Abstract
Context: Ginkgo biloba L. (Ginkgoaceae) leaves have been used as an herbal medicine that has a complex range of biological activities. However, when we consider that biological activity of plant extracts is highly variable according to the source, location, and harvest season, technology to obtain the natural products with homogeneity is extremely important.
Objective: We established the technology to obtain the cambial meristematic cells (CMCs) of Ginkgo biloba, which were expanded in vitro with homogeneity through a suspension culture and then determined the anti-inflammatory activity of fractionated samples prepared from the ethanol extract of CMCs.
Materials and methods: We determined the anti-inflammatory activity of samples using lipopolysaccharide (LPS)-stimulated RAW 264.7 macrophage cells. Especially, influence of sample treatment on the expression of various indicators, such as nitric oxide (NO), inducible nitric oxide synthase (iNOS), cyclooxygenase (COX)-2, mitogen-activated protein (MAP) kinases, transcription factor, and cytokines, involved in inflammatory activity was assessed.
Results: A fractionated sample demonstrated 53.4% inhibition of LPS-induced NO production from the cells. Additionally, when fractionated samples were treated, iNOS and COX-2 expressions were almost completely suppressed. Fractionated samples also inhibited the phosphorylation of LPS-induced extracellular signal-regulated (ERK) and p38 MAP kinases more than 60%. IκB phosphorylation and subsequent nuclear factor (NF)-κB activation were also suppressed by fractionated samples. The expression of pro-inflammatory cytokines, IL-6 and tumor necrosis factor (TNF)-α, was significantly inhibited by the sample treatment.
Discussion and conclusion: Fractionated samples from the ethanol extract of Ginkgo biloba CMCs could potentially be the source of a powerful anti-inflammatory substance.
Introduction
Organic changes caused by physical and/or chemical stress as well as microbial infection result in inflammation to restore and revive injured organs and tissues (CitationTizard, 1996). Following inflammatory stimulation, active vascular substances such as histamine, serotonin, bradykinin, prostaglandins, and leukotriene are released locally, vascular permeability is increased, and an inflammatory response is initiated. Macrophages are known to be critically involved in inflammatory response and produce NO and cytokines to protect tissues during the inflammatory responses (CitationHiguchi et al., 1990). However, a prolonged inflammatory reaction facilitates the damage of various tissues and can result in the generation of diverse inflammation-related diseases including cancer (CitationWilloughby, 1975).
As preventive and therapeutic measures addressing inflammatory diseases gain importance, many investigators have focused their efforts on distinguishing anti-inflammatory substances from natural materials. Among the natural materials, plants are a valuable resource for a wide range of secondary metabolites, which are used as pharmaceuticals, agrochemicals, flavors, fragrances, colors, biopesticides, and food additives (CitationRao & Ravishankarb, 2002). In particular, the secondary metabolites of plants are a major source for drugs, with approximately half of all pharmaceuticals in current use originating from natural plant products (CitationClark, 1996). In that sense, it is plausible that various plant-based natural products such as resveratrol and polyphenolic compounds are known as important anti-inflammatory substances (Citationde la Lastra & Villegas, 2005; CitationVafeiadou et al., 2007).
Among the natural plant resources possessing anti-inflammatory properties, Ginkgo biloba L. (Ginkgoaceae) has been used as a traditional medicine to treat long-standing inflammatory disease while the use of ginkgo as an alternative medicine in developed countries has in popularity (CitationKoh, 2010; CitationRogerio et al., 2010; CitationLee et al., 2011). Nut and leaf extracts from ginkgo have been used in traditional oriental medicine for several hundred years. Ginkgo nut extract has been used to treat asthma and cough (CitationSmith & Luo, 2004) while extract from the leaves is known to have anticancer (CitationZhang et al., 2008) and antioxidant (CitationSener et al., 2007) properties as well as a role in the improvement of blood circulation (CitationHuang et al., 2004). Reports indicate that the administration of ginkgo leaf extract resulted in decreases in platelet aggregation, allergic reaction, general inflammatory response, oxygen radical discharge, and other pro-inflammatory functions of macrophages (CitationBraquet, 1987; CitationBraquet & Hosford, 1991). More importantly, studies suggest increased blood flow and anti-inflammatory effects resulting from ginkgo leaf extract are related to the inhibition of COX and lipoxygenase activity (CitationHoughton, 1994). In addition, mechanisms involved in anti-inflammatory activity of leaf extract of Ginkgo biloba have been extensively elucidated (CitationPark et al., 2006). However, there are not many studies about the medicinal efficacy of the ginkgo stem compared with the well-recorded medicinal effects of ginkgo leaf extract.
The biological activity of plant extracts is highly variable according to the plant source, location, and harvest season, and can be adversely affected by unpredictable environmental circumstances (CitationRoberts, 2007). Consequently, plant cell cultures have been considered as an alternative method for producing useful compounds with consistent medicinal plant properties. Plant cell cultures have long been acknowledged as an ideal biotechnology for producing valuable plant-derived compounds stably without any negative environmental influence (CitationRoberts, 2007). However, this technique requires a dedifferentiation procedure to obtain undifferentiated cells with dividing ability from already differentiated plant tissues such as the leaf, stem, and roots (CitationGrafi et al., 2007). During the dedifferentiation process, somatic mutation leading to genetic variation occurs, eventually limiting the stability of secondary metabolite production (CitationBaebler et al., 2002). Genetically stable and physiologically homogeneous cells can be obtained from cambium, a meristematic tissue (vascular stem cells) with division capabilities that can circumvent the dedifferentiation process. As a result, researchers including our group established a technology to isolate and culture pure CMCs from various plants and superior quality of the stem cells was assessed (CitationYe, 2002; CitationHirakawa et al., 2008; CitationLee et al., 2010).
We isolated and cultured CMCs from the ginkgo stem to obtain efficient and stable cell lines and studied the efficacy of these cell lines to enhance the value of ginkgo as an important natural resource. To provide a scientific basis for the anti-inflammatory potential of CMCs cultured from Ginkgo biloba, we prepared fractionated samples from CMC ethanol extract cultured from ginkgo and investigated its effects on well-known indicators of inflammatory response, including NO production, iNOS and COX-2 gene expression, NF-κB activation, and cytokine secretion in LPS-stimulated RAW 264.7 macrophage cells.
