by enzyme rate method (Roche Modular DDP System, USA).
Metabolic assay
Glucose and lactate content were tested using a hexokinase method (Roche modular DDP System) and dry chemical method (Ortho Clinical Diagnostic Systems, Johnson & Johnson, Rochester, NY) according to the manufacturer’s instructions. The values of glucose and lactate concentration in the cultured supernatant were used to indicate the metabolic status of cardiomyocyte in different groups.
Hoechst staining
Hoechst 33258 staining was used for the assessment of apoptotic cardiomyocytes. Briefly, cells were fixed by 4% paraformaldehyde for 10 min at room temperature. After being washed by PBS, cells were exposed to Hoechst 33258 solution (5 µg/mL in PBS) and incubated for 10 min at room temperature. Following a final wash with PBS, cells were examined under a fluorescence microscope with an appropriate filter. The percentage of apoptotic cells displaying chromatin condensation, nuclear shrinkage, and fragmentation was determined.
Flow cytometry assay
Cell death was analyzed by flow cytometry following the manufacturer’s instructions. Briefly, after the different treatments, cells were detached by enzymatic digestion, and washed and resuspended in binding buffer (HEPES). And then cells were incubated for 10 min in the dark with Annexin V-FITC (1 µg/mL) and propidine iodide solution (5 µg/mL) at 4°C, and analyzed with a FACScan flow cytometer (BD FACSCalibur, Basking Ridge, NJ).
Real-time PCR
The mRNA levels of genes were measured by fluorescent quantitative real-time RT-PCR. Total RNA isolation and cDNA synthesis were done according to the manufacturer’s instructions. Real-Time PCR was performed using SYBR® Premix Ex Taq™ (type 2) and the iCycler real-time PCR detection system. The following primers were used: Hypoxia-inducible factor-1α (HIF-1α; forward primer, 5′-CCA GAT TCA AGA TCA GCC AGC A-3′; reverse primer, 5′-GCT GTC CAC ATC AAA GCA GTA CTC A-3′) and β-actin (forward primer, 5′-TGG AAT CCT GTG GCA TCC ATG AAA C-3′; reverse primer, 5′-TAA AAC GCA GCT CAG TAA CAG TCC G-3′).
Statistical analysis
All data were presented as mean ± SD of at least three independent experiments. The one-way ANOVA was used to compare numeric data among the four related experimental groups and the difference between groups was determined by further analysis with least significant difference test. p < 0.05 was considered to be of statistical significance.
Results and discussion
At the beginning of our research, we primary-cultured cardiomyocytes and identified them. was the morphological image of cardiomyocyte in inverted phase-contrast microscope. In , we can easily find that the sarcomeric actin (a myocardium-specific protein) is widely distributed in almost all the cells, which indicates the high purity of the primary cultures of cardiomyocytes. The percentage of positive cells (more than 96.5%) was calculated in 10 random horizons (data not shown).
Figure 1. Morphological observation and immunofluorescence of cardiomyocytes. (A) Primary cultures of neonatal rat cardiomyocytes at 36 h (100×). (B) Sarcomeric actin protein was revealed by immunofluorescence using a mouse polyclonal antibody to Sarcomeric actin (green fluorescence; 200×).
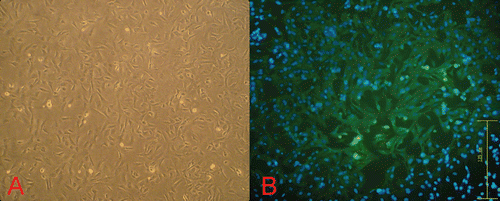
Currently, the establishment of a hypoxia model in vitro can be divided into physical and chemical hypoxia. A chemical hypoxic condition is usually established by CoCl2, because Co2+ can substitute Fe2+ in heme, an oxygen sensor, and block the combination of oxygen sensors and oxygen to simulate a hypoxic condition. By contrast, the physical hypoxia model was usually established by airproof gas-control capsule. Comparatively, physical hypoxia fits the low-oxygen environment, so physical hypoxia has become the most popular hypoxia model in the studies in vitro. Additionally, the oxygen-controlled cell incubator makes hypoxic condition establishment easier and more stable. Therefore, we used an oxygen-controlled cell incubator to simulate hypoxic conditions in our study.
