Abstract
Context: Amla [Emblica officinalis Gaertn. (Euphorbiaceae)], a major constituent of several herbal formulations, is a well-known hepatoprotectant. Despite its extensive use, mechanistic understanding of its antioxidant action is rather limited.
Objective: In the current study, we investigated the effects of E. officinalis extracts (from dried fruits) on cellular oxidative state using a hepatocyte cell line (HepG2). We hypothesize that E. officinalis aqueous extracts have potency to modulate basal oxidative markers and enhance endogenous antioxidant defenses.
Materials and methods: Cells were incubated with aqueous extracts of E. officinalis (1–100 μg/ml) for varied time points (4–24 h) and biochemical markers of oxidative stress were determined in cell lysate.
Discussion: Aqueous extracts of E. officinalis at 100 μg/ml can significantly modulate the basal levels of oxidative markers and enhance antioxidant defenses of the cells.
Conclusions: Our findings clearly indicate the propensity of E. officinalis aqueous extracts to improve endogenous antioxidant defenses in HepG2 cells. Although further studies are required to assess their efficacy under experimentally induced oxidative, our data suggest that the hepatoprotective effects of E. officinalis reported earlier may be largely due to its potential to enhance the antioxidant defenses in vivo.
Results: Because E. officinalis up to 100 μg/ml concentrations had no effect on cell viability; it was considered noncytotoxic. Incubation with E. officinalis for 24 h resulted in significant diminution in the levels of lipid hydroperoxide (18–42%) and reactive oxygen species (11–29%). Furthermore; E. officinalis increased the levels of glutathione (GSH; 18–32%); antioxidant capacity (19–31%); and activities of antioxidant enzymes (superoxide dismutase; 25–41%; catalase; 39–50%; GSH peroxidase; 20–35%; GSH reductase; 26–35%; and GSH S-transferase; 12–30%).
Introduction
Amla [Emblica officinalis, Gaertn. (Euphorbiaceae)], commonly referred as Indian gooseberry, is extensively used in Ayurveda (traditional Indian healthcare system) for treatment of several chronic ailments. The fruits have been reported to contain constituents with various biological activities and form the main constituent of rasayana (ayurvedic herbal drug to promote health and longevity). Experiments conducted with fruits of E. officinalis have shown that they possess hypolipidemic (CitationAnila & Vijayalakshmi, 2002), antidiabetic (CitationRao et al., 2005), anti-inflammatory (CitationSai Ram et al., 2002), and antiaging activity (CitationYokozawa et al., 2007).
Increase in free radical generation or an impaired endogenous antioxidant mechanism is known to result in a state of oxidative stress (OS), which is one of the important factors implicated in metabolic syndrome (MS). OS is also prominent in nonalcoholic fatty liver disease (NAFLD), a spectrum of diseases of the liver ranging from steatosis to cirrhosis. NAFLD is gaining lot of interest because of its close association with MS and occurs in 14–30% of general populations across all age groups and ethnicities (CitationKotronen & Yki-Järvinen, 2008). Lipotoxicity, OS, cytokines, and other proinflammatory mediators may all play a role in the mechanisms of disease progression and liver damage (CitationVidela et al., 2004). There is an ever increasing need for therapeutic agents to protect liver from such damage by reducing OS and thereby improve its functions.
Few studies in rodent models have demonstrated efficacy of E. officinalis in reversal of hepatotoxicity induced by dimethyl hydrazine (CitationAnilakumar et al., 2007); ethanol (CitationPramyothin et al., 2006); antituberculosis drugs (CitationTasduq et al., 2005), and CCl4 (CitationSultana et al., 2005). Although the underlying basis of such efficacy of E. officinalis is largely attributed to its antioxidant effect, mechanistic approaches to understand the efficacy of aqueous extract in cell models are rather limited. Hence, we set out to assess the potency of E. officinalis extract to modulate oxidative status using cell models such as HepG2 cells. In this study, to systematically investigate the effect of E. officinalis on cellular oxidative state, we focused on the effects of E. officinalis on: i) levels of basal oxidative markers, and ii) activities of endogenous antioxidant enzymes in HepG2 cells. We hypothesized that aqueous extracts of E. officinalis can significantly attenuate basal oxidative markers at cellular level by scavenging free radicals and enhance endogenous antioxidant defenses. We consider that an understanding of these two aspects of antioxidant effects would be helpful in gaining insights into the mechanistic basis of efficacy of E. officinalis to be used as preventive or therapeutic against various chronic ailments.
Materials and methods
Materials
Aqueous extract of E. officinalis was purchased from Natural Remedies, Bangalore, India, which is prepared as follows. The sun-dried fruits of E. officinalis were deseeded, ground to fine powder, and sieved through an 80-mesh sieve. The sieved powder was extracted in water in a ratio of 1:8 by boiling for 4 h. The extracts were subsequently filtered through four layers of muslin cloth, and filtrates were spray dried.
For preparing aqueous solution of E. officinalis extracts to treat the cells, dried extract powder (500 mg) was boiled in distilled water (10 ml) for 10 min at 100°C and then centrifuged at 500g for 15 min. The supernatants were transferred to microcentrifuge tubes and stored at −20°C. The amount of total soluble solids in supernatant was measured using gravimetric analysis and used as the basis to define the E. officinalis concentrations in experiments.
