Abstract
Context: Baicalin has been characterized as the active compound and quality control marker in Scutellaria baicalensis Georgi, traditionally used as a hypotensive herb.
Objectives: To investigate the inhibitory activities of baicalin against renin and angiotensin-I converting enzyme (ACE) and their molecule mechanism of interactions.
Methods: The fluorescence method using renin substrate 1(R-2932) and the spectroscopy method by Cushman were used to determine renin and ACE activities, respectively. The fluorescence quench techniques were used to characterize their interactions.
Results: The results showed that baicalin inhibited renin activity with an IC50 value of 120.36 µM and inhibited ACE activity with an IC50 value of 2.24 mM in vitro. The fluorescence emission of both renin and ACE were efficiently quenched by baicalin and a complete quenching was achieved at a high concentration of baicalin. Furthermore, baicalin was more effective in quenching the fluorescence of renin (KSV = 60 × 103 M−1) than ACE (KSV = 17.1 × 103 M−1). The quenching of fluorescence of renin and ACE involved static interactions, which was characterized by the formation of quencher–enzyme complex. The baicalin–renin complex formed through three-sites binding including the active site with a binding constant of 796.15 × 1013 M−1, but there was only one binding site for the baicalin–ACE complex with a much smaller binding constant of 6.8 × 105 M−1.
Conclusion: The inhibition activity of baicalin against renin was a result of the formation of stable complex through multisites binding including the active site, which could explain the higher inhibitory efficiency.
Introduction
Renin–angiotensin system (RAS) plays a crucial role in the development of cardiovascular diseases such as congestive heart failure and hypertension (CitationBrunner et al., 1972; CitationRemkova & Remko, 2010; CitationShah et al., 2010). Renin and angiotensin-I-converting enzyme (ACE) are two key enzymes responsible for maintaining the operation of RAS. Renin specifically converts angiotensinogen to angiotensin I (Ang I) and then angiotensin I (AngI) is broken down by ACE to release angiotensin II, which had been identified as vasoconstrictor, a major factor in the pathogenesis of hypertension (CitationPeach, 1977). Thus, inhibition of RAS has become the primary means of treatment of cardiovascular diseases, especially hypertension (CitationZaman et al., 2002). Currently, ACE inhibitors are the most commonly used agents for the clinical management of hypertension (CitationBlack & Wrapson, 2001). However, certain side effects such as dry cough and allergies (CitationMorice et al., 1989; CitationIsraili & Hall, 1992; CitationGraves, 2002) that are associated with the use of ACE inhibitors are thought to be unavoidable since ACE can also hydrolyze bradykinin, a known vasodilator. Moreover, angiotensin II formation by the action of chymase could render ineffective certain ACE inhibitors. Therefore, there is an increasing interest in the search and development of natural ACE and renin inhibitors that would be effective but produce fewer side effects. Theoretically, renin inhibitors are considered more selective and provide an improved side effect profile because the naturally occurring substrate for renin is only angiotensinogen (CitationLynch &Peach, 1991). Thus, renin inhibitors have continued to draw great attention (CitationMaibaum & Feldman, 2003; CitationStanton, 2003). Although much effort had been paid to the design of renin inhibitors, very few orally active agents have been found since the early renin inhibitors were analogs of angiotensinogen (CitationCumin et al., 1985). These renin inhibitors were not effective as clinical tools because of easy degradation, low bioavailability, and the difficulty of large-scale production. However, in 2003, a nonpeptide small molecule compound, aliskiren (IC50 = 0.6 nM), with highly inhibitory activity against renin, was discovered via molecular design and pioneered a new field for studying renin inhibitors (CitationStanton et al., 2003; CitationWood et al., 2003). Despite the great devotions to renin inhibitors, little attention has been paid to natural products, especially the compounds used in traditional Chinese medicine such as baicalin and baicalien (CitationHuang et al., 2005) isolated from Scutellaria baicalensis Georgi, a traditional Chinese medicine (CitationChina Pharmacopoeia Committee, 2010). This work aims at the renin and ACE inhibitory activities of baicalin (7-glucuronic acid-5,6-dihydroxyl-flavone, C21H18O11, MW: 446.38), which is the quality control marker of this herb. The molecular mechanism of interactions was established as well, which could provide a structural basis for the future development of potent renin inhibitors.
