Abstract
Context: Tournefortia sarmentosa Lam. (Boraginaceae), a Chinese herbal medicine, is commonly used as a detoxicant or anti-inflammatory agent.
Objective: As acetaminophen (APAP) is a well-known hepatotoxin, we investigated the effect of the aqueous extract of the T. sarmentosa on APAP-induced hepatotoxicity in vivo and in vitro.
Materials and methods: Levels of liver function markers serum glutamate oxaloacetate transaminase (SGOT), glutamate pyruvate transaminase (SGPT), and alkaline phosphatase (ALP), inflammatory markers tumor necrosis factor (TNF)-α, interleukin (IL)-1b, and IL-6 in serum, and antioxidant enzymes catalase (CAT), superoxide dismutase (SOD), and glutathione peroxidase (GPx), as well as lipid peroxidation were determined.
Results: T. sarmentosa significantly reduced the elevated liver function (SGOT, SGPT, and ALP, p < 0.01) and inflammatory markers (TNF-α, IL-1β, and IL-6, p < 0.01) in serum of APAP-intoxicated rats. Malondialdehyde level (p < 0.05) and antioxidant enzyme levels (CAT, SOD, and GPx, p < 0.05) were also reduced in APAP-intoxicated rats treated with T. sarmentosa. Incubation of rat hepatocyte cell line clone-9 cells with APAP reduced cell viability and increased the extent of lipid peroxidation. APAP stimulation also reduced the level of glutathione (GSH) and caused reduction in the activities of the antioxidant enzymes, CAT, SOD, and GPx. Pretreatment of hepatocytes with T. sarmentosa aqueous extract before and during APAP stimulation attenuated the extent of lipid peroxidation, increased cell viability and GSH level, and enhanced the activities of antioxidant enzymes.
Discussion and conclusion: These data suggest that the aqueous extract of T. sarmentosa can prevent APAP-induced hepatotoxicity.
Keywords::
Introduction
Acetaminophen [N-acetyl-p-aminophenol (APAP)] is a widely used analgesic and antipyretic drug. However, overdose of APAP is a frequent cause of acute hepatic failure and death (CitationChun et al., 2009). Additionally, chronic APAP usage may also cause hepatotoxicity even in low dosages in adults or children (CitationGeiger & Howard, 2007). The normal dose of APAP is conjugated by glucronic acid and eliminated in bile without any toxicity. However, levels of APAP over the limit of detoxification by glucronic acid conjugation are metabolized by cytochrome P-450 and form a chemically reactive metabolite, N-acetyl-p-benzoquinonimine (NAPQI) (CitationJosephy, 2005). NAPQI is conjugated with glutathione (GSH) and this reaction induces GSH depletion, which causes reactive oxygen species (ROS) generation (CitationHinson et al., 2004). APAP also induces oxidative stress causing significant alternation in the activities of some antioxidant enzymes in free-radical metabolism, such as superoxide dismutase (SOD), catalase (CAT), and glutathione peroxidase (GPx) (CitationHinson et al., 2004). Maintaining the balance between ROS and antioxidant enzymes is, therefore, crucial and could serve as a major mechanism in preventing liver injury by oxidative stress. This balance has been suggested to play an important role in APAP-induced hepatotoxicity. In addition, increasing evidence has indicated that inflammatory processes are involved in APAP-induced hepatotoxicity (CitationJaeschke, 2005). Recent data have implicated molecular mediators of the immune response such as tumor necrosis factor-α (TNF-α), interleukin (IL)-1β, and IL-6 in acute and chronic liver damage (CitationLacour et al., 2005). These cytokines have been implicated in mediating the hepatic response to APAP (CitationBlazka et al., 1995; CitationBourdi et al., 2002; CitationJames et al., 2003). Furthermore, these cytokines are released into the bloodstream both from the liver and from distal sites during hepatic toxic injury (CitationLacour et al., 2005).
Many antioxidant agents have been studied in experimental studies to reduce or prevent APAP-induced hepatotoxicity. Tournefortia sarmentosa Lam. (Boraginaceae) has been used as detoxicant and anti-inflammatory agents, and a circulation promoter to remove blood stasis (CitationChiu & Chang, 1987). It has been reported that the aqueous ethanol extract and water-soluble fraction from the stems of T. sarmentosa potently inhibited low-density-lipoprotein peroxidation (CitationLin et al., 1999, Citation2002). Since T. sarmentosa is reported as an antioxidant, it might be useful for preventing APAP-induced hepatotoxicity.