Characterization of E. officinalis extracts
The consistency of composition of E. officinalis extracts across four separate preparations was checked for compliance to monograph in Indian Pharmacopoeia (CitationIndian Pharmacopeia Commission, 2007) and were determined in terms of their spectral [infrared (IR) and H1 nuclear magnetic resonance (H1 NMR)] and chemical (high-performance liquid chromatography) profile. The IR spectra of E. officinalis extract was taken in an IR spectrophotometer (Perkin Elmer Spectrum 1) in potassium bromide pellets, whereas H1 NMR spectra were taken in 20 mg/ml of D2O in Bruker 600 MHz facility. IR (Supplementary Figure a) and H1 NMR profiles (phenolic and glycoside region) of four separate preparations of E. officinalis extracts were found to be similar (Figures a and b).
The chemical profiles of extracts were checked in a Shimadzu High-Performance Liquid chromatographic (HPLC) system equipped with LC-10A pump with SPD-M 10Avp Photo Diode Array Detector. The HPLC elution profile of four separate E. officinalis extract preparations matched and showed similar amounts of gallic acid and ellagic acid (Figures a and b). The E. officinalis extract used in this study contained total polyphenols equivalent to 33.6% w/w gallic acid and 10.8% w/w total tannins.
Cell line
The human hepatocyte cell line (HepG2) was purchased from American Type Cell Culture (ATCC, Manassas, VA). HepG2 cells were cultured in Dulbecco’s Modified Eagle’s Medium (Sigma, Bangalore India) containing 10% heat-inactivated fetal bovine serum (Gibco, NJ), 100 units/ml of penicillin, and 100 μg/ml of streptomycin. Cells were grown at 37°C in humidified air/CO2 (19:1) atmosphere and harvested at a logarithmic growth. The culture medium was changed twice every week, and the cells were subcultured at 1:3 ratios twice a week using 0.5% trypsin and 0.2% ethylenediaminetetraacetic acid in Ca2+, Mg2+ free phosphate-buffered saline (PBS).
Treating cells with aqueous extract of E. officinalis
HepG2 cells were seeded at a density of 105 was in six well plates and grown to confluence. Media of the confluent cells were replaced with fresh media and incubated along with different concentrations of E. officinalis aqueous extracts (0, 5, 10, 20, 50, and 100 μg/ml). Cells were harvested at varied time points (4, 8, 12, 16, 20, and 24 h). Each treatment was conducted in three replicates and repeated thrice. For testing the effects on various antioxidant parameters and antioxidant enzyme activities, three independent concentrations, low (25 μg/ml), intermediate (50 μg/ml), and highest (100 μg/ml), of E. officinalis extract was used.
Preparation of cytosolic extracts
At the defined time point, media was removed and cells were washed twice with ice-cold PBS (0.1 M PBS, pH 7.4). Cells were then harvested in lysis buffer (PBS with 0.1% triton X-100) using a cell scrapper. Later, cells were subjected to sonication for 5 s (pulse-10; power-30) keeping the vial containing the cells in ice bucket. After sonication, cells were centrifuged at 8000g for 15 min. Supernatants were stored in −80°C for further analysis. Separate aliquots were used for each parameter to avoid repeated freeze thaw cycle. Protein concentration in the cell cytosol was determined according to the method of CitationBradford (1976), using the “protein determination kit” from the Cayman Chemical Company (CCC), US.
Lactate dehydrogenase cytotoxicity assay
Lactate dehydrogenase (LDH) assay kit from CCC measures LDH activity using a coupled two-step reaction. LDH catalyzes the reduction of NAD+ to NADH and H+ by oxidation of lactate to pyruvate in the first step. In the second step, diaphorase uses the newly formed NADH and H+ to catalyze the reduction of a tetrazolium salt 2-(4-Indophenyl)-3-(4-nitrophenyl)-5-phenyl-2H-tetrazolium chloride ((INT)) to formazan, which is proportional to the amount of LDH released into the culture medium as a result of cytotoxicity (CitationWolterbeek & van der Meer, 2005). For cytotoxicity assay, cells were seeded in a 96-well plate at a density of 105 cells/well in 120 μl of serum-free culture medium with or without E. officinalis (0–4 mg/ml) for various time points (2–48 h). Experiments were performed in quadruplets for each concentration and time points.
MTT cell proliferation assay
In this assay, 3-(4, 5-dimethyl-2-thiazolyl)-2, 5-diphenyl-2H-tetrazolium bromide (MTT) intake into the cells is facilitated through the plasma membrane potential and is reduced to formazan by intracellular NAD(P)H-oxidoreductases (CitationFrancoeur & Assalian, 1996). Cells were seeded in a 96-well plate at a density of 105 cells/well in 100 μl of culture medium with or without E. officinalis (0.1–4 mg/ml) for various time points (2–48 h). Experiments were performed in quadruplets for each concentration and time points. After the end of desired time of incubation, 10 μl of MTT mixture was added to each well and allowed to incubate further for 4 h at 37°C in a CO2 incubator. After the incubation, culture medium was carefully removed by aspiration. Formazan produced in the cells was dissolved with 100 μl of crystal-dissolving solution and resultant purple color was read at 570 nm using a microplate reader.
Measurement of lipid hydroperoxides
Lipid hydroperoxides (LHPs) were measured with CCC’s LHP assay kit, which measures hydroperoxides based on its redox reaction with ferrous ions. Hydroperoxides, being highly unstable, react readily with ferrous ions to produce ferric ions. The resulting ferric ions are detected using thiocyanate ions as the chromogen at 500 nm (Mihaljević et al., 1996).