Materials and methods
Reagents
Unless otherwise specified, chemicals and laboratory materials were purchased from Sigma Chemical Co. (St. Louis, MO) and Falcon Labware (Becton-Dickinson, Franklin Lakes, NJ), respectively. All monoclonal and polyclonal antibodies used in this study (specific to ERK, p-ERK, P38, p-P38, p-IκBα, IκBα, NF-κB, β-actin, and HDAC2) were purchased from Santa Cruz Biotechnology (Santa Cruz, CA).
Establishment of CMC cultures
Cambial meristematic cells were obtained from Ginkgo biloba cambium using mB5 medium containing 1–3 mg/L auxin, 0.5 mg/L gibberellic acid, 500 mg/L casein hydrolysate, 4 g/L gelrite, and 3% sucrose (CitationGamborg et al., 1968). To establish a CMC suspension culture, CMCs were transferred to a 250 mL Erlenmeyer flask containing the growth medium, which consisted of mB5 medium supplemented with 1–3 mg/L auxin, 0.1 mg/L gibberellic acid, 2% sucrose, and various amino acids. The cell suspension was agitated at 100 rpm at 25 ± 1°C in the dark. A subculture of the CMC suspension culture was taken every two weeks by inoculating 0.17 g (dry cell weight) of CMCs into 70 mL of fresh medium. The bioreactor cultures were performed in 20 L airlift bioreactors by inoculating 2.36 g/L dry cell weight with 80% working volume and air strength of 0.1 volume per volume per minute at 25 ± 1°C in the dark. To confirm the biological activity of CMCs derived from Ginkgo biloba, CMCs were initially expanded in the growth medium described above and then cultured in a production medium containing sterilized water, 30–50 g/L raw sugar, and 100 μM of methyl jasmonate for 14 days in the dark.
Preparation and fractionation of CMC extract
Ethanol extract of CMC derived from ginkgo was prepared as follows. Fresh CMCs (20 g) was mixed with 1 L of 80% ethanol in a flask and shaken at room temperature for two days. The supernatant was collected and concentrated through lyophilization (Eyela Model FDU-2100, Tokyo, Japan), reaching 8 g (40% of the initial weight) of CMC extract.
To prepare fractionated samples from the ethanol extract, 4.2 g of the concentrated powder was dissolved in 80% ethanol and fractionated through column chromatography using a Sephadex LH-20 column (15 × 1260 mm) with a bead volume of 222 mL (Amersham Pharmacia, Piscataway, NJ). The sample was loaded onto the column and eluted with 100% methanol at a flow rate of 0.84 mL/min. The eluted solution was collected every 10 mL/fraction up to 120 fractions. The thin layer chromatography (TLC) pattern of each sample was compared. Fractions showing similar TLC patterns were combined and seven fractionated samples were eventually prepared. Finally, their antioxidant activity was analyzed by determining superoxide anion radical scavenging activity. Fractions #4, #5, and #6, which demonstrated the highest levels of radical scavenging activity, were used to assess anti-inflammatory activity in this study because anti-inflammatory activity is known to be closely related with antioxidant activity (CitationMiddleton, 1998; CitationGarcia-Bailo et al., 2011; CitationGonzalez et al., 2011).
Cell culture and treatment
RAW 264.7 macrophage cells were purchased from the American Type Culture Collection (Manassas, VA) and cultured in Dulbecco’s modified Eagle’s medium (DMEM) supplemented with 10% fetal bovine serum (FBS, HyClone, Logan, UT), 2 mM l-glutamine, and antibiotics. Cells (1 × 106/mL) in 2 mL or 200 μL of media were seeded in 6- or 96-well flat-bottomed plates, respectively. When cells reached 70–80% confluence, the cells were exposed to various concentrations of sample prior to exposure to 1 μg/mL LPS. Cells and/or culture media undergoing LPS treatment were collected and processed at various times for further experiments.
MTT assay
MTT [3-(4,5-dimethylthiazol-2yl-)-2,5-diphenyl tetrazolium bromide] was used to evaluate cell viability. Cells exposed to the samples and/or LPS were treated with 10 µL of MTT solution (5 mg/mL in PBS as stock solution) and then incubated for an additional 4 h at 37°C. Acidic isopropanol (70 μL) were added to 96-multiwell plates, and the absorbance was read at 570 nm using a SpectraCount™ ELISA reader (Packard Instrument Co., Downers Grove, IL).
Measurement of NO production
Nitrite concentration in the culture medium was measured as an indicator of NO production using a Griess reagent (CitationWeismann & Gross, 2001). Briefly, cells incubated in 96-multiwell plates were treated with different concentrations of CMC extract before adding LPS to the cultures. Following 48 h of incubation, the cultured media (100 μL) was mixed with the same volume of Griess reagent, and the absorbance of the mixture was measured at 540 nm using an ELISA reader (Packard Instrument Co.).
RNA isolation and reverse transcriptase-polymerase chain reaction (PCR)
To measure the gene expression levels, total cellular RNA was isolated with a total RNA extraction kit (Invitrogen, Carlsbad, CA). Poly (A)+ RNAs were reverse transcribed to cDNAs using oligo dT primer with reverse transcriptase (Promega, Madison, WI). Specific genes were amplified from the cDNA using Ex-Taq™ DNA polymerase (Takara Bio. Inc., Shiga, Japan) with specific primer sets. The forward and reverse primers for amplifying the COX-2 gene were 5′-AAG AAG AAA GTT CAT TCC TGA TCC C-3′ and 5′-TGA CTG TGG GAG GAT ACA TCT CTC C-3′, respectively. The forward and reverse primers for amplifying the iNOS gene were 5′-CTG CAG CAC TTG GAT CAG GAA CCT G-3′ and 5′-GGG AGT AGC CTG TGT GCA CCT GGA A-3′, respectively. The forward and reverse primers for amplifying the GAPDH gene were 5′-CCG ATG CCC CCA TGT TTG TG-3′ and 5′-GGC CAT GCC AGT GAG CTT CC-3′, respectively. The PCR mixture contained 1 μg cDNA, 10 mM dNTPs, 1x Ex-Taq™ polymerase buffer, and 2.5 U Ex-Taq™ polymerase in a 50 μL final volume. Cycling conditions included an initial melt at 95°C for 2 min followed by 30 cycles of a three-step program (95°C for 1 min, 55°C for 1 min, and 72°C for 1.5 min). The reaction was held at 72°C for 10 min and cooled to 4°C.