Methods
Chemicals and reagents
Baicalin with at least 95% purity was purchased from the Chinese National Institute for the Control of Pharmaceutical and Biological Products (NICPBP). Renin (human recombinant), purity ≥99% by SDS-PAGE, was purchased from Cayman Chemical Company Molecular Probes Inc. (4849 Pitchford Ave.Eugene, OR 97405-9165). Renin substrate 1(R-2932), (Arg-Glu(EDANS)−lle-His-Pro-Phe-His-Leu-Val-lle-His-Thr-Lys(dabcyl)-Arg) was purchased from Molecular Probes Inc. Cayman Chemical Company (1180 East Ellsworth Road, Ann Arbor, Michigan 48108, USA). ACE, HHL (Hippuryo-histidyl-leucine), and Ang I (synthetic human angiotensin I) were from Sigma Chemicals (St. Louis, MO, USA). Other chemicals used in this study were at least of analytical grade. The pure water was generated by Milli-Q system (Millipore, Bedford, MA, USA). A JASCO FP-6300 spectrofluorometer (JASCO Corp., Tokyo, Japan) equipped with a thermostated micro-cell holder was used.
Assay for renin and ACE inhibitory activities
The inhibitory activity against renin was assayed by a fluorescence method (CitationWang et al., 1993). Renin (500 μM) was diluted 10–30 times with 50 mM Tris buffer (pH 8.0, containing 100 mM NaCl). The reaction mixture consisted of 80 µL renin substrate (5 µM), 20 µL Tris buffer (no inhibition) or baicalin in varying concentrations. The mixture was incubated at 37°C for 2 min to reach a thermal equilibrium. The reaction was then initiated by the addition of 2 µL of 1:10 diluted renin solution and the time-dependent increase in fluorescence intensity was monitored for 5–10 min. The initial reaction velocity, expressed in arbitrary fluorescence units per minute (INT/min), was obtained by least-square regression analysis of the initial phase of the reaction. The concentration of inhibitors, which inhibited 50% of activity, was defined as the IC50 value. To determine IC50, the inhibitory activities were assayed under a series of concentrations of baicalin, with constant concentrations of enzyme and substrate. The IC50 values were obtained by plotting percentage enzyme inhibition versus the concentrations of inhibitors, using nonlinear regression analysis. Instrument parameters: excitation wavelength at 340 nm and emission wavelength at 490 nm; excitation slit 5 nm, and emission slit 10 nm.
The ACE inhibitory activity was determined using the method of Cushman with modifications (CitationCushman & Cheung, 1971). An aliquot of 150-μL HHL (5 mM in 50 mM sodium borate buffer containing 0.5 M NaCl at pH 8.3) and 25 μL baicalin solution (inhibition test) or 25 μL borate buffer (without inhibition) were incubated at 37°C for 5 min, followed by the addition of 25 μL ACE solution (25 mU/mL). The reaction mixture was maintained at 37°C for 40 min, terminated by the addition of 350 μL HCl (1 M). Ethyl acetate (1.5 mL) was added to the reaction mixture and vortexed for 1 min for the extraction of hippuric acid produced by ACE. After centrifugation at 10,000g for 5 min, 1 mL upper layer (acetic ester) was transferred into a test tube and the acetic ester was evaporated by heating at 120°C for 30 min. The obtained residue was then dissolved in 1-mL distilled water and the absorbance was determined at 228 nm using water as blank. The inhibition percentage (%) was calculated as IP (%) = [1−(Ai/Aj)] × 100%, where A. represents the absorption of the test with baicalin, and Aj represents the absorption of the test without baicalin. The IC50 value was calculated with nonlinear regression from a plot of percentage inhibition against different concentration of baicalin.
Interactions between baicalin and renin
The interactions between baicalin and renin were studied by fluorescence quenching techniques. The baicalin solutions at different concentrations were prepared by diluting the stock solution with Tris buffer. A total volume of 50 μL reaction mixture containing 46 μL Tris buffer, 2 μL baicalin at varying concentrations (or 2 μL of tris-buffer for the control) and 2 μL renin (40 µg/mL) was excited at 280 nm and 37°C in each assay. The changes in the fluorescence intensity were measured at maximum emission wavelength of renin 3 min after the addition of baicalin to the renin solution. Each assay was repeated in triplicate, and the mean and standard deviation were calculated. The emission spectra of renin were recorded to allow the observation of any changes in the renin spectra in the presence of baicalin.
