Abstract
Context: The marine diatoms Cocconeis scutellum Ehrenberg (Bacillariophyceae) are known to trigger apoptosis in the androgenic gland of the Mediterranean crustacean Hippolyte inermis Leach (Decapoda), affecting the shrimp’s sex reversal.
Objective: The aim of this study was to evaluate a possible apoptotic effect of extracts and fractions from these microalgae also on human tissues.
Materials and methods: The chemical profile of C. scutellum was determined by gas chromatography-mass spectrometry (GC-MS) and, afterwards, organic extracts and fractions from the diatoms were used to treat to breast cancer BT20 cells. Double labeling with annexin V-FITC and isotonic propidium iodide (PI) along with flow cytometry analysis enabled the evaluate of cell apoptosis and viability, whereas hypotonic PI staining was used to analyze the cell cycle in BT20 lines. The involvement of specific caspases was studied by Western blotting.
Results: Results demonstrated that the diethyl ether extract and, in particular, fraction 3, the richest fraction in eicosapentaenoic acid (EPA) from the diethyl ether extract, selectively induced apoptosis (up to 89.2% at 1 μg/well of fraction 3) and decreased viability in BT20 cells. The apoptotic effect was displayed in a concentration and time-dependent manner, by activating caspases-8 and 3, and arresting the progression of the cell cycle from S to G2-M phase. EPA alone showed similar apoptotic effects in BT20 cells.
Discussion and conclusion: The study demonstrates the apoptotic activity of C. scutellum diatoms on breast cancer cells and suggests their potential use as a source of apoptotic compounds.
Keywords::
Introduction
Several pathologies, such as neurodegenerative diseases or cancer, are due to the deregulation of apoptosis, an important mechanism for controlling homeostasis and development in multicellular organisms (CitationVaux & Korsmeyer, 1999). Treating tumors through selective induction and manipulation of apoptosis in malignant cells, avoiding drug-resistance and side effects, has been recognized as a novel strategy in cancer therapy (CitationLowe & Lin, 2000), hence increasing the needs to find apoptotic compounds from nature.
The sea, with its high biodiversity, is a rich source of molecules provided with apoptotic activity. In fact, some products of marine origin have overcome the clinical trials and have been introduced into the therapeutic protocols (CitationJimeno, 2007). Fish oil, containing docosahexaenoic acid and eicosapentaenoic acid (EPA), has shown to inhibit the proliferation of the human breast cancer cell line MDA-MB-231 (CitationMandal et al., 2010). EPA, in addition, induced apoptosis in human promyelocytic HL-60 cells and pancreatic cancer cell lines SW1990, AsPC-1, and PANC-1 (CitationGillis et al., 2007).
Diatoms have been traditionally considered as a key point in the marine food chain providing sea animals with polyunsaturated fatty acids (PUFAs), including EPA. Previous studies showed that Cocconeis scutellum Ehrenberg (Bacillariophyceae) diatoms provoke specific apoptosis in the androgenic gland of the decapod Hippolyte inermis Leach (Decapoda) (CitationZupo & Messina, 2007), affecting the crustacean’s sex reversal. Here we report the potential of C. scutellum diethyl ether extract and its fractions, and EPA to induce apoptosis in several human cell lines, and mainly in the breast carcinoma-derived line BT20.
Material and methods
Diatom cultivation
C. scutellum diatoms were isolated and cultivated in the Stazione Zoologica “A. Dohrn”, Ischia, (Italy) as described in CitationZupo and Messina (2007).
Metabolite analysis
C. scutellum diatoms (1.5 g dry weight) were processed to obtain both the diethyl ether and the butanol extracts. The diethyl ether extract (182 mg) was separated by Sephadex™ LH-20 column [Amersham Pharmacia Biotech (Uppsala, Sweden)] which was eluted with chloroform/methanol 1:1 v/v to afford three fractions: 1 (43 mg), 2 (40 mg) and 3 (72 mg). Aliquots of fractions 1-3 (ca. 2–3 mg) were silylated, transmethylated and analyzed by gas chromatography-mass spectrometry (GC-MS) as previously described (CitationNappo et al., 2009).