The aim of this study was to investigate the protective effect of the aqueous extract of T. sarmentosa on liver function and inflammatory markers in the serum, as well as on the levels of antioxidant enzymes and lipid peroxidation of the rats administrated with APAP. Serum levels of liver function markers serum glutamate oxaloacetate transaminase (SGOT), glutamate pyruvate transaminase (SGPT), and alkaline phosphatase (ALP), as well as inflammatory markers TNF-α, IL-1β, and IL-6 were determined. The extent of lipid peroxidation was determined by measuring the content of malondialdehyde (MDA), and the prooxidant−antioxidant status was evaluated by measuring the activity of the antioxidant enzymes SOD, CAT, and GPx in rat liver homogenates. In addition, an in vitro cell culture model was used to study the effect of T. sarmentosa on APAP-induced cytotoxicity in rat hepatocyte cell line clone-9 cells. The cellular damage was assessed by determining the cell viability in APAP-treated clone-9 cells. To evaluate whether the water extract of T. sarmentosa has antioxidative properties, the MDA content, GSH level, and activity of the antioxidant enzymes in APAP-treated clone-9 hepatocytes were examined.
Materials and methods
Reagents
All culture materials were purchased from Gibco (Grand Island, NY, USA). The MDA assay kit (Cat.No. LPO-586) was purchased from Calbiochen (La Jolla, CA). The GSH (Cat. No. CS0260), GPx (Cat. No. CGP1), SOD (Cat. No. 19160), and CAT (Cat. No. CAT100) activity assay kits were purchased from Sigma (St Louis, MO). Enzyme-linked immunosorbent assays (ELISAs) for TNF-α, IL-1β, and IL-6 were purchased from R&D Systems (Minneapolis, MN). Other chemicals of reagent grade were obtained from Sigma.
Preparation of the extract
Stems from field grown T. sarmentosa were harvested, dried, and chopped into about 5-cm pieces with fodder cutter. The dried pieces were extracted with distilled water for 120 min at 100°C. The water extracts were then centrifuged at 4°C and 3,000 rpm for 3 min, and filtered through Whatman No. 3 filter paper. After filtration, it was dried using a rotary vacuum evaporator at 60°C for 3 h. Dried T. sarmentosa extracts were resuspended in distilled water and stored at −20°C until use.
Experimental animals and design
Pathogen-free male Wistar-albino rats (4 weeks) were purchased from the National Laboratory Animal Center, Taiwan, and were kept for a week under environmentally controlled conditions. All animals were fed with a standard rat chow diet and water ad libitum, and then rats weighing 180 ± 20 g were used for induction of APAP-induced hepatotoxicity. All rats received human care in accordance to the “Guide for the Care and Use of Laboratory Animals” (National Academies Press, Washington, DC, USA, 1996).
Animals were divided into four groups of eight animals each. Group I treated with vesicle (distilled water) was kept as normal control. Group II served as hepatotoxicity control and was treated with a single dose of APAP (2,000 mg/kg body, p.o.). Group III and IV were treated with extract of T. sarmentosa 250 and 500 mg/kg body weight plus APAP. After 24 h of APAP intoxication, the rats were euthanized by ether and then sacrificed. The blood was collected by cardiac puncture in heparinized tubes. The blood was centrifuged at 3,000 rpm and 4°C for 10 min to separate the serum. Serum SGOT, SGPT, and ALP were measured with an auto-analyzer (Hitachi 7050, Tokyo, Japan).
The liver was immediately taken out and washed with ice-cold saline, then weighed and stored at −80°C. Ten percent of homogenate was prepared in 0.05-M ice-cold Tris−HCl buffer (pH 7.4) by using a glass Teflon homogenizer. The homogenate was then centrifuged at 5,000 rpm for 30 min to remove debris. Supernatant fluids were collected and used for the estimation of SOD, CAT, GPx, and the level of lipid peroxidation, and total protein.