Analysis of PCR products
A 10 μL aliquot from each PCR reaction was electrophoresed on a 1% agarose gel containing 0.2 μg/mL ethidium bromide. The gel was then photographed under a UV transilluminator (Vilber Lourmat, Marne-la-Vallée, France). To quantify the PCR product, PCR bands on a photograph of the gel were scanned and analyzed using AlphaDigiDoc 1200 software (Cell Biosciences, Santa Clara, CA). The results were calculated as the percentage of gene expression obtained by LPS treatment after normalizing the gene signal relative to the corresponding GAPDH signal from each sample.
Western blot analysis
Whole lysates from the treated cells were created in a lysis buffer as previously described (CitationSon et al., 2009). Cells were briefly incubated in a 200 μL lysis buffer (250 mM sucrose, 20 mM HEPES, pH 7.5, 10 mM KCl, 1.5 mM MgCl2, 1 mM EGTA, 1 mM EDTA, 1 mM DTT, 1 mM PMSF, and 10 μg/mL each of leupeptin, aprotinin, and pepstatin A) on ice for 30 min. The lysate was then centrifuged at 750g for 10 min at 4°C and the supernatants were further centrifuged at 10,000g for 25 min at 4°C to prepare the cytosolic fraction. The nuclear fraction from the cells was prepared using the NE-PER® nuclear and cytoplasmic extraction reagents kit (Thermo Scientific, Pierce Biotechnology, Rockford, IL) according to the manufacturer’s protocol to analyze NF-κB activation.
Protein from each sample (30 μg) were separated by 10% SDS-PAGE and blotted onto PVDF membranes (Bio-Rad, Hercules, CA). The blots were probed with specific primary and secondary antibodies and then developed with enhanced chemiluminescence (Amersham Pharmacia Biotech, Buckinghamshire, UK) prior to exposure on X-ray film (Eastman-Kodak, Rochester, NY). To quantify the band intensity, blots were scanned and analyzed using AlphaDigiDoc 1200 software (Cell Biosciences). The results were calculated as the percentage of band intensity obtained by LPS treatment after normalizing the intensity relative to the corresponding internal marker signal from each sample.
Measurement of cytokines
The amount of cytokine expression by sample-treated macrophages was determined using ELISA, which was conducted by the Bank for Cytokine Research (Chonbuk National University, Jeonju, Korea). Briefly, cells cultured on a 24-well plate were pre-treated with different concentrations of extract for 30 min before stimulating with 1 μg/mL LPS. After 48 h of stimulation, culture supernatants were collected and assessed by ELISA using TNF-α and IL-6-specific OptEIA™ kit (BD Biosciences, Franklin Lakes, NJ). Cytokine production was calculated from standard curves generated using known concentrations of recombinant cytokine proteins.
Statistical analysis
All data were expressed as the mean ± standard error (SE). One-way analysis of variance (ANOVA, SPSS version 16.0, San Rafael, CA) followed by Scheffe’s test was applied to determine differences between the groups. A value of p < 0.05 was considered significant.
Results
Selection of combined fractions of ethanol extract prepared from CMCs of ginkgo to measure anti-inflammatory activity and their influence on the viability of RAW 264.7 cells
We initially fractionated the ethanol extract of CMCs prepared from ginkgo through column chromatography and, then, combined the fractions to seven samples based on similarity in the TLC pattern. Because anti-inflammatory activity is known to be closely related with antioxidant activity, we initially tested the antioxidant activity of combined fractions based on the superoxide radical scavenging activity of the samples and selected the three fractions displaying the three highest antioxidant activities (Fractions #4–6, data not shown). Fifty micrograms per milliliter of each sample was treated for 48 h and the cell viability was assessed using MTT assay to determine if the samples had any marked influence on the viability of RAW 264.7 macrophage cells (). As shown in , cells treated with crude CMC ethanol extract from ginkgo demonstrated 90% viability compared to the control and did not demonstrate significant cytotoxicity against RAW 264.7 macrophage cells. However, the viability of RAW 264.7 cells was significantly reduced to around 50% of the control value when fractionated samples were treated. This result suggests that the fractionated samples prepared from ginkgo-derived CMC ethanol extract exhibited some degree of adverse effect on cell viability.
Figure 1. Influence of ginkgo-derived CMC ethanol extract sample treatment on the viability of the RAW 264.7 macrophage cell line. RAW 264.7 cells were pre-treated with various concentrations of each sample before adding 1 μg/mL LPS. After 24 h of incubation, the cells were processed using MTT assay to determine viability of the cells. This figure shows the results obtained after treating 50 μg/mL of each sample. CE represents the crude initial ethanol extract of the ginkgo-derived CMCs before fractionation. **p < 0.01 indicates significant differences.