Cell cultures
BT20 (breast carcinoma), MB-MDA468 (breast adenocarcinoma), LNCaP (prostate carcinoma), COR (Epstein Barr Virus-transformed B cells isolated from human tonsils), JVM2 [Burkitt’s lymphoma t(11;14)] and BRG-M [Burkitt’s lymphoma t(8;14)] cell lines were cultured as reported in CitationNappo (2010).
Apoptosis experiments
C. scutellum diatoms (3.5 mg) were dissolved in distilled water and administered to the cancer cell lines at different concentrations (0.1–10 ng/ml).
Aliquots of both the diethyl ether and the butanol extracts, and fractions 1–3, were dissolved in distilled water and dimethyl sulfoxide (DMSO) to afford concentrations in the range of 0–1.7 and 0.1–4 μg/well, respectively. BT20 cell lines were incubated for 18 h with the extracts, and for 24 h with fractions 1–3. EPA and AA (Sigma Aldrich) were tested at 7 μg/well in DMSO on BT20 cells incubated for 18 and 24 h.
In the cell cycle analysis, BT20 populations were incubated with fractions 1–2 at 2.5 μg/well, and fraction 3 at 4 μg/well for 48 h, concentrations which were determined by preceding titration experiments. The experimental conditions, the statistical analysis of all the assays, the evaluation of both cell viability and apoptosis as well as the methods of staining and quantification of cells in each cell cycle phase were reported in CitationNappo (2010).
Western blotting experiments
After stimulating BT20 populations with fraction 1 (4.1 μg/well), fraction-2 (2.7 μg/well), fraction 3 (2.0 and 4.0 μg/well), Vepesid, and the medium only, cells were lysed and their proteins were separated by SDS-PAGE (10% acrylamide) electrophoresis, probing the filters with anti-caspase 8, anti-caspase 9 and anti-caspase 3 mAntibodies. Further details can be found in CitationNappo (2010).
Results
C. scutellum diatoms, responsible for triggering apoptosis in the crustacean Hippolyte inermis’ androgenic gland during its post larval development (CitationZupo & Messina, 2007), were investigated in order to establish whether they displayed apoptotic activity also on human tissues. The preliminary screening (unpublished data from the authors) revealed that BT20 is the most responsive cell line to C. scutellum (up to 26.1% of apoptotic cells at 2.5 ng/ml of diatoms).
MB-MDA468 and LNCaP cell lines, were also affected, while BRG-M, COR and JVM2 apoptotic cells were comparable with those of the negative control (only medium used). These results demonstrated that diatom apoptotic activity was more selective towards solid tumors with respect to the hematologic ones.
At each tested concentration, the diethyl ether extract produced a higher amount of apoptotic cells than the butanol extract. The active extract was, thus, fractionated and the metabolite profiles of the obtained fractions (1–3) are presented in . Fraction 1 consisted mainly of glycerides (77.2% of total ion current) and fatty acids (FAs, 2.4%). Fraction-2 contains mainly FAs (66.7%), monoglycerides (11.0%) and sterols (3.2%), while fraction 3 was composed mainly by FAs (81.7%) and 4-methylcholesterol (2.3%).
Table 1. Metabolite profiles of fractions 1–3 determined by GC-MS and reported as % of TIC (total ion current).
BT20 cells () were highly viable upon incubation with fractions 1 (93.5%), 2 (92.1%), and the medium (90.0%), whereas their viability was reduced at 56.2% in presence of fraction 3. On the other hand, the percentage of late apoptotic cells in fraction 3-treated cells (20.5%) was significantly higher with respect to those incubated with the control (4.4%), fraction 1 (2.4%) and fraction-2 (2.6%). The apoptotic activity of fractions 1–3 on BT20 cells was dose-dependent, reaching a maximum (89.2% of apoptotic cells) at 1 μg/well of fraction 3. On the contrary, apoptotic cells at 0.1 μg/ml of fraction-2 was ca. 40%, whereas fraction 1 produced comparable effects with the control.