ELISA for serum TNF-α, IL-1β, and IL-6 levels
The levels of serum TNF-α, IL-1β, and IL-6 were determined by using sandwich ELISA (sensitivity 18 pg/mL; R&D) according to manufacturer’s protocols, as previously described (CitationLee et al., 2006).
Cell culture
Rat liver clone-9 cells were cultured in Dulbecco’s modified Eagle’s medium containing 10% fetal bovine serum at 37°C in a humidified atmosphere of 5% CO2, as previously described (CitationSahu et al., 2008). The clone-9 cells kept in culture medium was served as normal control. The toxin control was prepared by incubating the clone-9 cells with APAP for 24 h. Before administrating with APAP, clone-9 cells were pretreated with 0−100 μg/mL aqueous extract of T. sarmentosa for 1 h prior to APAP addition. At the end of incubation period, clone-9 cells were collected to carry out each experiments in triplicates.
Measurement of cell viability by MTT assay
Cells were cultured on monomer or polymerized collagen in 96-well plates. Cell viability was determined by methylthiazol tetrazolium assay (MTT) assay. After the incubation period, 3-(4,5-dimethylthiazol-2-yl)-2,5-diphenyltetrazolium bromide (MTT) solution was added to each well to a final concentration of 0.5 mg/mL and the mixture was incubated at 37°C for 3 h to allow MTT reduction. The formazan crystals were dissolved by adding dimethylsulfoxide and absorbance was measured at 570 nm with a spectrophotometer (CitationChu et al., 2007).
Determination of antioxidant enzyme activities
The SOD, CAT, and GPx enzyme activities, and the level of GSH in clone-9 hepatocytes were measured in triplicate by assay
kits from Sigma using 96-well plates. The protein content was determined with the Bradford method using BioRad protein assay (BioRad, Espoo, Finland) with bovine serum albumin (Sigma) as the standard.
MDA assay for lipid peroxidation
The treated cells were incubated in 24-well plates. The culture medium was removed and 0.5-mL cell lysis buffer was added to each well after cells were washed three times with phosphate-buffered saline. The MDA contents of cell were determined by MDA assay kit.
Statistical analysis
The results are expressed as mean ± standard error of the mean. Statistical analysis was determined by using an independent Student’s t-test for two groups of data and analysis of variance followed by Scheffe’s test for multiple comparisons. P values less than 0.05 were considered significant.
Results
Effect of T. sarmentosa extract on APAP-induced hepatotoxicity in rat
Serum activities of SGOT, SGPT, and ALP are summarized in . The leakage of these enzyme activities in the blood reflects the damage of liver function due to APAP-induced hepatotoxicity. Administration of APAP significantly elevated SGOT, SGPT, and ALP activities when compared with the normal control rats. Co-treatment with 250 or 500 mg/kg of T. sarmentosa extract significantly reduced the elevation of SGOT, SGPT, and ALP (p < 0.01).
Table 1. Effect of T. sarmentosa extract on liver function and inflammatory markers in APAP-administrated rats.
Activities of hepatic SOD, CAT, and GPx are provided in the . Amounts of SOD, CAT, and GPx were significantly diminished in the APAP-administrated rats as compared with the normal control rats (p < 0.01). Co-treatment of T. sarmentosa extract significantly raised the antioxidant enzyme levels as compared with the rats administrated with APAP. In addition, administration of APAP caused a significant increase in MDA level when compared with the normal control rats. However, rats co-treated with 250 or 500 mg/kg of T. sarmentosa extract significantly reduced MDA concentration in the rat liver homogenate as compared with APAP-administrated rats ().
Table 2. Effect of T. sarmentosa extract on liver antioxidant enzyme activities and lipid peroxidation levels in APAP-administrated rats.
Effect of T. sarmentosa extract on serum inflammatory markers in APAP-administrated rat
Serum levels of TNF-α, IL-1β, and IL-6 are shown in . Administration of APAP significantly increased TNF-α, IL-1β, and IL-6 concentrations when compared with the normal control. Co-treatment with 250 or 500 mg/kg of T. sarmentosa extract significantly reduced the elevation of TNF-α, IL-1β, and IL-6 (p < 0.01).