Measurement of total antioxidant capacity
Total antioxidant capacity (TAC) of the cells is measured as the ability of antioxidants in the cell lysate to inhibit the oxidation of ABTS (2,2-azino-di[3-ethylbenzthiazoline sulfonate]) to ABTS+ by metmyoglobin, which is monitored at 750 nm. The suppression of the absorbance at 750 nm by antioxidants in cells is proportional to their concentration and is compared to Trolox (water-soluble tocopherol analog) using CCC’s TAC kit (CitationRice-Evans & Miller, 1994). Measurement of reactive oxygen species
The method uses the fluorescent property of dichlorofluorescein (DCFH), which fluoresces after its reaction with reactive oxygen species (ROS). The nonfluorescent dye dichlorofluorescein diacetate (DCFH-DA) is preincubated for 15 min at room temperature to allow it to be incorporated into membrane-bound vesicle and the diacetate group is cleaved by esterases. After 30 min, the conversion of DCFH to the fluorescent product DCF was measured using a fluorescence spectrophotometer (excitation, 485 nm; emission, 530 nm). Background fluorescence (autofluorescence) was corrected by the inclusion of parallel blanks. ROS production was quantified from a DCF standard curve, and results were expressed as pmol DCF formed per milligram protein per minute (CitationLeBel et al., 1992).
Measurement of reduced glutathione
Glutathione (GSH) levels in cells are quantified by optimized enzymatic recycling method, using GSH reductase (GR) and CCC’s GSH assay kit. The sulfhydryl group of GSH reacts with DTNB (5,5-dithiobis-2-nitrobenzoic acid, Elman’s reagent) and produces TNB (5-thio-2-nitrobenzoic acid), a yellow compound. The mixed disulfide, GSTNB that is concomitantly produced, is reduced by GR to recycle the GSH and produce more TNB. The rate of TNB production is directly proportional to this recycling reaction, which in turn is directly proportional to the concentration of GSH in sample (CitationEyer & Podhradský, 1986).
Measurement of activities of antioxidant enzymes
Catalase (CAT).
Catalase assay kit (from CCC) is based on the reaction of the enzyme with methanol in the presence of optimal concentration of H2O2. The formaldehyde produced is measured spectrophotometrically with purpald (4-amino-3-hydrazino-5-mercapto-1,2,4-triazole) as the chromogen. Purpald specifically forms a bicyclic heterocycle with aldehydes, which on oxidation changes from colorless to purple (CitationJohansson & Borg, 1988).
Superoxide dismutase.
Superoxide dismutase (SOD) assay kit (from CCC) uses a tetrazolium salt for detection of superoxide radicals generated by xanthine oxidase and hypoxanthine. One unit of SOD is defined as the amount of enzyme needed to exhibit 50% dismutation of the superoxide radical (CitationFlohé & Otting, 1984).
GSH peroxidase.
The CCC’s GSH peroxidase (GPX) assay measures GPX activity indirectly by a coupled reaction with GR. Oxidized GSH (GSSG), produced on reduction of hydroperoxide by GPX, is recycled to its reduced state by GR and NADPH. The oxidation of NADPH to NADP+ is accompanied by a decrease in absorbance at 340 nm (CitationFlohé & Günzler, 1984).
GSH reductase.
The CCC’s GR kit measures GR activity by measuring the rate of NADPH oxidation. The oxidation of NADPH to NADP+ is accompanied by a decrease in absorbance at 340 nm and is directly proportional to the GR activity in the sample (CitationCarlberg & Mannervik, 1985).
GSH S-transferase.
The CCC’s GSH S-transferase (GST) assay kit measures total GST activity by measuring the conjugation of CDNB (1-chloro-2,4-dinitrobenzene) with GSH. The conjugation is accompanied by an increase in absorbance at 340 nm. The rate of increase is directly proportional to GST activity in the sample (CitationWarholm et al., 1985).
Statistical analysis
All values are expressed as the mean ± SD of nine measurements. Statistical analysis was performed using the Holm–Sidak test, which can be used for both pairwise comparisons and comparisons versus a control group. This test is more powerful than the Turkey and Bonferroni test and is recommended as the first-line procedure for most multiple comparison testing (Sigma Stat 3.5, Systat Software, Point Richmond, CA). A p value <0.05 was considered statistically significant.
Results
Effect of E. officinalis on cell viability
The percent of viability of cells when treated with different concentration of E. officinalis measured as formazan formed from MTT is shown in . E. officinalis at a concentration of up to 100 μg/ml did not affect the cell viability. However, E. officinalis at a concentration of ≥ 250 μg/ml caused a significant decrease in viability of HepG2 cells (21–62%). At the highest concentration (4 mg/ml) tested in our experiment, the percent viability was reduced to 38%.
Figure 1. Effect of Emblica officinalis on cell proliferation and cytotoxicity in HepG2 cells. (a) Cell proliferation assay as measured by MTT reduction. (b) Lactate dehydrogenase (LDH) leakage into culture medium; HepG2 cells were treated with aqueous extracts of E. officinalis (0–4 mg/ml) for various time points (2–48 h) and assayed for its effects on cytotoxicity and cell proliferation. Values are mean ± SD. Data analyzed by Holm–Sidak test, *p < 0.05. The number against E. officinalis denotes concentration in micrograms per milliliter.