Effects of different hypoxia conditions on cardiomyocytes
Since 1% O2 is the most prevalent concentration in in vitro study, our first step was to evaluate effects of different hypoxic durations in 1% O2. In , treppe response is present in the response of cardiomyocyte to hypoxia, and its entire period includes the rapid injury phase in the first 8 h, the plateau phase in 8–24 h, and a new round of injury. In detail, the cell viability of cardiomyocyte continuously decreased, while the lactate dehydrogenase (LDH) release increased in the first 8 h. During 8–24 h in hypoxia, there was not any significant difference in the two parameters. However, after 30 h of culture in hypoxia, marked increase (p < 0.05) appeared again. We extrapolated that hypoxic culture for a certain time period (about 8 h) would induce endogenous protective mechanism (CitationSun et al., 2005; such as inhibition of reactive oxygen species generation and intracellular Ca2+ overload) to protect against hypoxia injury. Therefore, 8–24 h of hypoxia did not aggravate damage to cardiomyocytes significantly. After 24 h in hypoxia, the endogenous protective mechanism might diminish or be insufficient to antagonize the hypoxic condition, so the damage caused by hypoxia was aggravated continuously. Although its underlying mechanism remains unclear, this phenomenon highlighted three time points with specific meaning (4th, 8th, and 30th h): 4th h (the beginning of hypoxia injury), although LDH release did not show any significant increase; 8th h (damage at relatively higher levels, which lasted for 16 h without marked changes); 30th h (a new round of increased injury). These data show that we need not culture cardiomyocytes for a long time under the hypoxic condition (1% O2), and that 8 h is enough to achieve significant changes in most cellular parameters that are used for pharmacological study.
Figure 2. Effects of hypoxic condition on cell viability and lactate dehydrogenase (LDH) release. (A) Cell viability and LDH release in 1% O2 in different hypoxic duration; (B) cell viability in different oxygen concentration; (C) LDH release in different oxygen concentration. (Data for cell viability are derived from five independent experiments; data for LDH release are derived from three independent experiments; *p < 0.05: compared with normoxic control group; #p < 0.05: Compared with the other treatment group respectively in the same time.)
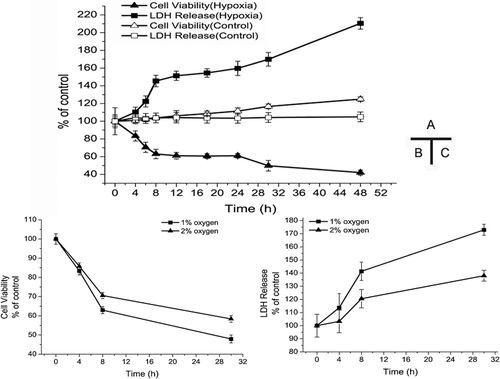
To explore the most suitable hypoxic condition, we also established models with different oxygen concentrations. To extract evidence from , we set three observation points (4th, 8th, and 30th h in hypoxia) to compare two oxygen concentration values in terms of cell viability. In , , when cells are cultured for 4 h in hypoxia, no significant difference exists between 1% O2 and 2% O2. However, at the 8th h in hypoxia, marked decreases occur between the two oxygen concentration values and it is the same as the 30th h in hypoxia. In summary, 1% O2 shows more damage on cells than 2% O2 in terms of both cell viability and LDH release, but the significant difference occur 4 h after hypoxia (with the exception of cell viability within 4 h). As to cell death, in line with cell viability and LDH release, the results of Hoechst 33258 staining () indicated that cells cultured in 1% O2 showed a higher apoptosis rate than those cultured in 2% O2, and significant increases occurred only 4 h after hypoxia. And it is hard to find apoptotic cells (almost less than 2%) in the staining result in normoxia group. These apoptosis data from Hoechst 33258 staining were further confirmed by the flowcytometry analysis, as is shown in .