Effect of T. sarmentosa extract on APAP-induced cell death/survival in clone-9 hepatocytes
As T. sarmentosa extract showed the liver protective activity in vivo, the protective effect of the T. sarmentosa extract was further tested in cultured clone-9 hepatocytes (CitationSahu et al., 2008). Clone-9 cells were stimulated with APAP at different doses (0−20 mM) for 24 h. Viability of cells was analyzed by MTT assays. Cells treated with APAP at different concentrations for 24 h showed significantly decreased viability when compared with controls (). When hepatocytes were pretreated with different concentrations of T. sarmentosa for 1 h before the addition of APAP (10 mM), cell viability was increased in a dose-dependent manner ().
Figure 1. (A) Dose-dependent effect of acetaminophen (APAP) on clone-9 hepatocyte viability. Results have been given as percentage over control (CL). Cells were kept as CL or treated with different concentrations (0−20 mM) of APAP for 24 h. The cell viability was detected by MTT assay. Each bar represents the mean ± standard error of the mean (SEM) from six independent experiments. *p < 0.05 versus CL. (B) Dose-dependent effect of the aqueous extract of T. sarmentosa against APAP-induced toxic effect on cell viability. Cells were kept as CL or stimulated with 10 mM APAP for 24 h. Before stimulated with APAP, clone-9 cells were pretreated with 0−100 μg/mL aqueous extract of T. sarmentosa for 1 h. The cell viability was detected by MTT assay. Each bar represents the mean ± SEM from six independent experiments. *p < 0.05 versus CL. #p < 0.05 versus APAP-treated cells.
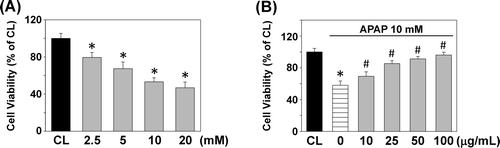
Effect of T. sarmentosa extract on APAP-induced lipid peroxidation in clone-9 cells
Lipid peroxidation, measured as MDA level, is shown in . APAP administration increased the lipid peroxidation to 212.6% compared with the normal cells. T. sarmentosa water extract treatment prior to APAP stimulation caused inhibition in the lipid peroxidation in a dose-dependent manner.
Figure 2. Effect of T. sarmentosa on malondialdehyde (MDA) level in clone-9 cells treated with acetaminophen (APAP). Results have been given as percentage over control (CL). Cells were kept as CL or treated with 10 mM APAP. Before stimulation with acetaminophen (APAP), clone-9 cells were pretreated with 0−100 μg/mL aqueous extract of T. sarmentosa for 1 h. Each bar represents the mean ± standard error of the mean (SEM) from six independent experiments. *p < 0.05 versus CL. #p < 0.05 versus APAP-treated cells.
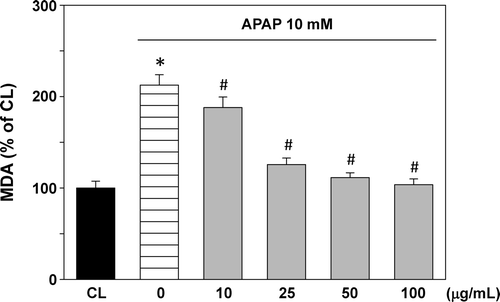
Effect of T. sarmentosa extract on APAP-induced oxidative stress in clone-9 cells
Changes in GSH content are shown in . APAP treatment decreased GSH level to about 58.4%. T. sarmentosa water extract treatment prior to APAP stimulation increased the GSH level in a dose-dependent manner. The GSH level was recovered almost to its normal level at the T. sarmentosa concentration of 100 μg/mL.
Figure 3. Effect of T. sarmentosa on glutathione (GSH) level in clone-9 cells treated with acetaminophen (APAP). Results have been given as percentage over control (CL). Cells were kept as CL or treated with 10 mM APAP. Before stimulation with APAP, clone-9 cells were pretreated with 0−100 μg/mL aqueous extract of T. sarmentosa for 1 h. Each bar represents the mean ± standard error of the mean (SEM) from six independent experiments. *p < 0.05 versus CL. #p < 0.05 versus APAP-treated cells.