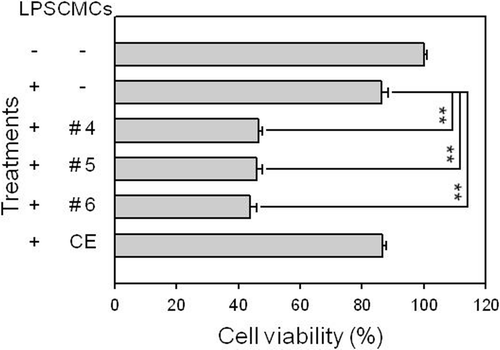
Inhibitory effect of fractionated samples from ginkgo-derived CMCs on nitrite production in LPS-stimulated RAW 264.7 cells
LPS-stimulated macrophage cells are a widely used model in investigating inflammatory response, in which up-regulation of NO production in the cells is one of the critical indicators for the induction of inflammatory responses. To verify whether or not fractionated samples of ginkgo-derived CMCs exhibited anti-inflammatory properties, we assessed the inhibitory effect of the samples on the production of NO from LPS-treated RAW 264.7 macrophage cells by measuring nitrite concentration levels in the culture supernatant using the Griess reaction (). Nearly basal level of nitrite production was detected in RAW 264.7 cells without any treatment, while nitrite production levels were measured at 67.3 ± 0.8 μM in the culture supernatant of LPS-stimulated RAW 264.7 cells. When 50 μg/mL of the crude ethanol extract of ginkgo-derived CMCs was treated, LPS-induced nitrite production from RAW 264.7 cells was significantly inhibited. Furthermore, the same amount of fractionated samples prepared from CMC ethanol extract significantly inhibited LPS-induced nitrite production from the cell line. Fraction #6 demonstrated the strongest inhibitory effect on LPS-induced nitrite production from the macrophage cell line with 35.9 ± 0.1 μM of nitrite detected in the culture supernatant and a degree of inhibition of 53.4% from the LPS-stimulated cells. This result indicates that the fractionated samples prepared from ginkgo-derived CMC ethanol extract have anti-inflammatory potential.
Figure 2. Effect of fractionated sample treatments on nitrite production in the LPS-stimulated RAW 264.7 macrophage cell line. RAW 264.7 cells were pre-treated with various concentrations of each sample before adding 1 μg/mL LPS. After 24 h of incubation, the culture supernatant of the cells was processed to measure nitrite production. This figure shows the results obtained after treating 50 μg/mL of each sample. CE represents the crude initial ethanol extract of the ginkgo-derived CMCs before fractionation. *p < 0.05, **p < 0.01, and ***p < 0.001 indicate significant differences.
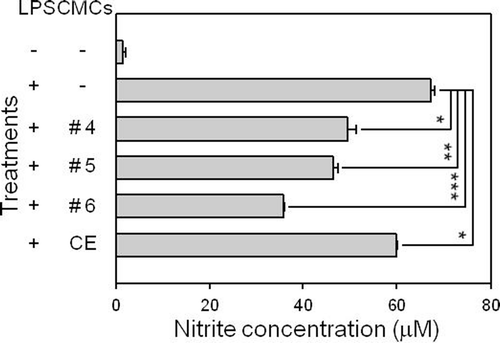
Fractionated samples also inhibited the expression of iNOS and COX-2 genes
Nitric oxide is primarily produced in macrophages following the enzymatic reaction of iNOS (CitationHesslinger et al., 2009) and COX-2 is well-known for its involvement in inflammatory response (CitationZhang & Li, 2009). Because the fractionated samples of ginkgo-derived CMC ethanol extract demonstrated significant inhibitory activity in nitrite production, we further investigated the effect of fractionated sample treatments on COX-2 and iNOS expression in LPS-stimulated RAW 264.7 macrophage cells (). As expected, 1 μg/mL of LPS treatment induced a significant increase in COX-2 and iNOS gene expression in RAW 264.7 cells. Notably, when 50 μg/mL of ginkgo-derived CMC crude ethanol extract was applied, LPS-induced COX-2 and iNOS gene expression was dramatically inhibited by about 83.4% and 57.1%, respectively. In this circumstance, the same concentration of fractionated samples resulted in the inhibition of COX-2 and iNOS gene expression to the point that nearly 69% to 100% of that in LPS-treated cells. These results confirm that the fractionated samples prepared from ginkgo-derived CMCs are very efficient in anti-inflammatory activity.
Figure 3. Influence of fractionated sample treatment on LPS-induced iNOS and COX-2 gene transcription in RAW 264.7 cells. RAW 264.7 cells were pre-treated with various concentrations of each sample before adding 1 μg/mL LPS. After 24 h of incubation, total RNA was extracted and RT-PCR was performed. Levels of GAPDH gene, a RT-PCR product, were used as an internal control. This figure shows the results obtained after treating 50 μg/mL of each sample. CE represents the crude initial ethanol extract of the ginkgo-derived CMCs before fractionation.
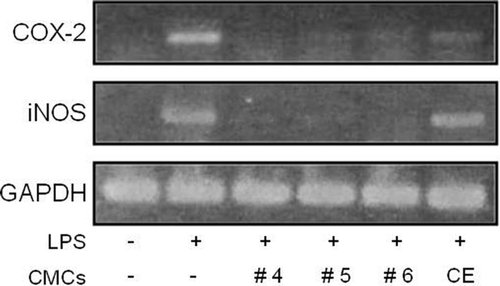
LPS-induced activation of MAP kinases in RAW 264.7 cells was inhibited by the fractionated sample treatment
LPS is known to signal macrophages to produce a variety of inflammatory mediators through the activation of MAP kinases, such as ERK and p38 (CitationSung et al., 2009). We next determined the effect of fractionated samples from ginkgo-derived CMCs on the activation of ERK and p38 MAP kinases in LPS-stimulated RAW 264.7 cells (). As shown in , μg/mL of LPS treatment induced a significant increase in the phosphorylation of ERK1/2 and p38 in RAW 264.7 cells. On the contrary, 50 μg/mL of crude ethanol extract of ginkgo-derived CMCs dramatically reduced the levels of phosphorylated ERK1/2 and p38 to approximately 27.4% and 37.3% of their original value, respectively. Furthermore, the same concentration of these fractionated samples reduced phosphorylated ERK1/2 and p38 levels that had previously been increased by the LPS stimulation of RAW 264.7 cells. Notably, the inhibitory effect of Fraction #4 on the activation of ERK1/2 and p38 MAP kinases was the highest among the three fractionated samples with an inhibition rate of 50% detected in ERK1/2 and p38 MAP kinase activation. These findings suggest that fractionated samples of ginkgo-derived CMCs inhibit LPS-induced NO production via down-regulation of ERK1/2 and p38-mediated pathways in macrophages.