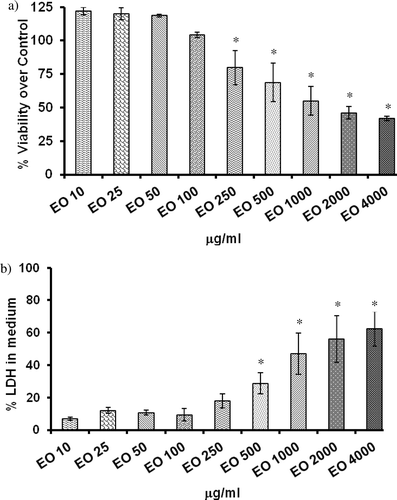
Cytotoxic effect of E. officinalis on HepG2 cells
The cytotoxic effect of E. officinalis was investigated using LDH leakage assay, and results are shown in . A 24-h treatment with 10, 25, 50, and 100 μg/ml of E. officinalis failed to evoke an increase in LDH activity in the cell culture medium, indicating these doses are not cytotoxic. However, E. officinalis at the doses above 500 μg/ml caused a significant and dose-dependent increase of LDH leakage. Considering these results, the noncytotoxic doses of E. officinalis were selected to study the effect of E. officinalis on biochemical markers of OS in HepG2 cells.
Effect of E. officinalis on basal LHP
Data on basal levels of LHP’s in control and E. officinalis – treated HepG2 cells are shown in . Basal LHPs varied according to time of sampling. While at 4 h, LHP’s were 5.42 ± 0.46, which increased to 5.98 ± 0.73 (12 h) and later decreased to 5.02 ± 1.13 at 24 h. In general, there was concentration- and time-dependent decrease in the basal LHPs in the cytosol of HepG2 cells incubated with different concentration of E. officinalis for varied length of time. E. officinalis at a concentration of 25 μg/ml did not alter the basal LHPs at any observed time points. However, at higher concentration (50 μg/ml), E. officinalis offered significant protection (18–42%) at all time points. Similar trend of protection was also observed with the highest (100 μg/ml) concentration of E. officinalis.
Figure 2. Effect of Emblica officinalis on (a) levels of lipid hydroperoxides (LHPs), and (b) pattern of generation of reactive oxygen species (ROS) in HepG2 cells incubated for various time points (4–24 h). Values are mean ± SD. Data analyzed by Holm–Sidak test, *p < 0.05. The number against E. officinalis denotes concentration in micrograms per milliliter.
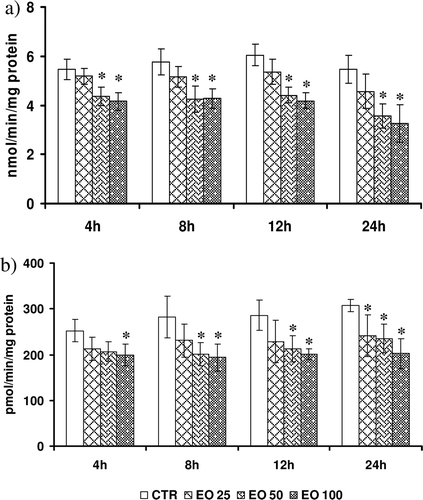
Effect of E. officinalis on basal ROS generation
The generation of ROS in the cytosol of both control and E. officinalis–treated HepG2 cells are presented in . The basal ROS levels in the HepG2 cytosol at 4 h were 264.2 ± 1.9, which increased to 308 ± 19 at 24 h. The lower dose did not alter the basal ROS levels much (8–18%) at any of our experimental time points except at 24 h. A significant decline (11–29%) in the ROS generation was observed at all time points with the highest concentration. The highest protection against ROS generation was observed at 24 h (29%) with 100 μg/ml E. officinalis.
Modulation of basal levels of TAC by E. officinalis
Data on the basal antioxidant capacity of HepG2 cells expressed as millimolar Trolox equivalent is presented in . The basal TAC varied from 2.42 ± 0.21 (4 h) to 3.64 ± 0.47 (24 h). E. officinalis at a lower concentration significantly enhanced TAC of HepG2 cells at 4 and 24 h (19 and 31%). However, intermediate and highest concentrations of E. officinalis evoked an increase in TAC at all time points significantly (23–44%).
Figure 3. Effect of Emblica officinalis on (a) total antioxidant capacity of cells, and (b) levels of reduced glutathione in HepG2 cells incubated for various time points (4–24 h). Values are mean ± SD. Data analyzed by Holm–Sidak test, *p < 0.05. The number against E. officinalis denotes concentration in micrograms per milliliter.
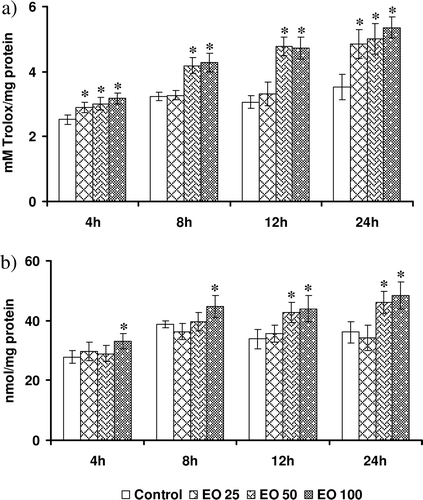
Alterations in the GSH levels
The intracellular GSH levels serve as an index of nonenzymatic antioxidant defenses. We measured levels of the GSH in cells treated with different concentrations of E. officinalis, and the data are depicted in . Lower doses of E. officinalis did not alter the GSH levels at any of the time points. However, intermediate and highest concentrations increased the GSH levels at 12 and 24 h (20–45%). Highest concentrations of E. officinalis also increased GSH at 8 h.