Figure 3. Hoechst staining of cardiomyocyte in different oxygen concentrations. The Hoechst 33258 staining results in different oxygen concentration (200×). Apoptosis cells show nuclear fragmentation and condensation (red arrows). The apoptotic index was determined by the number of positively stained apoptotic cardiomyocytes/the total number of cardiomyocytes counted × 100%. (*p < 0.05: compared with each other.)
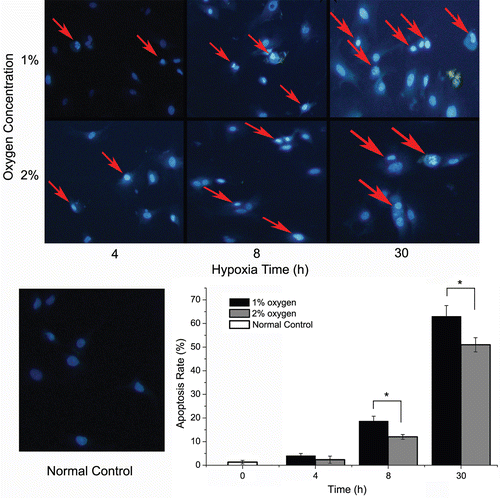
Figure 4. Flowcytometry analysis of cardiomyocytes in different oxygen concentrations. The apoptosis rates of cardiomyocyte in different oxygen concentrations were quantified by fluorescence-activated cell sorting analysis after staining with Annexin V and propidine iodide (PI). The data are from a representative experiment repeated three times with similar results.
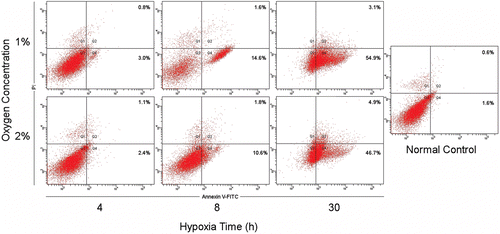
It is worthy of note that although less damage was caused by 2% O2 than by 1% O2, damage still increased significantly when compared to normoxia control. Our preliminary data showed that the replacement of 1% O2 by 2% O2 had saved us a lot of time and almost 30% nitrogen consumption. And most importantly, culturing cardiomyocytes under both 1% O2 and 2% O2 conditions for 8 h could induce hypoxia-related gene expression. HIF-1, a heterodimer composed of a constitutively expressed subunit HIF-1β and an oxygen-regulated subunit HIF-1α, is believed to play a key role in the regulation of hypoxic response in mammalian cells. In the present study, we determined the effects of different hypoxic conditions on the mRNA level of HIF-1α. As is shown by results in , 4, 8, and 30 h culture did not show any significant difference on the mRNA level of HIF-1α between 1% O2 and 2% O2. As a vital part of cell properties, energy status of the injury model was brought to researchers’ attention. So the levels of glucose uptake and lactate production were determined in various oxygen conditions. In the metabolic data (), there is not any marked change in different oxygen concentrations until 30 h.
Figure 5. mRNA levels and metabolic status in different oxygen concentrations. The mRNA levels of hypoxia-inducible factor-1α (HIF-1α) and metabolic status in different oxygen concentrations were quantified by real-time PCR or biochemical analyzer. The data are derived from five independent experiments. Total RNA of the treated cells was extracted and reverse transcribed. The relative mRNA levels for HIF-1α were calculated as described in the text and expressed as fold increase over control.