Figure 4. Influence of fractionated sample treatment on phosphorylation of ERK and p38 MAP kinases in LPS-stimulated RAW 264.7 cells. Cells were exposed to 50 μg/mL of the fractionated samples 30 min before exposure to LPS (1 μg/mL). Thirty minutes after the LPS treatment, phosphorylated ERK and p38 levels were detected using specific antibodies. The level of β-actin protein expression was used as an internal control. CE represents the crude ethanol extract, which was the initial ethanol extract prepared from ginkgo-derived CMCs before fractionation.
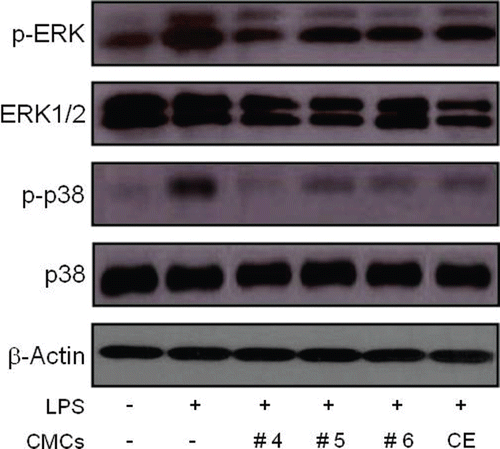
Fractionated samples from ginkgo-derived CMCs inhibit the activation of NF-κB, the degradation of IκB, and the expression of pro-inflammatory cytokines in LPS-stimulated RAW 264.7 cells
The phosphorylation of MAP kinases and NF-κB activation are crucial events in the inflammatory response process. IκB degradation followed by its phosphorylation is an essential step for the nuclear translocation of NF-κB, which is a critical transcription factor in pro-inflammatory cytokine gene expression (CitationKang et al., 2009). As a result, we determined the effect of fractionated samples from ginkgo-derived CMCs on NF-κB activation and phosphorylation of IκB in LPS-stimulated macrophage cells (). As expected, the LPS treatment increased NF-κB levels in the nuclear extract of LPS-treated RAW 264.7 cells (). However, the pretreatment of cells with ginkgo-derived CMC crude ethanol extract slightly inhibited the activation of NF-κB by about 10.7%. More importantly, the fractionated sample treatment dramatically inhibited the activation of NF-κB with an inhibition rate of nearly 70% of the level obtained from control LPS-treated cells. The level of p-IκB in whole cell lysates which will be subsequently degraded was also dramatically inhibited by the fractionated sample treatment.
Figure 5. Influence of fractionated sample treatment on the activation of NF-κB and phosphorylation of IκB in LPS-stimulated RAW 264.7 cells. Cells were exposed to 50 μg/mL of the fractionated samples 30 min prior to the LPS (1 μg/mL) treatment. Two hours after the LPS treatment, nuclear extract and whole cell lysates were extracted and NF-κB and IκB levels were detected using specific antibodies from the nuclear extract and whole cell lysate, respectively. HDAC2 protein levels were used as an internal control. CE represents the crude ethanol extract, which was the initial ethanol extract of the ginkgo-derived CMCs before fractionation.
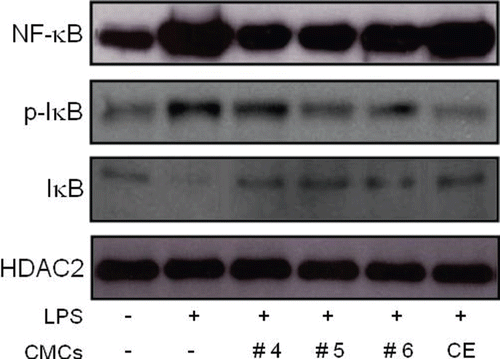
We also assessed the effect of the fractionated sample treatment on the production of pro-inflammatory cytokines, TNF-α and IL-6, in LPS-exposed RAW 264.7 cells (). The addition of LPS into RAW 264.7 cells increased the production of both pro-inflammatory cytokines. As expected, the treatment of LPS-stimulated RAW 264.7 cells with fractionated samples significantly inhibited the production of the pro-inflammatory cytokines, while the crude ethanol extract itself demonstrated no significant inhibitory effect on the production of pro-inflammatory cytokines in the macrophage cells. These results confirm that the fractionated samples from ginkgo-derived CMCs exhibit anti-inflammatory potential in LPS-stimulated macrophage cells.
Figure 6. Influence of fractionated sample treatment on the production of pro-inflammatory cytokines in LPS-stimulated RAW 264.7 cells. Cells were stimulated with 1 μg/mL of LPS in the presence of a 50 μg/mL fractionated sample treatment. After 48 h of incubation pro-inflammatory cytokines TNF-α, and IL-6 were measured in the culture supernatant using ELISA. Data represent the mean value of triplicates and *p < 0.05 and **p < 0.01 indicate significant differences.
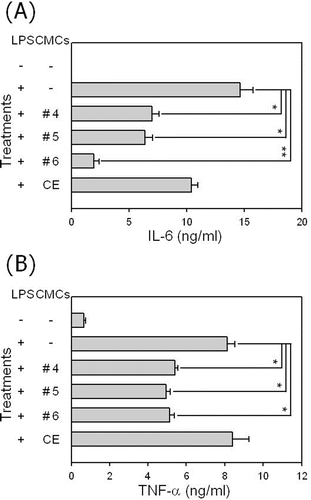
Discussion
Inflammation is a host response to restore and revive injured organs and tissues. Inflammation is mediated by complex processes with several signs, in which macrophages play an important role (CitationLaskin & Pendino, 1995; CitationHawiger, 2001). The overproduction of inflammatory mediators by macrophages has been implicated in several inflammatory diseases and cancers (CitationLin & Karin, 2007). Consequently, LPS-stimulated macrophage cells are a widely used model in investigating inflammatory response. LPS signals macrophages to produce a variety of inflammatory mediators through the activation of MAP kinases (CitationMurakami, 2009) and the MAP kinases, ERK and p38, are involved in the up-regulation of iNOS induction and NO production in LPS-stimulated macrophage cells (CitationSung et al., 2009). MAP kinases also induce the activation of NF-κB, which is involved in the expression of pro-inflammatory cytokines including IFN-γ, IL-6, IL-1β, and TNF-α (CitationSung & Wong, 2007; CitationVuolteenaho et al., 2007).