Alterations in the activity of antioxidant enzymes by E. officinalis
Modulation in the SOD activity by E. officinalis in HepG2 cells is shown in . Basal SOD activity did not vary over time, and it ranged between 30.51 ± 0.38 (4 h) and 36.55 ± 0.65 (24 h) U/min/mg protein. E. officinalis at a concentration of 25 μg/ml failed to increase SOD activity at all time points tested. However, it was found to be increased by 20–33% in response to intermediate concentration. E. officinalis at highest concentration enhanced the SOD activity at all tested time points ranging from 25% at 4 h to 41% at 24 h.The catalase activities expressed as nanomoles per minute per milligram of protein in the cytosol of HepG2 cells incubated with E. officinalis and controls are shown in . The basal enzyme activity varied from 90.2 ± 9.4 at 4 h to 146.4 ± 10.6 at 24 h. After 4 h of incubation, lower and intermediate concentrations of E. officinalis failed to enhance the catalytic activity. However, highest concentrations of all treatments and intermediate concentrations after 8 h increased the catalase activity significantly (33%).
Figure 4. Effect of Emblica officinalis on the activities of endogenous antioxidant enzymes (a) superoxide dismutase (SOD), and (b) catalase in HepG2 cells incubated for various time points (4–24 h). Values are mean ± SD. Data analyzed by Holm–Sidak test, *p < 0.05. The number against E. officinalis denotes concentration in micrograms per milliliter.
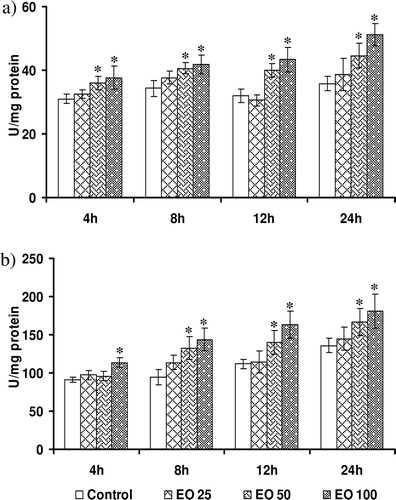
Data on the effect of E. officinalis on the activities of GSH-based antioxidant enzymes are shown in . In general, lower dose of E. officinalis failed to evoke a significant increase in any of the GSH-based antioxidant enzymes at all the tested time points. Although intermediate concentration enhanced (20%) GPX activity only at 8 and 24 h, it enhanced GR activity at all time points, except 4 h. However, GST activity was enhanced only at 24 h with intermediate concentration. Highest concentration of E. officinalis could enhance the activities of all the tested GSH-based enzymes at all time points. Although it enhanced GPX activity by 25–30%, GR activity was enhanced by 20–40%. The increase in GST activity was less and limited to 15–25% with highest concentration of E. officinalis.
Figure 5. Modulatory effect of Emblica officinalis on (a) glutathione peroxidase (GPX), (b) glutathione reductase (GR), and (c) glutathione S-transferase (GST) in HepG2 cells incubated for various time points (4–24h). Values are mean ± SD. Data analyzed by Holm–Sidak test, *p < 0.05. The number against E. officinalis denotes concentration in micrograms per milliliter.
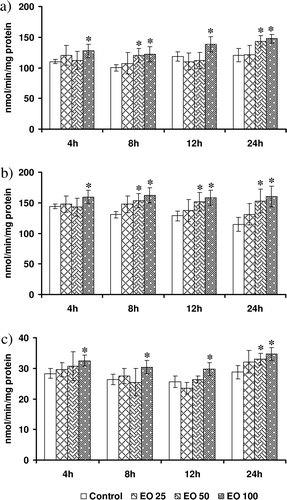
Discussion
Amla, a major constituent of several herbal formulations, is shown to improve general health and is a well-known hepatoprotectant. Although the antioxidant effect of E. officinalis is considered to be central to the efficacy of E. officinalis, mechanistic data supporting such efficacy in cell models are limited. The extract of E. officinalis is shown to exhibit antioxidant properties in chemical models of antioxidant assay system and found to be a potent scavenger of hydroxyl and superoxide radicals in vitro (CitationSabu & Kuttan, 2002). Antioxidant properties of E. officinalis have been explored in a few studies involving rodent models of hepatotoxicity (CitationAnilakumar et al., 2007; CitationBhattacharya et al., 2002; CitationRajak et al., 2004). The nature of E. officinalis extract and the monitored tissue in these studies were, however, varied. Earlier few studies, which have attempted to study the antioxidant effect of E. officinalis, used potassium metabisulphite, methanol, and hydroaqueous extracts to study the effect in rat liver (CitationAnilakumar et al., 2007), rat heart (CitationRajak et al., 2004) and brain, and rat heart (CitationBhattacharya et al., 2002). Despite many therapeutic effects of E. officinalis, data on the putative antioxidant effect of E. officinalis and its propensity to modulate the activity of antioxidant enzymes at cellular levels are limited. Accordingly, we examined the effect of E. officinalis further in a liver cell (HepG2)–based model system by monitoring a broad range of parameters to determine the status of oxidative markers, TAC, and activity of antioxidant enzymes. HepG2 is a well-differentiated transformed cell line in which steady-state functioning of the antioxidant defenses is relatively higher than that in hepatocytes and other nontransformed cells, which makes it a better and more sensitive system for studying the variations of responses to different conditions (Alia et al., 2006).