Interactions between baicalin and ACE
ACE and baicalin solution were prepared in borate buffer. The reaction mixture containing 30 μL ACE (3mU), 30 μL baicalin at various concentrations, and 10 μL Ang I (or 10-μL borate buffer for the control) was excited at 295 nm and kept at 37°C, and the change in fluorescence intensity was measured at maximum emission wavelength of ACE 3 min after the addition of baicalin to the ACE solution. The emission spectra of ACE were recorded to allow the observation of any changes in the ACE spectra in the presence of baicalin.
Determination of fluorescence quenching constants
The fluorescence quenching data were plotted as Stern−Volmer equation (Eq.1) and modified Stern−Volmer equation (Eq.2).
Where ksv is the Stern−Volmer quenching constant with the unit of L mol−1, [Q] is the concentration of the quencher, kq is the rate quenching constant of the biological macromolecule, and τ0 is the average lifetime of the molecule without any quencher and the fluorescence lifetime of the biopolymer is 10−8 s. Kb is the binding constant, and n is the number of binding sites per macromolecule (CitationBlatt et al., 1986; CitationEpps et al., 1999).
Statistical analysis
Statistical analysis was performed by the Duncan Multiple Range Test using a computer software package (SAS, Version 6.12, Cary, NC, USA). Significant difference was taken as p < 0.05.
Results
Renin and ACE inhibitory activities of baicalin
The inhibitory activities of baicalin against renin are shown in . The change in fluorescence intensity as a function of time was a horizontal line before 160 sec and then the line was steadily upward when the renin was added. The addition of baicalin slowed down the increase of fluorescence intensity when compared with the addition of buffer. The observed decreased rates of fluorescence intensity indicated that the activity of renin was inhibited by baicalin. Furthermore, the plots of the percentage inhibition against the concentration of inhibitors () showed that the inhibitory activity was dose-dependent. The calculated IC50 value for baicalin was 120.36 µM.
Figure 1. Renin inhibitory phenomena of baicalin (0.1 mg/mL) observed by fluorescence spectrometry assay.
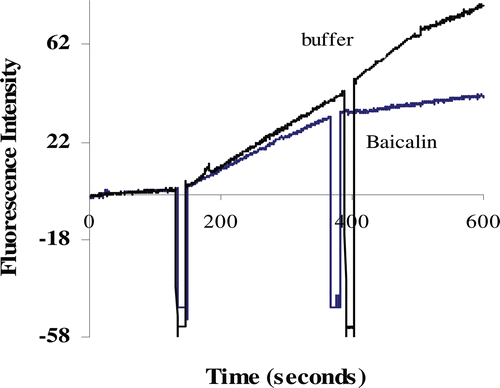
Figure 2. Plot of percentage inhibition of renin activity versus concentration of baicalin. The IC50 for baicalin is 120.36 µM.
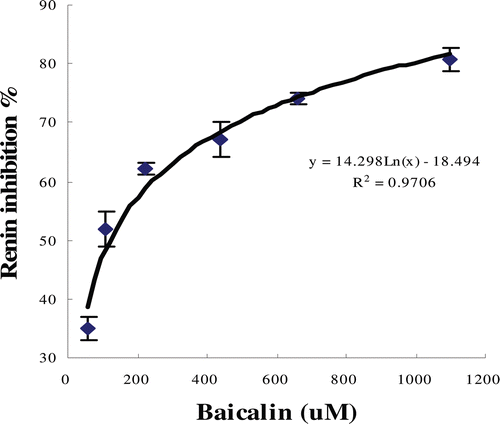
ACE inhibitory activity as determined by the UV spectrometry method showed that baicalin exhibited weak inhibition of ACE with IC50 value at 2.24 mM compared with the inhibition of renin activity.
Effects of baicalin on fluorescence emission spectrum of renin
shows the fluorescence emission spectra of renin obtained at 37°C and pH 8.0 with baicalin as quenchers. The maximum emission wavelength for renin is 329 nm. No significant shift in the maximum emission wavelength (only increased 3 nm) of renin was observed in the presence of baicalin. The emission fluorescence intensity of renin decreased with the addition of baicalin in a dose-dependent manner, and the width of emission spectrum was only 50 nm.