The overproduction of NO by iNOS is also closely involved in the pathogenesis of numerous diseases such as carcinogenesis, septic shock, rheumatoid arthritis, and autoimmune diabetes (CitationHesslinger et al., 2009; CitationKern, 2007; CitationKanwar et al., 2009). Additionally, COX-2 is well-known for its involvement in inflammatory response (CitationZhang & Li, 2009). Thus, we have performed our studies in murine macrophage cell line RAW 264.7 cells, which express iNOS and COX-2, following stimulating with LPS ( and ). Our results demonstrate that LPS-induced NO production in RAW 264.7 cells is susceptible to inhibition by the fractionated sample of ginkgo-derived CMC ethanol extract. These results confirm that the fractionated samples prepared from ginkgo-derived CMCs are very efficient in anti-inflammatory activity (). We also demonstrated that fractionated samples prepared ginkgo-derived CMC ethanol extract inhibited COX-2 expression in LPS-stimulated macrophage cells (). Consequently, our data strongly indicate that fractionated samples of ginkgo-derived CMCs inhibit the key mediators developed during activation of macrophages.
LPS is known to signal macrophages to produce a variety of inflammatory mediators through the activation of MAP kinases, such as ERK and p38 (CitationSung et al., 2009). MAP kinases regulate a broad range of cellular events including proliferation, differentiation, and apoptosis (CitationWagner & Nebreda, 2009). MAP kinases also mediate cellular signaling involved during the activation of macrophages in response to microbial pathogens (CitationGaestel et al., 2007). Our current results consequently indicate that fractionated samples of ginkgo-derived CMCs blocked LPS-induced NO production by inhibiting the phosphorylation of ERK1/2 and p38 MAP kinases in LPS-stimulated RAW 264.7 macrophage cells (). This is consistent with previous reports indicating that luteolin suppresses LPS-induced NO production via the inhibition of ERK1/2 and p38 activation (CitationXagorari et al., 2002). Previous reports also indicate that vitisin A, a resveratrol tetramer, prevents LPS-induced NO production by inhibiting ERK and p38 phosphorylation in the macrophages (CitationSung et al., 2009). However, other studies suggest that either ERK1/2 or p38 MAP kinase, but not both, can be related to LPS-induced NO production (CitationWatters et al., 2002; CitationKang et al., 2008; CitationKim et al., 2009). Although it is unclear whether the activation of just ERK1/2, just p38 MAP kinase, or both is necessary for LPS-induced NO production in macrophages, our data suggest that LPS activates macrophages through the phosphorylation of both ERK1/2 and p38. In addition, fractionated samples from ginkgo-derived CMCs later demonstrated powerful inhibitory effects on the activation of the MAP kinases.
The phosphorylation of MAP kinases and NF-κB activation are crucial events in the inflammatory response process. IκB degradation followed by its phosphorylation is an essential step for the nuclear translocation of NF-κB, which is a critical transcription factor in pro-inflammatory cytokine gene expression (CitationKang et al., 2009). MAP kinases tightly regulate the activity of NF-κB transcription factor, which is essential for iNOS gene transcription and subsequent NO production. This study observed that fractionated samples from ginkgo-derived CMCs inhibited LPS-induced NF-κB activation in macrophage cells (). The fractionated samples also blocked IκB degradation by inhibiting IκB phosphorylation in LPS-activated cells. As a result, we predicted that fractionated samples block LPS-induced NO production through the inhibition of ERK/p38-mediated NF-κB activation and subsequent iNOS expression. We also considered that the fraction-mediated decrease of pro-inflammatory cytokines in LPS-stimulated cells is related to its inhibitory potential on NF-κB activation. This is because NF-κB activation is necessary for the production of cytokines, such as TNF-α, IL-1β, and IL-6, by activated macrophages.
Many researchers indicate the benefits of phenolic compounds in plant resources on antioxidant, antitumor, and anti-inflammatory properties. We presume the phenolic compounds included in the fractionated samples from ginkgo-derived CMCs may be responsible for their anti-inflammatory and antioxidative properties (CitationRíos et al., 2009; CitationWilson, 2009). Polyphenolic compounds including catechin, quercetin, myricetin, and myricetin-rutinosides are identified as the main components of ethanol extract from many plants (CitationZhao et al., 2007). In the fractionated samples, we also identified gallocatechin and catechin as major active ingredients through NMR analysis after fractionation with prep-HPLC using a C18 column (manuscript in preparation). However, other reports indicate that purified bioactive materials often display a certain level of toxicity in cells (CitationLee et al., 2002, Citation2003). We believe that the inhibitory effect of the fractionated samples on the viability of LPS-treated RAW 264. 7 cells may be due to the concentrated bioactive ingredients found in ginkgo-derived CMCs (). On the other hand, certain toxic impurities may be concentrated and the fractionated samples display a significant inhibitory effect on LPS-treated RAW 264.7 cells. In any case, the observation that fractionated samples display a different degree of inhibition on various inflammatory response indicators of similar cytotoxicity suggests that there may be a complex mixture of active ingredients involved in anti-inflammatory activity in fractionated samples. As a result, it is necessary to determine the appropriate treatment dose that yields maximum benefits with minimal adverse effects.
Conclusion
Anti-inflammatory activity of ginkgo leaf extract has been well-investigated. However, when we consider that the biological activity of plant extract is highly variable according to the plant source and affected by environmental circumstances, technology to obtain plant tissue or cells with homogeneity is extremely important. We previously established the technology to obtain CMCs of Ginkgo biloba through in vitro suspension culture and tested their anti-inflammatory activity in this study. This study demonstrates that fractionated samples prepared from ginkgo-derived CMC ethanol extract exhibited clear anti-inflammatory properties related to LPS-activated macrophages. Furthermore, this study provides the first scientific basis for the use of ginkgo-derived CMCs to develop a therapeutic approach for the treatment of inflammatory disease.