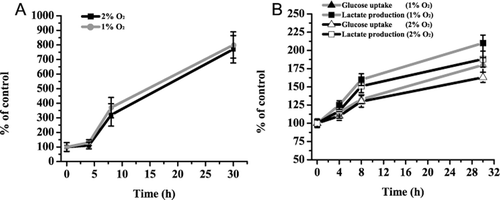
The above data strongly implied that culturing cardiomyocyte for 8 h in 2% O2 may be a suitable condition for hypoxia injury study in vitro in both economy and efficacy. Since we suggested a suitable condition, our further studies will focus on whether this condition would be suitable for natural-product pharmacological study.
Protective effects of Danshen in our model
Danshen is a famous herbal extract in compliance with national standards. It is widely used in clinical science to treat ischemic diseases in past decades, and its protection on ischemic myocardium has been corroborated. In this study, we chose this specific THM to investigate whether our model was suitable for natural-product pharmacological study.
Our three test groups and the results () indicated that our hypoxia models showed steady damage to cell viability and did not interfere with the protective effect of Danshen treatment. Both the normal control group vs. hypoxia group and hypoxia group vs. hypoxia + Danshen group were significant (p < 0.05). We analyzed the protective effect of Danshen against cell death in the hypoxia model with Hoechst 33258 staining and flowcytometry. In , the Hoechst 33258 staining indicated that cells exposed in our hypoxia model showed a great nuclear fragmentation and condensation, and that Danshen improved this condition. These results from Hoechst 33258 staining were further confirmed by flowcytometry analysis. As is shown in , our model increased both the apoptosis and necrosis rate of cardiomyocytes, and DSI significantly reduced hypoxia-induced cell death in our model. In a word, our hypoxia model–induced cardiomyocyte injury and Danshen significantly attenuated hypoxia-induced damage throughout the time. The results indicated that the condition we suggested was efficacious and did not interfere with the protective effects of Danshen.
Figure 6. Cell viability and LDH release in different treatment group. Normal control: cells cultured in normal oxygen condition; hypoxia: cells cultured in 2% O2 for 8 h; hypoxia + Danshen: cells pretreated with 1.8 mg/mL Danshen and then cultured in 2% O2 for 8 h. (Data for cell viability are derived from five independent experiments; data for LDH release are derived from three independent experiments; *p < 0.05: compared with normal control group; #p < 0.05: compared with hypoxia group.)
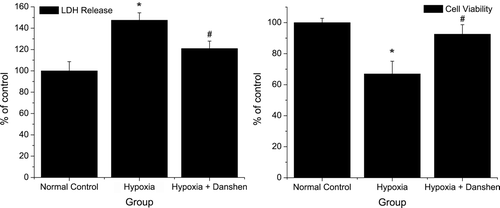
Figure 7. Apoptosis rate in different treatment groups. Normal control group: cells cultured in normal oxygen condition; hypoxia group: cells cultured in 2% O2 for 8 h; hypoxia + Danshen group: cells pretreated with 1.8 mg/mL Danshen and then cultured in 2% O2 for 8 h. Apoptosis cells show nuclear fragmentation and condensation (red arrows). The apoptotic index was determined by the number of positively stained apoptotic cardiomyocytes/the total number of cardiomyocytes counted ×100%. (*p < 0.05: compared with normal control group; #p < 0.05: compared with hypoxia group.)
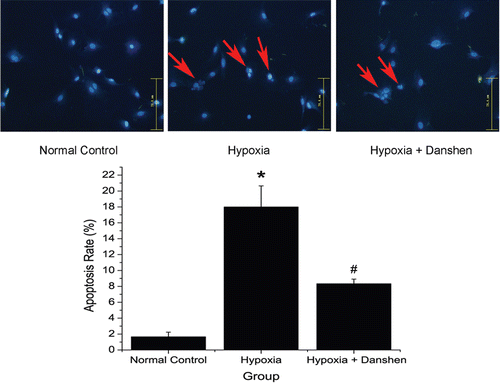
Figure 8. Flowcytometry analysis of cardiomyocytes in different treatment group. The apoptosis rates of cardiomyocyte in different treatment group were quantified by FACS analysis after staining with Annexin V and propidine iodide (PI). The data are from a representative experiment repeated three times with similar results.