Effects of baicalin on fluorescence emission spectrum of ACE
The quench effect of baicalin on ACE fluorescence was determined in the presence or absence of Ang I, respectively, and the results were about same as shown in and . The maximum emission wavelength of ACE at 340 nm was obtained. The fluorescence intensity at 340 nm decreased with the increased concentration of baicalin. A new peak at 449 nm was found and its intensity increased with the increasing concentration of baicalin. The width of the emission spectrum of ACE was around 150 nm, much larger than that of the emission spectrum of renin.
Fluorescence quenching constants and binding parameters
From and , complete quench of renin fluorescence was achieved at 8.8 μM of baicalin, and for the total quench of ACE fluorescence, the concentration of baicalin was 250 μM.
The fluorescence quench constants, binding constants, and number of binding sites were calculated by Stern−Volmer plot and modified Stern−Volmer plot, to investigate the mechanisms for the quench of both renin and ACE fluorescence.
Stern−Volmer plot of both the renin and ACE fluorescence quench by baicalin yielded nonlinear equations with low R2 value of about 0.95 (). The calculated Ksv for renin and ACE fluorescence quench were 60 × 103 M−1 and 17.1 × 103 M−1, respectively, indicating that baicalin was more effective in renin fluorescence quenching than ACE fluorescence quenching. The quench efficiency was in agreement with the enzyme inhibitory potency. And the calculated Kq were 6 × 1012 M−1S−1 and 1.71 × 1012 M−1S−1 for renin and ACE fluorescence quench, respectively, which are 100-times higher than the maximum value possible for diffusion-limited quenching in water (2 × 1010 M−1S−1).
Table 1. Stern−Volmer constant and coefficient for the interaction of baicalin with renin and angiotensin-I converting enzyme (ACE).
The binding constants, number of binding sites, and Gibbs free energy were calculated from modified Stern−Volmer plot and are shown in . The binding constant of baicalin–renin complex was 796.15 × 1013, and the binding constant of baicalin–ACE complex was only 6.8 × 105 M−1. There were three sites or regions in renin molecule for baicalin to bind and one site or regions in ACE molecule for baicalin to bind. Moreover, the ΔG value for renin–baicalin interaction was −94.38 KJ/M and for ACE–baicalin interaction was −34.64 KJ/M.
Table 2. The binding constants, number of binding sites of complex, and Gibbs free energy for the interaction of baicalin with renin and angiotensin-I-converting enzyme (ACE).
Discussion
Baicalin (7-glucuronic acid-5, 6-dihydroxy-flavone, C21H18O11, MW: 446.38), the main ingredient in the commonly used traditional Chinese medicine Scutellariae radix, has various pharmaceutical effects such as anti-inflammatory, antiviral, free radical scavenging, antioxidant, and anticancer properties (CitationLin & Shieh, 1996). In addition, the vasodepressor effect of baicalin has been previously reported in rats (CitationTakizawa et al., 1998). The results of this study revealed that baicalin is more potent in renin inhibition than ACE inhibition, similar to the activity of sodium houttuyfonate analogs (CitationYuan et al., 2006), suggesting that baicalin, formerly an antimicrobial and antiviral agent, may interfere the RAS in vivo through renin and ACE inhibitions.
Fluorescence quenching refers to any processes that decrease the fluorescence intensity of a given substance, and fluorescence study provides useful information that could reveal the types of interactions between a fluorophore and quencher molecule. A total quench of renin and ACE fluorescence by baicalin provided the evidence that the molecule of baicalin interacted close enough to tryptophan (Trp) and tyrosine (Tyr) in renin molecule and Trp in ACE molecule. Since no significant change in the maximum emission wavelength was observed, it suggested that minimal structural change in the renin protein but rather specific interactions between the inhibitors and fluorophore molecules.
The narrow fluorescence emission range indicated that renin and the aqueous environment had a limited interaction, which means the limited degree of unfolding of the enzyme protein structure. Thus, it was difficult for baicalin to cause extensive unfolding of renin protein at the concentrations used in this study. However, the larger fluorescence emission range for ACE suggested that ACE has a more open or expanded structure, hence greater interaction with aqueous environment compared with the structure of renin.
The emission spectrum for ACE in the presence and absence of Ang I were nearly identical in its fluorescence quench process by baicalin suggesting that Ang I had no any effect on the emission spectrum as well as the quench efficiency. Also, the results suggested that baicalin did not interact with active site in the process of quenching the intrinsic fluorescence of ACE. This could explain the weak inhibitory effect of baicalin on ACE activity since the active site seems to be accessible to substrate even in the presence of the inhibitor molecules.