Acknowledgements
This work was supported by a grant from Ministry of Knowledge Economy, Republic of Korea (No. 70004355).
Declaration of interest
The authors report no declarations of interest.
References
- Baebler S, Camloh M, Kovac M, Ravnikar M, Zel J. (2002). Jasmonic acid stimulates taxane production in cell suspension culture of yew (Taxus x media). Planta Med, 68, 475–476.
- Braquet P. (1987). The ginkgolides: Potent platelet-activating factor antagonists isolated from Ginkgo biloba L.: Chemistry, pharmacology and clinical applications. Drugs Future, 12, 643–649.
- Braquet P, Hosford D. (1991). Ethnopharmacology and the development of natural PAF antagonists as therapeutic agents. J Ethnopharmacol, 32, 135–139.
- Clark AM. (1996). Natural products as a resource for new drugs. Pharm Res, 13, 1133–1144.
- de la Lastra CA, Villegas I. (2005). Resveratrol as an anti-inflammatory and anti-aging agent: Mechanisms and clinical implications. Mol Nutr Food Res, 49, 405–430.
- Gaestel M, Mengel A, Bothe U, Asadullah K. (2007). Protein kinases as small molecule inhibitor targets in inflammation. Curr Med Chem, 14, 2214–2234.
- Gamborg OL, Miller RA, Ojima K. (1968). Nutrient requirements of suspension cultures of soybean root cells. Exp Cell Res, 50, 151–158.
- Garcia-Bailo B, El-Sohemy A, Haddad PS, Arora P, Benzaied F, Karmali M, Badawi A. (2011). Vitamins D, C, and E in the prevention of type 2 diabetes mellitus: Modulation of inflammation and oxidative stress. Biologics, 5, 7–19.
- González R, Ballester I, López-Posadas R, Suárez MD, Zarzuelo A, Martínez-Augustin O, Sánchez de Medina F. (2011). Effects of flavonoids and other polyphenols on inflammation. Crit Rev Food Sci Nutr, 51, 331–362.
- Grafi G, Ben-Meir H, Avivi Y, Moshe M, Dahan Y, Zemach A. (2007). Histone methylation controls telomerase-independent telomere lengthening in cells undergoing dedifferentiation. Dev Biol, 306, 838–846.
- Hawiger J. (2001). Innate immunity and inflammation: A transcriptional paradigm. Immunol Res, 23, 99–109.
- Hesslinger C, Strub A, Boer R, Ulrich WR, Lehner MD, Braun C. (2009). Inhibition of inducible nitric oxide synthase in respiratory diseases. Biochem Soc Trans, 37, 886–891.
- Higuchi M, Higashi N, Taki H, Osawa T. (1990). Cytolytic mechanisms of activated macrophages. Tumor necrosis factor and l-arginine-dependent mechanisms act synergistically as the major cytolytic mechanisms of activated macrophages. J Immunol, 144, 1425–1431.
- Hirakawa Y, Shinohara H, Kondo Y, Inoue A, Nakanomyo I, Ogawa M, Sawa S, Ohashi-Ito K, Matsubayashi Y, Fukuda H. (2008). Non-cell-autonomous control of vascular stem cell fate by a CLE peptide/receptor system. Proc Natl Acad Sci USA, 105, 15208–15213.
- Houghton P. (1994). Herbal products. 2. Ginkgo. Pharm J, 253, 122–124.
- Huang SY, Jeng C, Kao SC, Yu JJ, Liu DZ. (2004). Improved haemorrheological properties by Ginkgo biloba extract (Egb 761) in type 2 diabetes mellitus complicated with retinopathy. Clin Nutr, 23, 615–621.
- Kang EH, Gebru E, Kim MH, Cheng H, Park SC. (2009). EstA protein, a novel virulence factor of Streptococcus pneumoniae, induces nitric oxide and pro-inflammatory cytokine production in RAW 264.7 macrophages through NF κB/MAPK. Microb Pathog, 47, 196–201.
- Kang JS, Lee KH, Han MH, Lee H, Ahn JM, Han SB, Han G, Lee K, Park SK, Kim HM. (2008). Antiinflammatory activity of methanol extract isolated from stem bark of Magnolia kobus. Phytother Res, 22, 883–888.
- Kanwar JR, Kanwar RK, Burrow H, Baratchi S. (2009). Recent advances on the roles of NO in cancer and chronic inflammatory disorders. Curr Med Chem, 16, 2373–2394.
- Kern TS. (2007). Contributions of inflammatory processes to the development of the early stages of diabetic retinopathy. Exp Diabetes Res, 2007, 95103.
- Kim JY, Shin JS, Ryu JH, Kim SY, Cho YW, Choi JH, Lee KT. (2009). Anti-inflammatory effect of anemarsaponin B isolated from the rhizomes of Anemarrhena asphodeloides in LPS-induced RAW 264.7 macrophages is mediated by negative regulation of the nuclear factor κB and p38 pathways. Food Chem Toxicol, 47, 1610–1617.
- Koh P-O. (2010). Ginkgo biloba extract (EGb 761) prevents cerebral ischemia-induced p70S6 kinase and S6 phosphorylation. Am J Chin Med, 38, 815–827.
- Laskin DL, Pendino KJ. (1995). Macrophages and inflammatory mediators in tissue injury. Annu Rev Pharmacol Toxicol, 35, 655–677.
- Lee EK, Jin YW, Park JH, Yoo YM, Hong SM, Amir R, Yan Z, Kwon E, Elfick A, Tomlinson S, Halbritter F, Waibel T, Yun BW, Loake GJ. (2010). Cultured cambial meristematic cells as a source of plant natural products. Nat Biotechnol, 28, 1213–1217.
- Lee JC, Lim KT, Jang YS. (2002). Identification of Rhus verniciflua Stokes compounds that exhibit free radical scavenging and anti-apoptotic properties. Biochim Biophys Acta, 1570, 181–191.