In the current study, aqueous extracts of E. officinalis at a concentration of ≥250 μg/ml significantly decreased the cell viability in HepG2 cells. Earlier, several workers have shown that polyphenolic compounds can induce apoptosis in human cancer cells (CitationSakagami et al., 1995, Citation1999). HepG2 (hepatocellular carcinoma), being a cancerous cell line, is susceptible to apoptotic effect of these compounds in the E. officinalis extracts. Recently, aqueous extracts of E. officinalis were shown to induce apoptosis in several cancer cell lines (Hela, A549, HepG2, MRC5, etc.) at varying concentrations (CitationNgamkitidechalul et al., 2010). Studying the antitumor effects of E. officinalis, CitationNgamkitidechalul et al. (2010) found that LC50 of E. officinalis in HepG2 was 350 μg/ml and total growth inhibition was 180 μg/ml. Although the researchers have used aqueous extracts, their polyphenol content is high (43%) compared with the extract used by us. This could be due to the variations in the method used by earlier workers to quantify. The Farnworth method used to analyze alkaloids in an earlier study gives a crude estimate compared with the more refined estimate given by HPLC and NMR (used in our study).
Significant diminution in the ROS levels on exposure to 50 and 100 μg of E. officinalis at all the tested time points indicate that bioactive ingredients present in E. officinalis have stoichiometrically scavenged the ROS or facilitated the cells to counter the basal ROS production more efficiently. Our data corroborate the antioxidant and free radical activity of E. officinalis extracts demonstrated earlier in cell-free in vitro assays (CitationBhattacharya et al., 1999). However, direct evaluation of intracellular ROS after treatment of human cell lines with E. officinalis has not been studied. Although there are various methods to assess oxidative damage of cells, a direct evaluation of the ROS yields a good indication of the status of oxidative markers in living cells (CitationLeBel et al., 1992). To the best of our knowledge, this is the first report to show the efficacy of E. officinalis to reduce the ROS generation in a concentration-dependent manner in a cell model by using a DCF-based assay system.
In the current model, E. officinalis significantly diminished the levels of LHP as evident by lower levels of LHP. Earlier few workers have consistently shown that E. officinalis reduced pro-oxidant-induced lipid peroxidation (LPO) in various tissues (CitationAnilakumar et al., 2007; CitationSultana et al., 2005; CitationTasduq et al., 2005). Previous studies reported no change in basal LPO among rats administered aqueous E. officinalis extract in hepatic tissues (CitationAnilakumar et al., 2007), tannin-enriched E. officinalis fractions in heart and brain of rats (CitationBhattacharya et al., 2000). Furthermore, ethanol extracts of E. officinalis (100 μg/ml) also failed to modulate the basal LPO levels in rat lymphocytes when incubated for 18 h (CitationSai Ram et al., 2002). However, the methanol extract significantly decreased LPO in rat heart (CitationRajak et al., 2004). Considering nonspecific and lower sensitivity of thiobarbituric acid reactive substances (TBARS), we have used a more sensitive method to measure LPO and demonstrate that basal LHP has been diminished significantly by aqueous extract of E. officinalis.
The overall antioxidant capacity may provide more relevant biological information compared with that obtained by the measurement of individual components, because it considers the cumulative effect of all antioxidants present in the cell cytosol (CitationRice-Evans & Miller, 1994). Although TAC has been used as a valuable marker to study the effects of many other phytonutrients in cell models, there are no studies that used this parameter to study the antioxidant effect of E. officinalis in any tissues or cells. We report that E. officinalis at higher concentrations (50 and 100 μg) increased the antioxidant capacity of cells.
In the current study, we found significantly enhanced GSH levels in cells at higher concentrations of E. officinalis, suggesting its potency to increase the antioxidant molecule. An increase in GSH concentration prepares the cell against a potential oxidative insult (CitationAlia et al., 2006). Our data are consistent with earlier observations in which rats fed with E. officinalis-supplemnted diet (for 12 weeks) showed significant increase in hepatic GSH levels (CitationAnilakumar et al. (2007). GSH (reduced) levels were also unaltered in rat lymphocytes incubated with 100 μg/ml ethanol extract of E. officinalis (CitationSai Ram et al., 2002). The variations in the effect of E. officinalis in terms of modulating the basal OS parameters in rat tissues may be due to the fact that different extracts (methanol, aqueous, ethanol, petroleum ether, and potassium metabisulphite) have been used by various authors and also the target tissues were different in which these parameters were assayed.
The activity levels of SOD present in cellular and extracellular environments are crucial for the prevention of diseases linked to OS. The reaction catalyzed by SOD is extremely fast: having a turnover of 2 × 109 M/s, and the presence of sufficient amounts of enzyme in cells and tissues typically keeps the concentration of superoxide (O2−.) low (CitationHalliwell, 1996). In our study, E. officinalis at concentrations of 50 and 100 μg consistently increased the activity of SOD. This observation is consistent with previous studies that have reported similar increase in SOD activity in various tissues of rat after administration of E. officinalis (CitationAnilakumar et al., 2007; CitationBhattacharya et al., 2002; CitationRajak et al., 2004).