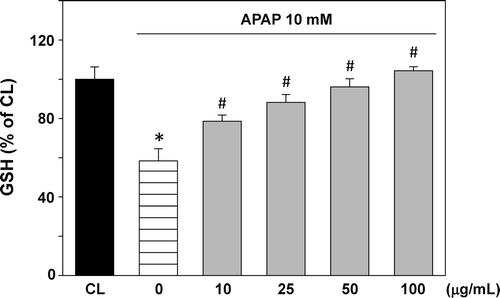
Effect of T. sarmentosa extract on APAP-induced antioxidant enzyme activity in clone-9 cells
The antioxidant enzyme activities are shown in . APAP treatment decreased the CAT activity by about 61.3%. Significant increase in CAT activity was observed at a T. sarmentosa concentration 10 μg/mL, and at the concentration 100 μg/mL, the CAT activity was reached to almost normal (). and demonstrate the effect of the T. sarmentosa on SOD and GPx activities, respectively. The results are similar to CAT activity.
Figure 4. Effect of T. sarmentosa on (A) catalase (CAT), (B) superoxide dismutase (SOD), and (C) glutathione peroxidase (GPx) activity in clone-9 cells treated with acetaminophen (APAP). Results have been given as percentage over control (CL). Cells were kept as CL or treated with 10 mM APAP. Before stimulated with APAP, clone-9 cells were pretreated with 0−100 μg/mL aqueous extract of T. sarmentosa for 1 h. Each bar represents the mean ± standard error of the mean (SEM) from six independent experiments. *p < 0.05 versus CL. #p < 0.05 versus APAP-treated cells.
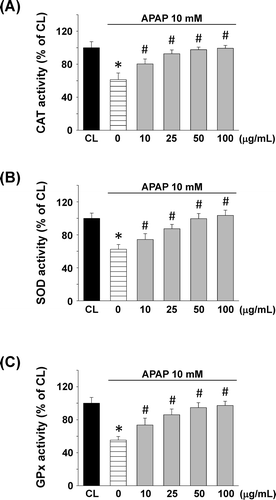
Discussion
APAP is a widely used analgesic and antipyretic. When taken in high doses, it becomes a potent hepatotoxin and can cause fatal hepatic necrosis (CitationChun et al., 2009; CitationLarson et al., 2005). In this study, the aqueous extract of T. sarmentosa was observed to exhibit hepatoprotective effect as demonstrated by a significant decrease in SGOT, SGPT, and ALP concentrations in rats administrated with APAP. The aqueous extract of T. sarmentosa also increased the activities of antioxidant enzymes and diminished the amount of lipid peroxidation in liver homogenate of APAP-treated rats. In addition, we used rat clone-9 hepatocytes for evaluation of APAP cytotoxicity. Clone-9 cells were treated with various concentrations of APAP for different time periods and the changes in viability were measured. Results obtained by MTT assay showed a decline in cell viability detectable at 12 and 24 h of incubation. Moreover, the study also demonstrated that APAP causes toxic effects on clone-9 cells. Our study showed that there was a higher MDA level in APAP-treated clone-9 cells in comparison with the control cells. In addition, our findings indicated that APAP stimulation caused depletion of the GSH level and the antioxidant enzymes, including CAT, SOD, and GPx. The aqueous extract of T. sarmentosa was observed to exhibit hepatoprotective effect as demonstrated by significant increase in cell viability, GSH level, and antioxidant enzyme activities, and decrease in MDA level in APAP-treated clone-9 cells.
Several studies have been revealed that serum levels of liver function markers SGOT, SGPT, and ALP prominently increase are remarkable feature of APAP overdose (CitationAjith et al., 2007; CitationChandrasekaran et al., 2008; CitationFakurazi et al., 2008). In this study, the serum levels of SGOT, SGPT, and ALP were augmented in the APAP-intoxicated rats. The aqueous extract of T. sarmentosa with concentrations of 250 and 500 mg/kg could decrease the SGOT, SGPT, and ALP in these APAP-treated animals. These results suggested that the inhibition of liver function markers elevation may play a significant role in the protective effect of the T. sarmentosa against APAP-induced hepatotoxicity. In addition, the mechanism responsible for the inhibition of APAP hepatotoxicity may involve immunologic factors, since T. sarmentosa decreases serum levels of inflammatory mediators TNF-α, IL-1β, and IL-6. The major source of these cytokines production in liver is believed to be the Kupffer cells, which are central to hepatic inflammatory processes (CitationAndrés et al., 2003; CitationDing et al., 2003). The toxic injury can be driven via activation of Kupffer cells and adjacent sinusoidal endothelial cells; these in turn release inflammatory mediators (CitationLacour et al., 2005). Our results in this study showed that the serum levels of TNF-α, IL-1β, and IL-6 following APAP administration were elevated in rats, suggesting that cytokines release may also play a crucial role in the induction of hepatic damage by APAP in the animal model used. Treatment of T. sarmentosa could protect against APAP-induced elevation of TNF-α, IL-1β, and IL-6. These results suggested that T. sarmentosa may also have anti-inflammatory effect on rats intoxicated with APAP.