- Lee JC, Kim J, Jang YS. (2003). Ethanol-eluted extract of Rhus verniciflua stokes inhibits cell growth and induces apoptosis in human lymphoma cells. J Biochem Mol Biol, 36, 337–343.
- Lee YW, Lin JA, Chang CC, Chen YH, Liu PL, Lee AW, Tsai JC, Li CY, Tsai CS, Chen TL, Lin FY. (2011). Ginkgo biloba extract suppresses endotoxin-mediated monocyte activation by inhibiting nitric oxide- and tristetraprolin-mediated toll-like receptor 4 expression. J Nutr Biochem, 22, 351–359.
- Lin WW, Karin M. (2007). A cytokine-mediated link between innate immunity, inflammation, and cancer. J Clin Invest, 117, 1175–1183.
- Middleton EJr.. (1998). Effect of plant flavonoids on immune and inflammatory cell function. Adv Exp Med Biol, 439, 175–182.
- Murakami A. (2009). Chemoprevention with phytochemicals targeting inducible nitric oxide synthase. Forum Nutr, 61, 193–203.
- Park YM, Won JH, Yun KJ, Ryu JH, Han YN, Choi SK, Lee KT. (2006). Preventive effect of Ginkgo biloba extract (GBB) on the lipopolysaccharide-induced expressions of inducible nitric oxide synthase and cyclooxygenase-2 via suppression of nuclear factor-κB in RAW 264.7 cells. Biol Pharm Bull, 29, 985–990.
- Rao SR, Ravishankar GA. (2002). Plant cell cultures: Chemical factories of secondary metabolites. Biotechnol Adv, 20, 101–153.
- Ríos JL, Recio MC, Escandell JM, Andújar I. (2009). Inhibition of transcription factors by plant-derived compounds and their implications in inflammation and cancer. Curr Pharm Des, 15, 1212–1237.
- Roberts SC. (2007). Production and engineering of terpenoids in plant cell culture. Nat Chem Biol, 3, 387–395.
- Rogerio AP, Sá-Nunes A, Faccioli LH. (2010). The activity of medicinal plants and secondary metabolites on eosinophilic inflammation. Pharmacol Res, 62, 298–307.
- Sener G, Sehirli O, Tozan A, Velioglu-Ovunç A, Gedik N, Omurtag GZ. (2007). Ginkgo biloba extract protects against mercury(II)-induced oxidative tissue damage in rats. Food Chem Toxicol, 45, 543–550.
- Smith JV, Luo Y. (2004). Studies on molecular mechanisms of Ginkgo biloba extract. Appl Microbiol Biotechnol, 64, 465–472.
- Son YO, Jang YS, Heo JS, Chung WT, Choi KC, Lee JC. (2009). Apoptosis-inducing factor plays a critical role in caspase-independent, pyknotic cell death in hydrogen peroxide-exposed cells. Apoptosis, 14, 796–808.
- Sung CS, Wong CS. (2007). Cellular mechanisms of neuroinflammatory pain: The role of interleukin-1beta. Acta Anaesthesiol Taiwan, 45, 103–109.
- Sung MJ, Davaatseren M, Kim W, Park SK, Kim SH, Hur HJ, Kim MS, Kim YS, Kwon DY. (2009). Vitisin A suppresses LPS-induced NO production by inhibiting ERK, p38, and NF-κB activation in RAW 264.7 cells. Int Immunopharmacol, 9, 319–323.
- Tizard IR. (1996). Inflammation. In: Veterinary Immunology: An Introduction. 2nd ed. New York: Saunders College Publishing, 423–441.
- Vafeiadou K, Vauzour D, Spencer JP. (2007). Neuroinflammation and its modulation by flavonoids. Endocr Metab Immune Disord Drug Targets, 7, 211–224.
- Vuolteenaho K, Moilanen T, Knowles RG, Moilanen E. (2007). The role of nitric oxide in osteoarthritis. Scand J Rheumatol, 36, 247–258.
- Wagner EF, Nebreda AR. (2009). Signal integration by JNK and p38 MAPK pathways in cancer development. Nat Rev Cancer, 9, 537–549.
- Watters JJ, Sommer JA, Pfeiffer ZA, Prabhu U, Guerra AN, Bertics PJ. (2002). A differential role for the mitogen-activated protein kinases in lipopolysaccharide signaling: the MEK/ERK pathway is not essential for nitric oxide and interleukin 1β production. J Biol Chem, 277, 9077–9087.
- Weissman BA, Gross SS. (2001). Measurement of NO and NO synthase. Curr Protoc Neurosci, Chapter 7, Unit7.13.
- Willoughby DA. (1975). Heberden Oration, 1974. Human arthritis applied to animal models. Towards a better therapy. Ann Rheum Dis, 34, 471–478.
- Wilson JX. (2009). Mechanism of action of vitamin C in sepsis: Ascorbate modulates redox signaling in endothelium. Biofactors, 35, 5–13.
- Xagorari A, Roussos C, Papapetropoulos A. (2002). Inhibition of LPS-stimulated pathways in macrophages by the flavonoid luteolin. Br J Pharmacol, 136, 1058–1064.
- Ye ZH. (2002). Vascular tissue differentiation and pattern formation in plants. Annu Rev Plant Biol, 53, 183–202.
- Zhao QW, Huang X, Lou YJ, Weber N, Proksch P. (2007). Effects of ethanol extracts from Adzuki bean (Phaseolus angularis Wight.) and Lima bean (Phaseolus lunatus L.) on estrogen and progesterone receptor phenotypes of MCF-7/BOS cells. Phytother Res, 21, 648–652.
- Zhang R, Li S. (2009). COX-2 as a novel target of CRF family peptides’ participating in inflammation. Biochem Biophys Res Commun, 382, 483–485.
- Zhang Y, Chen AY, Li M, Chen C, Yao Q. (2008). Ginkgo biloba extract kaempferol inhibits cell proliferation and induces apoptosis in pancreatic cancer cells. J Surg Res, 148, 17–23.