Normally two quench processes, static and dynamic quench, are involved in the fluorescence quench mechanism. In dynamic quenching, the excited fluorophore experiences contact with an atom or molecule that can facilitate nonradiative transitions to ground state, and static quenching involves the formation of a stable nonfluorescent complex of fluorophore−quencher (CitationBlatt et al., 1986). The rate quench constant Kq for both renin and ACE intrinsic fluorescence quench suggested that baicalin specially interacted with enzyme proteins, forming the protein−quencher complexes. Similar results were found for the interactions between sodium houttuyfonate analogs and renin (CitationYuan et al., 2007). The second emission peak at 449 nm in ACE emission spectrum seen in was also an important indication for the formation of ACE−baicalin complex.
A much larger binding constant for baicalin binding to renin suggests that the baicalin–renin complex is more stable than the baicalin–ACE complex, which could explain the stronger inhibitory activity of baicalin against renin than ACE. The negative value of ΔG0 indicated that both of the two reactions were spontaneous and the formation of baicalin–renin complex was easier than that of the baicalin–ACE complex under the experimental conditions.
Renin contains three Trp residues and several Tyr residues that are buried deeply in the hydrophobic interior at different domains within the molecule and one of Trp residues is in the region of the active site (CitationQuay et al., 1985). The excitation wavelength of 280 nm used for renin intrinsic fluorescence assays provides information on the location and accessibility of Trp and Tyr residues. Our results indicate that all the fluorophores including the active site were accessible to baicalin with different affinities as evident from the complete quench achieved and a nonlinear curve of Stern−Volmer plot (not shown). Investigation of the crystal structure of human renin revealed that it is composed of two β-sheet domains with a cleft between them. Studies of the conformational structure of the cleft showed that the S3−S1 subsites form a hydrophobic pocket that constitutes a large hydrophobic cavity (CitationSibanda et al., 1984). A previous finding observed optimal renin inhibition when the S3 subsite is occupied (CitationWood et al., 2003; CitationSarver et al., 2007). It was not confirmed that the renin inhibitory activity of baicalin was the result of S3 site binding in this study, but the formation of the complex and the elucidation of the three binding sites indicate that baicalin entered into hydrophobic pocket within the renin protein. Overall, the results suggest that future structural modification of baicalin to increase surface hydrophobicity may enhance its inhibitory activity against renin.
Conclusion
The inhibition activity of baicalin against renin was a result of the formation of stable complex through multisites binding including the active site, which could explain the higher inhibitory efficiency.
Acknowledgments
This work was supported by the National Natural Science Foundation of China (20673084), the Natural Science Foundation Project of CQ CSTC (CSTC, 2007BB0345), and the Natural Sciences and Engineering Research Council of Canada (NSERC).
Declaration of interest
The authors report no conflicts of interest. The authors alone are responsible for the content and writing of the paper.
References
- Black P, Wrapson C. (2001). ACE inhibitors for hypertension. N Z Med J, 114, 20–21.
- Blatt E, Chatelier RC, Sawyer WH. (1986). Effects of quenching mechanism and type of quencher association on Stern-Volmer plots in compartmentalized systems. Biophys J, 50, 349–356.
- Brunner HR, Laragh JH, Baer L, Newton MA, Goodwin FT, Krakoff LR, Bard RH, Bühler FR. (1972). Essential hypertension: Renin and aldosterone, heart attack and stroke. N Engl J Med, 286, 441–449.
- China Pharmacopoeia Committee, Pharmacopoeia of the People’s Republic of China, the first division of 2010 ed., China Chemical Industry Press, Beijing, 1999, p. 282–283.
- Cumin F, Evin G, Fehrentz JA, Seyer R, Castro B, Menard J, Corvol P. (1985). Inhibition of human renin by synthetic peptides derived from its prosegment. J Biol Chem, 260, 9154–9157.
- Cushman DW, Cheung HS. (1971). Spectrophotometric assay and properties of the angiotensin-converting enzyme of rabbit lung. Biochem Pharmacol, 20, 1637–1648.
- Epps DE, Raub TJ, Caiolfa V, Chiari A, Zamai M. (1999). Determination of the affinity of drugs toward serum albumin by measurement of the quenching of the intrinsic tryptophan fluorescence of the protein. J Pharm Pharmacol, 51, 41–48.
- Graves JW. (2002). Additional side effects of ACE inhibitors. Am Fam Physician, 66, 2048.