Figure 1. Annexin V-FITC isotonic PI double staining experiments along with flow cytometry analysis on BT20 cells incubated with fractions 1–3. The graphs on the left column represent physical parameters such as cell dimension and granulation. On the right column, each graph consists in four quadrants: lower left (LL) representing live cells; lower right (LR) with cells in early phase of apoptosis; upper right (UR) corresponding to cells in late phase of apoptosis; upper left (UL) which constitutes dead cells.
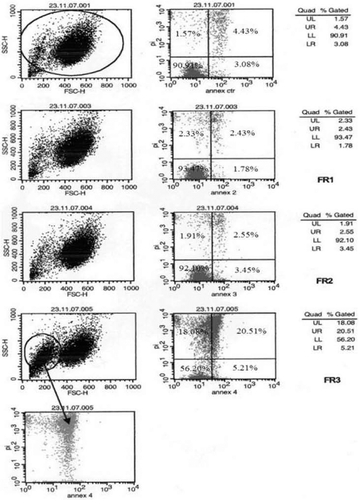
The fact that fraction-2 displayed apoptotic activity, but less than fraction 3, this latter containing more FAs (in fraction 3 EPA was ca. two-folder more than fraction-2), less glycerides (traces) and comparable proportion of sterols, led us to suppose that FAs could be responsible for the highest activity of fraction 3. Among the FAs found in fraction 3 (), only EPA (C20:5) has been reported to possess bioactivity (CitationChajès et al., 1995). Thus, we tested the apoptotic activity of EPA along with that of arachidonic acid (AA, C20:4), another PUFA detected in the diethyl ether extract (CitationNappo et al., 2009). The viable and apoptotic BT20 cells were 34.1% and 45.3%, respectively, at 7 μg/well of EPA. At 7 μg/well of AA, characterized by one double bound less than EPA, both BT20 cell viability (70.4%) and apoptosis (20.2%) did not show remarkable alterations in comparison with the control (78.0% and 14.3%, respectively).
Similar percentages of cells in G0/G1 phase were observed in the control and BT20 cells incubated with fractions 1–3 (50–62%, ). Fraction 3 reduced the amount of cells in S phase (18%), whereas the effects of fractions 1 and 2 were comparable to the control (30–33%). Cells were not found in G2-M phase after treatment with fraction 3, while 10%, 12% and 7% of cells were detected in this phase for the control, fraction 1 and 2-treated cells, respectively.
Figure 2. Determination of the cell amounts in each phase of the cell cycle by hypotonic PI staining, followed by cytometry analysis. BT20 cells were incubated with fractions 1–2 (2.5 μg/well) and fraction 3 (4 μg/well) for 48 h. Only fraction 3 provoked 32% of apoptotic cells and arrested the cell cycle at G2/M phase.
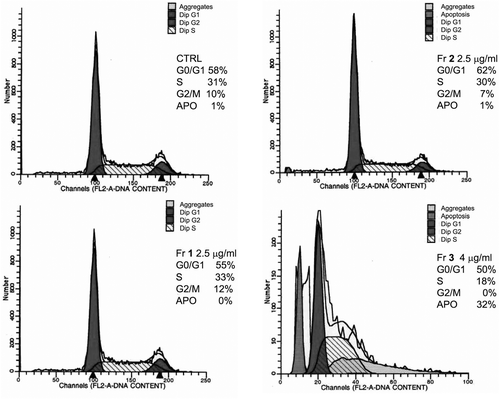
The results shown in indicate that apoptosis in BT20 cells is triggered by activation of caspase-8, the initiator protease involved in the extrinsic pathway. The cleaved/active caspase-8 (40–36 kDa) was found mainly in fraction 3-treated BT20 cells, but also in samples incubated with fraction-2 and Vepesid, whereas the inactive form (55–50 kDa) was present in all the samples. The intrinsic pathway, mediated by caspase-9, thus, is not activated by C. scutellum diatoms. Furthermore, as shown in , upon incubation of BT20 cells with EPA and fraction 3 for 6 h, the activation of caspase-3 (at 20 kDa) is also observed.