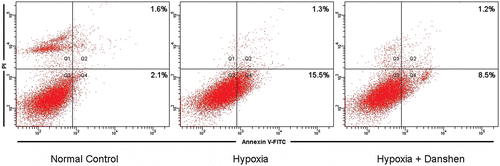
In conclusion, we established many cardiomyocyte hypoxia injury models in vitro, and tested cell states in different hypoxic conditions. We found the treppe response of cardiomyocyte in hypoxic condition, based on cell viability. In addition, we suggested a suitable condition (8 h in 2% O2) and evaluated this model by a THM. Our findings outlined more extended and in-depth capability of cardiomyocyte suffering from hypoxia and might be of particular interest due to the high prevalence of THM pharmacological study.
Acknowledgment
This research is supported by China National High-Tech R&D Program (or “the 863 Program”) [2007AA021900], and is a Key High-Tech Project of Sichuan Provincial Department of Science and Technology [No.2008SZ0035].
Declaration of interest
The authors report no conflicts of interest. The authors alone are responsible for the content and writing of the article.
References
- D’Ippolito G, Diabira S, Howard GA, Roos BA, Schiller PC. (2006). Low oxygen tension inhibits osteogenic differentiation and enhances stemness of human MIAMI cells. Bone, 39, 513–522.
- Goetze JP, Gore A, Møller CH, Steinbrüchel DA, Rehfeld JF, Nielsen LB. (2004). Acute myocardial hypoxia increases BNP gene expression. FASEB J, 18, 1928–1930.
- Hoffmann P, Richards D, Heinroth-Hoffmann I, Mathias P, Wey H, Toraason M. (1995). Arachidonic acid disrupts calcium dynamics in neonatal rat cardiac myocytes. Cardiovasc Res, 30, 889–898.
- Kulisz A, Chen N, Chandel NS, Shao Z, Schumacker PT. (2002). Mitochondrial ROS initiate phosphorylation of p38 MAP kinase during hypoxia in cardiomyocytes. Am J Physiol Lung Cell Mol Physiol, 282, L1324–L1329.
- Muschel RJ, Bernhard EJ, Garza L, McKenna WG, Koch CJ. (1995). Induction of apoptosis at different oxygen tensions: Evidence that oxygen radicals do not mediate apoptotic signaling. Cancer Res, 55, 995–998.
- Sun HY, Wang NP, Kerendi F, Halkos M, Kin H, Guyton RA, Vinten-Johansen J, Zhao ZQ. (2005). Hypoxic postconditioning reduces cardiomyocyte loss by inhibiting ROS generation and intracellular Ca2+ overload. Am J Physiol Heart Circ Physiol, 288, H1900–H1908.
- Wang Q, Liu HR, Mu Q, Rose P, Zhu YZ. (2009). S-propargyl-cysteine protects both adult rat hearts and neonatal cardiomyocytes from ischemia/hypoxia injury: the contribution of the hydrogen sulfide-mediated pathway. J Cardiovasc Pharmacol, 54, 139–146.
- Xu JZ, Shen J, Cheng YY, Qu HB. (2008). Simultaneous detection of seven phenolic acids in Danshen injection using HPLC with ultraviolet detector. J Zhejiang Univ Sci B, 9, 728–733.
- Zhang J, Liu A, Hou R, Zhang J, Jia X, Jiang W, Chen J. (2009). Salidroside protects cardiomyocyte against hypoxia-induced death: A HIF-1α-activated and VEGF-mediated pathway. Eur J Pharmacol, 607, 6–14.
- Zhu YZ, Huang SH, Tan BK, Sun J, Whiteman M, Zhu YC. (2004). Antioxidants in Chinese herbal medicines: A biochemical perspective. Nat Prod Rep, 21, 478–489.