The regulation of antioxidant enzymes forms a system of mutual protection, where superoxide can inactivate both catalase and GPX, whereas hydrogen peroxide can inactivate the cytosolic SOD. Thus, in a system experiencing substantial OS, the entire group of antioxidant enzymes may be subject to partial inactivation by the unscavenged superoxide and hydrogen peroxide. Thus, a simultaneous increase in catalase activity coupled with increase in SOD activity is shown to be more beneficial (CitationNelson et al., 2006). For the same reason, the activities of GR may have been unchanged. GR catalyze the conversion of oxidized GSH to reduced GSH, and activity is increased when there is increased concentration of GSSG or decrease in GSH. Because E. officinalis was able to increase the GSH concentration, GR activities were unaffected. These results become even more relevant when viewed in the light of a fundamentally new approach to antioxidant therapy, which emphasizes on sustained long-term induction of endogenous antioxidant enzymes to be more beneficial rather than the conventional scavenging of free radicals at cellular levels (CitationNelson et al., 2006). Intervention strategies with chronic administration of herbs such as E. officinalis, which augment major cellular endogenous defense systems, may be considered as more acceptable therapeutic approach.
Phytochemical evaluation of E. officinalis showed the presence of tannins emblicanin-A, emblicanin-B, punigluconin, pedunculagin, trigalloyl glucose, phyllembillin, phyllemblic acid flavonoids such as quercetin and its glycoside derivative rutin, ellagic acid, gallic acid, ferulic acid, and ascorbic acid (CitationBhattacharya et al., 2002). Enhanced gene expression of SOD, GPX and catalase by quercetin (CitationAlia et al., 2006) has been reported. Quercetin, being one of the major components in E. officinalis, may underlie the increased activity of antioxidant enzymes.
The liver is a major regulator of metabolite flow in the body regulating internal chemical environment and has great capacity to remove many toxic materials from circulation and release them or their products at a moderate rate. Among several diseases and disorder affecting the liver, NAFLD-related to insulin resistance is gaining a lot of interest, given its close association with MS (CitationKotronen & Yki-Järvinen, 2008). OS caused by increased β-oxidation during insulin resistance seems to be prominent in NAFLD and has been identified as a therapeutic target for antioxidants (CitationVidela et al., 2004). E. officinalis with its propensity to enhance basal antioxidant capacity and activity of antioxidant enzymes in HepG2 cells may be better exploited under such conditions.
Collectively, our findings suggest that E. officinalis exerts significant antioxidant effect in HepG2 cells by reducing generation of ROS and LHP and enhancement of reduced GSH levels, which may be effective under pro-oxidant exposure conditions in the cell. These effects may be brought about by the observed increase in the activity of antioxidant enzymes such as SOD and catalase, leading to a sustained and faster dissipation of superoxide and hydrogen peroxide.
Supplementary Material
Download PDF (428.4 KB)Acknowledgements
The authors are grateful to Dr. Vilas Sinkar for critically reviewing the manuscript; Dr. Shovan Ganguly for his support; Ms. Aparna Damle for her assistance with cell culture; and Mr. Deepak Mhasavade for analytical characterization of E. officinalis extracts.
Declaration of interest
The authors report no conflict of interests.
References
- Alía M, Mateos R, Ramos S, Lecumberri E, Bravo L, Goya L. (2006). Influence of quercetin and rutin on growth and antioxidant defense system of a human hepatoma cell line (HepG2). Eur J Nutr, 45, 19–28.
- Anila L, Vijayalakshmi NR. (2002). Flavonoids from Emblica officinalis and Mangifera indica-effectiveness for dyslipidemia. J Ethnopharmacol, 79, 81–87.
- Anilakumar KR, Nagaraj NS, Santhanam K. (2007). Reduction of hexachlorocyclohexane-induced oxidative stress and cytotoxicity in rat liver by Emblica officinalis gaertn. Indian J Exp Biol, 45, 450–454.
- Bhattacharya A, Chatterjee A, Ghosal S, Bhattacharya SK. (1999). Antioxidant activity of active tannoid principles of Emblica officinalis (amla). Indian J Exp Biol, 37, 676–680.
- Bhattacharya A, Ghosal S, Bhattacharya SK. (2000). Antioxidant activity of tannoid principles of Emblica officinalis (amla) in chronic stress induced changes in rat brain. Indian J Exp Biol, 38, 877–880.
- Bhattacharya SK, Bhattacharya A, Sairam K, Ghosal S. (2002). Effect of bioactive tannoid principles of Emblica officinalis on ischemia-reperfusion-induced oxidative stress in rat heart. Phytomedicine, 9, 171–174.
- Bradford MM. (1976). A rapid and sensitive method for the quantitation of microgram quantities of protein utilizing the principle of protein-dye binding. Anal Biochem, 72, 248–254.
- Carlberg I, Mannervik B. (1985). Glutathione reductase. Meth Enzymol, 113, 484–490.
- Eyer P, Podhradský D. (1986). Evaluation of the micromethod for determination of glutathione using enzymatic cycling and Ellman’s reagent. Anal Biochem, 153, 57–66.
- Flohé L, Günzler WA. (1984). Assays of glutathione peroxidase. Meth Enzymol, 105, 114–121.
- Flohé L, Otting F. (1984). Superoxide dismutase assays. Meth Enzymol, 105, 93–104.