Figure 3. Western blotting experiments. (a): Caspase-9 analysis (47 kDa: uncleaved caspase-9; 37 kDa: active form) upon 5, 6 and 18 h of incubation. (b): Caspase-8 analysis (55–50 kDa: uncleaved caspase-8; 40–36 kDa: active caspase-8). Time of incubation: 5 h. (c-d): Caspase-3 analysis (34 kDa: uncleaved caspase-3; 20 kDa: active caspase-3). Times of incubation: 3, 6 and 18 h. Active caspase-8 was found mainly in BT20 cells incubated with fraction 3 at 2 and 4 μg/well, and in a smaller amount also in those with fraction-2 and Vepesid. Active caspase-3 was found in BT20 cells treated with fraction 3 and EPA after 6 h incubation.
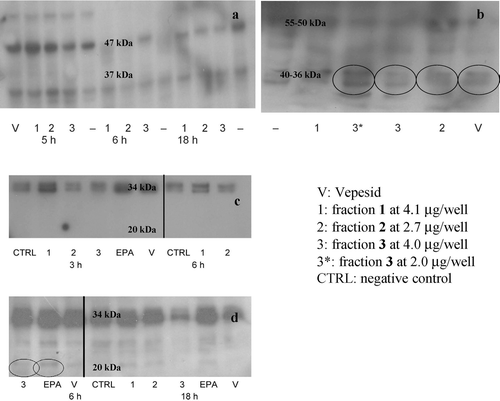
Although the molecular mechanisms of apoptosis have not been described in detail yet, it can be concluded that C. scutellum fractions and EPA induce apoptosis and decrease viability in the breast cancer cell line BT20 in concentration and time-dependent manner, associated with activation of caspases-8 and 3, and arresting the progression of the cell cycle from S to G2-M phase. Breast cancer comprises 22.9% of invasive cancers in women (CitationLin et al., 2007). Greater understanding of the molecular events associated with the biological activity of EPA should enhance the therapeutic potential of administration of EPA and associated algal extracts to these patients. This study, in addition, represents a further demonstration of the interesting bioactivity and potential applications of molecules of marine origin.
Discussion
The benthic diatom C. scutellum, responsible for triggering apoptosis in Hippolyte inermis’ androgenic gland (CitationZupo, 2000; CitationZupo & Messina, 2007), was investigated in order to establish whether it displayed an apoptotic effect also on human tissues. Diatoms have been traditionally considered a key point in the marine food chain. however, many studies deal with the toxic activity of some microalgae towards their predators (CitationShaw et al., 1995; CitationIanora et al., 1996; CitationLandsberg, 2002). A few examples include the neuroexcitatory domoic acid produced by the marine diatom Nitzschia pungens, responsible for shellfish poisoning (CitationDouglas et al., 1992), along with aldehyde compounds, produced by some planktonic microalgae such as Thalassiosira rotula and Skeletonema costatum, which are able to trigger abortive effects on copepods’ embryos and gametes (CitationMiralto et al., 1999). The effect of C. scutellum diatoms on H. inermis decapods seems not to be detrimental, but regulative from an ecological point of view. In fact, affecting the sex reversal of this shrimp by fasting the disruption of the androgenic gland, these diatoms guarantee an adequate number of females for the autumn mating period. It has been demonstrated that aldehydes are not involved in the diatom-induced apoptosis (CitationZupo et al., 2007; CitationNappo et al., 2009), but it cannot be excluded that other kinds of oxylipins could be responsible for the programmed cell death. Further investigations are required in order to clarify this point.