- Huang Y, Tsang SY, Yao X, Chen ZY. (2005). Biological properties of baicalein in cardiovascular system. Curr Drug Targets Cardiovasc Haematol Disord, 5, 177–184.
- Israili ZH, Hall WD. (1992). Cough and angioneurotic edema associated with angiotensin-converting enzyme inhibitor therapy. A review of the literature and pathophysiology. Ann Intern Med, 117, 234–242.
- Lin CC, Shieh DE. (1996). The anti-inflammatory activity of Scutellaria rivularis extracts and its active components, baicalin, baicalein and wogonin. Am J Chin Med, 24, 31–36.
- Lynch KR, Peach MJ. (1991). Molecular biology of angiotensinogen. Hypertension, 17, 263–269.
- Maibaum J, Feldman DL. (2003). Renin inhibitors as novel treatments for cardiovascular disease. Expert Opin Ther Pat,13, 589–603.
- Morice AH, Brown MJ, Higenbottam T. (1989). Cough associated with angiotensin converting enzyme inhibition. J Cardiovasc Pharmacol, 13 Suppl 3, S59–S62.
- Peach MJ. (1977). Renin-angiotensin system: Biochemistry and mechanisms of action. Physiol Rev, 57, 313–370.
- Quay SC, Heropoulos A, Commes K, Dzau VJ. (1985). Probing the renin active site by collisional quenching of endogenous fluorescence. J Biol Chem, 260, 15055–15058.
- Remková A, Remko M. (2010). The role of renin-angiotensin system in prothrombotic state in essential hypertension. Physiol Res, 59, 13–23.
- Sarver RW, Peevers J, Cody WL, Ciske FL, Dyer J, Emerson SD, Hagadorn JC, Holsworth DD, Jalaie M, Kaufman M, Mastronardi M, McConnell P, Powell NA, Quin J 3rd, Van Huis CA, Zhang E, Mochalkin I. (2007). Binding thermodynamics of substituted diaminopyrimidine renin inhibitors. Anal Biochem, 360, 30–40.
- Shah RV, Desai AS, Givertz MM. (2010). The effect of renin-angiotensin system inhibitors on mortality and heart failure hospitalization in patients with heart failure and preserved ejection fraction: A systematic review and meta-analysis. J Card Fail, 16, 260–267.
- Sibanda BL, Blundell T, Hobart PM, Fogliano M, Bindra JS, Dominy BW, Chirgwin JM. (1984). Computer graphics modelling of human renin. Specificity, catalytic activity and intron-exon junctions. FEBS Lett, 174, 102–111.
- Stanton A. (2003). Potential of renin inhibition in cardiovascular disease. J Renin Angiotensin Aldosterone Syst, 4, 6–10.
- Stanton A, Jensen C, Nussberger J, O’Brien E. (2003). Blood pressure lowering in essential hypertension with an oral renin inhibitor, aliskiren. Hypertension, 42, 1137–1143.
- Takizawa H, DelliPizzi AM, Nasjletti A. (1998). Prostaglandin I2 contributes to the vasodepressor effect of baicalein in hypertensive rats. Hypertension, 31, 866–871.
- Wang GT, Chung CC, Holzman TF, Krafft GA. (1993). A continuous fluorescence assay of renin activity. Anal Biochem, 210, 351–359.
- Wood JM, Maibaum J, Rahuel J, Grütter MG, Cohen NC, Rasetti V, Rüger H, Göschke R, Stutz S, Fuhrer W, Schilling W, Rigollier P, Yamaguchi Y, Cumin F, Baum HP, Schnell CR, Herold P, Mah R, Jensen C, O’Brien E, Stanton A, Bedigian MP. (2003). Structure-based design of aliskiren, a novel orally effective renin inhibitor. Biochem Biophys Res Commun, 308, 698–705.
- Yuan L, Wu J, Aluko RE, Ye X. (2006). Kinetics of renin inhibition by sodium houttuyfonate analogs. Biosci Biotechnol Biochem, 70, 2275–2280.
- Yuan L, Wu J, Aluko RE. (2007). Size of the aliphatic chain of sodium houttuyfonate analogs determines their affinity for renin and angiotensin I converting enzyme. Int J Biol Macromol, 41, 274–280.
- Zaman MA, Oparil S, Calhoun DA. (2002). Drugs targeting the renin-angiotensin-aldosterone system. Nat Rev Drug Discov, 1, 621–636.