- Francoeur AM, Assalian A. (1996). Microcat: A novel cell proliferation and cytotoxicity assay based on WST-1. Biochemica, 3,19–25.
- Halliwell B. (1996). Antioxidants in human health and disease. Annu Rev Nutr, 16, 33–50.
- Indian Pharmacopeia Commission. (2007). Amalaki monograph. Indian Pharmacopoeia 3:2018.
- Johansson LH, Borg LA. (1988). A spectrophotometric method for determination of catalase activity in small tissue samples. Anal Biochem, 174, 331–336.
- Kotronen A, Yki-Järvinen H. (2008). Fatty liver: a novel component of the metabolic syndrome. Arterioscler Thromb Vasc Biol, 28, 27–38.
- LeBel CP, Ischiropoulos H, Bondy SC. (1992). Evaluation of the probe 2′,7′-dichlorofluorescin as an indicator of reactive oxygen species formation and oxidative stress. Chem Res Toxicol, 5, 227–231.
- Mihaljevic B, Katusin-Razem B, Razem D. (1996). The reevaluation of the ferric thiocyanate assay for lipid hydroperoxides with special considerations of the mechanistic aspects of the response. Free Radic Biol Med, 21, 53–63.
- Ngamkitidechakul C, Jaijoy K, Hansakul P, Soonthornchareonnon N, Sireeratawong S. (2010). Antitumour effects of Phyllanthus emblica L.: induction of cancer cell apoptosis and inhibition of in vivo tumour promotion and in vitro invasion of human cancer cells. Phytother Res, 24, 1405–1413.
- Nelson SK, Bose SK, Grunwald GK, Myhill P, McCord JM. (2006). The induction of human superoxide dismutase and catalase in vivo: a fundamentally new approach to antioxidant therapy. Free Radic Biol Med, 40, 341–347.
- Pramyothin P, Samosorn P, Poungshompoo S, Chaichantipyuth C. (2006). The protective effects of Phyllanthus emblica Linn. extract on ethanol induced rat hepatic injury. J Ethnopharmacol, 107, 361–364.
- Rao TP, Sakaguchi N, Juneja LR, Wada E, Yokozawa T. (2005). Amla (Emblica officinalis Gaertn.) extracts reduce oxidative stress in streptozotocin-induced diabetic rats. J Med Food, 8, 362–368.
- Rajak S, Banerjee SK, Sood S, Dinda AK, Gupta YK, Gupta SK, Maulik SK. (2004). Emblica officinalis causes myocardial adaptation and protects against oxidative stress in ischemic-reperfusion injury in rats. Phytother Res, 18, 54–60.
- Rice-Evans C, Miller NJ. (1994). Total antioxidant status in plasma and body fluids. Meth Enzymol, 234, 279–293.
- Sabu MC, Kuttan R. (2002). Anti-diabetic activity of medicinal plants and its relationship with their antioxidant property. J Ethnopharmacol, 81, 155–160.
- Sai Ram M, Neetu D, Yogesh B, Anju B, Dipti P, Pauline T, Sharma SK, Sarada SK, Ilavazhagan G, Kumar D, Selvamurthy W. (2002). Cyto-protective and immunomodulating properties of Amla (Emblica officinalis) on lymphocytes: an in-vitro study. J Ethnopharmacol, 81, 5–10.
- Sakagami H, Kuribayashi N, Iida M, Sakagami T, Takeda M, Fukuchi K, Gomi K, Ohata H, Momose K, Kawazoe Y. (1995). Induction of DNA fragmentation by tannin- and lignin-related substances. Anticancer Res, 15, 2121–2128.
- Sakagami H, Kishino K, Kobayashi M, Hashimoto K, Iida S, Shimetani S, Nakamura Y, Takahashi K, Ikarashi T, Fukamachi H. (1999). Selective antibacterial and apoptosis-modulating activities of mastic. In Vivo, 23, 215–223.
- Sultana S, Ahmad S, Khan N, Jahangir T. (2005). Effect of Emblica officinalis (Gaertn) on CCl4 induced hepatic toxicity and DNA synthesis in Wistar rats. Indian J Exp Biol, 43, 430–436.
- Tasduq SA, Kaisar P, Gupta DK, Kapahi BK, Maheshwari HS, Jyotsna S, Johri RK. (2005). Protective effect of a 50% hydroalcoholic fruit extract of Emblica officinalis against anti-tuberculosis drugs induced liver toxicity. Phytother Res, 19, 193–197.
- Videla LA, Rodrigo R, Araya J, Poniachik J. (2004). Oxidative stress and depletion of hepatic long-chain polyunsaturated fatty acids may contribute to nonalcoholic fatty liver disease. Free Radic Biol Med, 37, 1499–1507.
- Warholm M, Guthenberg C, von Bahr C, Mannervik B. (1985). Glutathione transferases from human liver. Meth Enzymol, 113, 499–504.
- Wolterbeek HT, van der Meer AJ. (2005). Optimization, application, and interpretation of lactate dehydrogenase measurements in microwell determination of cell number and toxicity. Assay Drug Dev Technol, 3, 675–682.
- Yokozawa T, Kim HY, Kim HJ, Okubo T, Chu DC, Juneja LR. (2007). Amla (Emblica officinalis Gaertn.) prevents dyslipidaemia and oxidative stress in the ageing process. Br J Nutr, 97, 1187–1195.