The biological assays on several cancer cell lines indicated that solid tumors, such as breast carcinoma, breast adenocarcinoma, and prostate carcinoma, were affected by C. scutellum more than hematologic malignancies (Burkitt’s lymphomas) and normal cells, suggesting a targeted toxic effect exhibited by the diatoms. As previously reported (CitationNappo et al., 2009), the chemical composition of C. scutellum diatoms consisted mainly of FAs, sterols and glycerides, and low amounts of N-containing metabolites, disaccharides and isoprenoid compounds. The separation of the microalgal metabolites into a diethyl ether extract, composed by less polar compounds, and a butanol extract, consisting in more polar compounds, allowed us to obtain a preliminary assignment of the apoptotic activity among the most apolar compounds. In fact, it was observed that the diethyl ether extract was more active than the butanol extract in inducing apoptosis in BT20 cells. These results led us to fractionate the diethyl ether extract with the purpose to test each fraction and determine the most effective one. The chemical composition of the obtained fractions 1–3 was established by GC-MS. It was observed that fraction 3 contained EPA, inter alia, a PUFA with known apoptotic activity (CitationHawkins et al., 1998; CitationGillis et al., 2002; CitationShirota et al., 2005). It has been reported that EPA exhibits many pharmacological activities, among which inhibition of some tumors both inducing apoptosis and suppressing COX-2 expression (CitationShirota et al., 2005). In addition, it is used for treating some neoplastic conditions as well as its association with some anti-cancer drugs (camptothecin inter alia) has been patented (US 2003054053, WO 2004012661, respectively).
It is not still clear whether EPA is the only factor involved in the diatom-induced apoptosis in BT20 or if there is a synergic association among more compounds. In addition, it is still unknown whether the same compound(s) is/are also responsible for triggering apoptosis in H. inermis androgenic gland. Furthermore, our investigation showed that fraction 3 was able to affect the cell cycle. In contrast to fraction 1 and fraction-2 treated cells, whose distribution in the various phases of the cell cycle remained unchanged with respect to the control, a decrease in BT20 cells at G2-M phase was observed in presence of fraction 3. These results suggested that the inhibition of the cell growth induced by fraction 3 could be partially due to apoptosis and, on the other hand, to an arrest in the progression of the cell cycle from S to G2-M.
Western blotting experiments were carried out in order to identify the caspase responsible for inducing apoptosis in BT20 cells. It is known that caspases-8 are the main initiator proteins of the apoptotic extrinsic pathway, while caspases 9 regulate the apoptotic intrinsic pathway. As hypothesized, the experiments demonstrated that caspase-8 was involved in the diatom-induced apoptosis. In fact, it was activated in presence of fraction 3, whereas its presence was less evident in both fraction-2- and Vepesid-treated populations. We demonstrated, in addition, that caspase-3, was another mediator of the proteolytic cascade triggered by C. scutellum since the cleaved/active form of caspase-3 was found only in those samples treated with fraction 3 and EPA after 6 h of incubation.
Conclusion
The present study has dealt with the potential applications of marine diatoms in pharmacology, in particular in the oncological field. This is a further confirmation that compounds with special ecological properties can exhibit interesting pharmacological activities as well. We have demonstrated that the diatom apoptotic effects are highly selective towards determined cell targets, as demonstrated by the higher response of solid tumors with respect to lymphomas and normal cells. The high selectivity of action is one of the main goals to be achieved in the anti-cancer drug discovery, since it allows reducing the strong side effects that characterize the traditional chemotherapies. In addition, we have localized the apoptotic activity in the apolar fraction, the diethyl ether extract, and in one of its fractions in particular, that containing the highest amount of EPA. It is still questioned, if EPA acts alone or in a synergic association with other compounds and whether both influence the sex reversal in H. inermis and the action on BT20 cells is due to the same compounds. The results afforded by our study underline again the importance of natural products in the current drug discovery and the need to continue investigating the pharmacological properties of secondary metabolites from natural sources.
Acknowledgements
We are grateful to all the other participants of the project, Prof. A. Sagi and his research team (Ben-Gurion University, Beer Sheva, Israel), and Mr. S. Taboada (CEAB-CSIC, Blanes, Spain). Thanks also to Dr. A. Marín (Serveis Cientificotècnics, University of Barcelona) for performing GC-MS analyses, and Mrs. M.P. Pistillo for providing BT20 cells.
Declaration of interest
The study was supported by the European Project PHARMAPOX (FP6-2003-NEST-A/STREP 4800). The authors report no conflicts of interest. The authors alone are responsible for the content and writing of the paper.
References
- Chajès V, Sattler W, Stranzl A, Kostner GM. (1995). Influence of n-3 fatty acids on the growth of human breast cancer cells in vitro: Relationship to peroxides and vitamin-E. Breast Cancer Res Treat, 34, 199–212.