The aqueous ethanol extract of T. sarmentosa has been reported to possess antilipid peroxidative bioactivity in vitro (CitationLin et al., 2002). The ameliorative action of the T. sarmentosa was probably related to this antioxidant action. Liver damage is always associated with cellular necrosis, increase in tissue peroxidation, and depletion in the tissue GSH levels. The MDA level is a critical indicator of lipid peroxidation. The level of MDA was increased in the rat liver homogenate and clone-9 cells receiving APAP stimulation, but T. sarmentosa pretreatment showed effective decrease of MDA contents. These data suggested that T. sarmentosa might have a protective effect against the APAP-induced membrane damages. Moreover, APAP in high doses can cause hepatotoxicity by formation of NAPQI, a toxic quinine metabolite, which is produced by the cytochrome P4-50 enzymes (CitationJames et al., 2003). NAPQI is detoxified in a reaction with GSH leading to depletion of the protective thiol, resulting in no hepatic damage. Hepatic necrosis occurs when the NAPQI produced is enhanced by APAP that cause GSH depletion, or decrease in the antioxidative activity of the liver. Therefore, our results suggested that the hepatoprotective effects afforded by the aqueous extract of T. sarmentosa may be in part attributed to these factors. It has been shown that oxidative stress is a major mechanism in the APAP-induced hepatotoxicity (CitationBajt et al., 2004; CitationHinson et al., 2004). Our data suggested that APAP stimulation lead to decreased levels of CAT, SOD, and GPx activity. However, the T. sarmentosa extract could recover the levels of these antioxidant enzymes. Therefore, it could be said that APAP caused the liver damage by suppressing the activity of these antioxidant enzymes and that could be prevented by the T. sarmentosa pretreatment.
In conclusion, the protective activity of the aqueous extract of T. sarmentosa against APAP-induced injury in rat and hepatocytes may be due to its antioxidative and anti-inflammatory properties. Further safety and efficacy studies are needed to elucidate its mechanism of action in detail.
Declaration of interest
This study was supported by grants Armed-Forces Taichung General Hospital (AFTGH) 9824, CMRPF680051, and EZRPF390261 from AFTGH, and AFHLGH 805-C100-08 from Armed-Forces Hailien General Hospital, Taiwan.
References
- Ajith TA, Hema U, Aswathy MS. (2007). Zingiber officinale Roscoe prevents acetaminophen-induced acute hepatotoxicity by enhancing hepatic antioxidant status. Food Chem Toxicol, 45, 2267–2272.
- Andrés D, Sánchez-Reus I, Bautista M, Cascales M. (2003). Depletion of Kupffer cell function by gadolinium chloride attenuates thioacetamide-induced hepatotoxicity. Expression of metallothionein and HSP70. Biochem Pharmacol, 66, 917–926.
- Bajt ML, Knight TR, Lemasters JJ, Jaeschke H. (2004). Acetaminophen-induced oxidant stress and cell injury in cultured mouse hepatocytes: protection by N-acetyl cysteine. Toxicol Sci, 80, 343–349.
- Blazka ME, Wilmer JL, Holladay SD, Wilson RE, Luster MI. (1995). Role of proinflammatory cytokines in acetaminophen hepatotoxicity. Toxicol Appl Pharmacol, 133, 43–52.
- Bourdi M, Masubuchi Y, Reilly TP, Amouzadeh HR, Martin JL, George JW, Shah AG, Pohl LR. (2002). Protection against acetaminophen-induced liver injury and lethality by interleukin 10: role of inducible nitric oxide synthase. Hepatology, 35, 289–298.