- Douglas DJ, Ramsey UP, Walter JA, Wright JLC. (1992). Biosynthesis of the neurotoxin domoic acid by the marine diatom Nitzschia pungens forma multiseries, determined with [13C]-labelled precursors and nuclear magnetic resonance. J Chem Soc Chem Commun, 714–716.
- Gillis RC, Daley BJ, Enderson BL, Karlstad MD. (2002). Eicosapentaenoic acid and γ-linolenic acid induce apoptosis in HL-60 cells. j Surg Res, 107, 145–153.
- Gillis RC, Daley BJ, Enderson BL, Kestler DP, Karlstad MD. (2007). Regulation of apoptosis in eicosapentaenoic acid-treated HL-60 cells. j Surg Res, 137, 141–150.
- Hawkins RA, Sangster K, Arends MJ. (1998). Apoptotic death of pancreatic cancer cells induced by polyunsaturated fatty acids varies with double bond number and involves an oxidative mechanism. j Pathol, 185, 61–70.
- Ianora A, Poulet SA, Miralto A, Grottoli R. (1996). The diatom Thalassiosira rotula affects reproductive success in the copepod Acartia clausi. Mar Biol, 125, 279–286.
- Lin A-S, Shibano M, Nakagawa-Goto K, Tokuda H, Itokawa H, Morris-Natschke SL, Lee K-H. (2007). Cancer preventive agents. 7. Antitumor promoting effects of seven active flavonolignans from Milk Thistle (Silybum marianum) on Epstein-Barr Virus activation. Pharm Biol [Online]. Available at: http://www.informapharmascience.com/doi/abs/10.1080/13880200701585592. Accessed on 12 April 2009.
- Jimeno J. (2007). Yondelis® (trabectedin): Major clinical impact of a marine anti-cancer compound in the era of targeted therapies. 5th Eur Confer Mar Nat Prod, Ischia, Italy.
- Landsberg JH. (2002). The effects of harmful algal blooms on aquatic organisms. Res Fish Sci, 10, 113–390.
- Lowe SW, Lin AW. (2000). Apoptosis in cancer. Carcinogenesis, 21, 485–495.
- Mandal CC, Ghosh-Choudhury T, Yoneda T, Choudhury GG, Ghosh-Choudhury N. (2010). Fish oil prevents breast cancer cell metastasis to bone. Biochem Biophys Res Commun, 402, 602–607.
- Miralto A, Barone G, Romano G, Poulet SA, Ianora A, Russo LG, Buttino I, Mazzarella G, Laabir M, Cabrini M, Giacobbe MG. (1999). The insidious effect of diatoms on copepod reproduction. Nature, 402, 173–176.
- Nappo M, Berkov S, Codina C, Avila C, Messina P, Zupo V, Bastida J. (2009). Metabolite profiling of the benthic diatom Cocconeis scutellum. J Appl Phycol, 21, 295–306.
- Nappo M. (2010). Secondary metabolites from benthic organisms: Ecological and chemical aspects. PhD Thesis, University of Barcelona. Available at: http://www.tdx.cat/handle/10803/2627.
- Shaw BA, Andersen RJ, Harrison PJ. (1995). Feeding deterrence properties of apo-fucoxanthinoids from marine diatoms. I. Chemical structures of apo-fucoxanthinoids produced by Phaeodactylum tricornutum. Mar Biol, 124, 467–472.
- Shirota T, Haji S, Yamasaki M, Iwasaki T, Hidaka T, Takeyama Y, Shiozaki H, Ohyanagi H. (2005). Apoptosis in human pancreatic cancer cells induced by eicosapentaenoic acid. Nutrition, 21, 1010–1017.
- Vaux DL, Korsmeyer SJ. (1999). Cell death in development. Cell, 96, 245–254.
- Zupo V. (2000). Effect of microalgal food on the sex reversal of Hippolyte inermis (Crustacea: Decapoda). Mar Ecol Progr Ser, 201, 251–259.
- Zupo V, Messina P. (2007). How do dietary diatoms cause the sex reversal of the shrimp Hippolyte inermis Leach (Crustacea, Decapoda). Mar Biol, 151, 907–917.