- Chiu NY, Chang KH. (1987). The illustrated medicinal plants of Taiwan: SMC publishing: Taipei, Taiwan. Vol. 2, p 184.
- Chandrasekaran VR, Wan CH, Liu LL, Hsu DZ, Liu MY. (2008). Effect of sesame oil against acetaminophen-induced acute oxidative hepatic damage in rats. Shock, 30, 217–221.
- Chu SC, Yang SF, Liu SJ, Kuo WH, Chang YZ, Hsieh YS. (2007). In vitro and in vivo antimetastatic effects of Terminalia catappa L. leaves on lung cancer cells. Food Chem Toxicol, 45, 1194–1201.
- Chun LJ, Tong MJ, Busuttil RW, Hiatt JR. (2009). Acetaminophen hepatotoxicity and acute liver failure. J Clin Gastroenterol, 43, 342–349.
- Josephy PD. (2005). The molecular toxicology of acetaminophen. Drug Metab Rev, 37, 581–594.
- Ding H, Peng R, Reed E, Li QQ. (2003). Effects of Kupffer cell inhibition on liver function and hepatocellular activity in mice. Int J Mol Med, 12, 549–557.
- Fakurazi S, Hairuszah I, Nanthini U. (2008). Moringa oleifera Lam prevents acetaminophen induced liver injury through restoration of glutathione level. Food Chem Toxicol, 46, 2611–2615.
- Geiger TL, Howard SC. (2007). Acetaminophen and diphenhydramine premedication for allergic and febrile nonhemolytic transfusion reactions: good prophylaxis or bad practice? Transfus Med Rev, 21, 1–12.
- Hinson JA, Reid AB, McCullough SS, James LP. (2004). Acetaminophen-induced hepatotoxicity: role of metabolic activation, reactive oxygen/nitrogen species, and mitochondrial permeability transition. Drug Metab Rev, 36, 805–822.
- Jaeschke H. (2005). Role of inflammation in the mechanism of acetaminophen-induced hepatotoxicity. Expert Opin Drug Metab Toxicol, 1, 389–397.
- James LP, Mayeux PR, Hinson JA. (2003). Acetaminophen-induced hepatotoxicity. Drug Metab Dispos, 31, 1499–1506.
- James LP, McCullough SS, Lamps LW, Hinson JA. (2003). Effect of N-acetylcysteine on acetaminophen toxicity in mice: relationship to reactive nitrogen and cytokine formation. Toxicol Sci, 75, 458–467.
- Lacour S, Gautier JC, Pallardy M, Roberts R. (2005). Cytokines as potential biomarkers of liver toxicity. Cancer Biomark, 1, 29–39.
- Larson AM, Polson J, Fontana RJ, Davern TJ, Lalani E, Hynan LS, Reisch JS, Schiødt FV, Ostapowicz G, Shakil AO, Lee WM; Acute Liver Failure Study Group. (2005). Acetaminophen-induced acute liver failure: results of a United States multicenter, prospective study. Hepatology, 42, 1364–1372.
- Lee SD, Chen LM, Kuo WW, Shu WT, Kuo WH, Huang EJ, Tsai CC, Li PC, Liu JY, Chen TH, Huang CY. (2006). Serum insulin-like growth factor-axis and matrix metalloproteinases in patients with rheumatic arthritis or rheumatic heart disease. Clin Chim Acta, 367, 62–68.
- Lin YL, Tsai YL, Kuo YH, Liu YH, Shiao MS. (1999). Phenolic compounds from tournefortia sarmentosa. J Nat Prod, 62, 1500–1503.
- Lin YL, Chang YY, Kuo YH, Shiao MS. (2002). Anti-lipid-peroxidative principles from Tournefortia sarmentosa. J Nat Prod, 65, 745–747.
- Sahu SC, Garthoff LH, Robl MG, Chirtel SJ, Ruggles DI, Flynn TJ, Sobotka TJ. (2008). Rat liver clone-9 cells in culture as a model for screening hepatotoxic potential of food-related products: hepatotoxicity of deoxynivalenol. J Appl Toxicol, 28, 765–772.