Abstract
Context: Spices have been used as food adjuncts and in folklore for ages. Inhibition of key enzymes (α-amylase and α-glucosidase) involved in the digestion of starch and protection against free radicals and lipid peroxidation in pancreas could be part of the therapeutic approach towards the management of hyperglycemia and dietary phenolics have shown promising potentials.
Objective: This study investigated and compared the inhibitory properties of aqueous extracts of some tropical spices: Xylopia aethiopica [Dun.] A. Rich (Annonaceae), Monodora myristica (Gaertn.) Dunal (Annonaceae), Syzygium aromaticum [L.] Merr. et Perry (Myrtaceae), Piper guineense Schumach. et Thonn (Piperaceae), Aframomum danielli K. Schum (Zingiberaceae) and Aframomum melegueta (Rosc.) K. Schum (Zingiberaceae) against α-amylase, α-glucosidase, 1,1-diphenyl-2-picrylhydrazyl (DPPH) radicals and sodium nitroprusside (SNP)-induced lipid peroxidation in rat pancreas - in vitro using different spectrophotometric method.
Materials and methods: Aqueous extract of the spices was prepared and the ability of the spice extracts to inhibit α-amylase, α-glucosidase, DPPH radicals and SNP-induced lipid peroxidation in rat pancreas - in vitro was investigated using various spectrophotometric methods.
Result: All the spice extracts inhibited α-amylase (IC50 = 2.81–4.83 mg/mL), α-glucosidase (IC50 = 2.02–3.52 mg/mL), DPPH radicals (EC50 = 15.47–17.38 mg/mL) and SNP-induced lipid peroxidation (14.17–94.38%), with the highest α-amylase & α-glucosidase inhibitory actions and DPPH radical scavenging ability exhibited by X. aethiopica, A. danielli and S. aromaticum, respectively. Also, the spices possess high total phenol (0.88–1.3 mg/mL) and flavonoid (0.24–0.52 mg/mL) contents with A. melegueta having the highest total phenolic and flavonoid contents.
Discussion and conclusion: The inhibitory effects of the spice extracts on α-amylase, α-glucosidase, DPPH radicals and SNP-induced lipid peroxidation in pancreas (in vitro) could be attributed to the presence of biologically active phytochemicals such as phenolics and some non-phenolic constituents of the spices. Furthermore, these spices may exert their anti-diabetic properties through the mechanism of enzyme inhibition, free radicals scavenging ability and prevention of lipid peroxidation.
Introduction
Type 2 diabetes is regarded as the second most common noncommunicable disorder, after hypertension, in terms of public health significance (WHO, Citation1999). The high burden of diabetes mellitus (DM) in Nigeria is largely attributable to cardiovascular diseases which account for 15% of all DM deaths (CitationOgbera, 2007; CitationOgbera et al., 2007). Type 2 diabetes is a metabolic disorder resulting from the body’s inability to produce enough or properly utilize insulin. Type 2 diabetes patients have hyperglycemia but are ketosis resistant (WHO, Citation1999). Previous studies indicate that hyperglycemia-induced vascular dysfunction may be triggered by reactive oxygen species (ROS) produced in the mitochondrial electron transport chain (CitationKawamura et al., 1994; CitationBrownlee, 2005). ROS such as the superoxide anion radical (O2) hydrogen peroxide (H2O2) and hydroxyl radicals (OH) are physiological metabolites formed as a result of respiration in aerobic organisms. Uncontrolled generated ROS are very unstable, and react rapidly with other substances including DNA, membrane lipids and proteins. In humans, this is believed to be involved with DM (CitationNiedowicz & Daleke, 2005).
Starch is the major source of carbohydrates in most diets and plays a crucial role in energy supply. The dietary carbohydrates are first broken down to monosaccharides by some gastrointestinal enzymes, since only monosaccharides can be absorbed from intestinal lumen (CitationAbesundara et al., 2004; CitationKwon et al., 2006). α-Glucosidase and α-amylase are the key enzymes involved in the digestion of carbohydrates (CitationAli et al., 2006). α-Amylase degrades complex dietary carbohydrates to oligosaccharides and disaccharides that are ultimately converted into monosaccharides by α-glucosidase. Liberated glucose is then absorbed by the gut and results in postprandial hyperglycemia (CitationKim et al., 2000; CitationShim et al., 2003). The inhibition of enzymes involved in the digestion of carbohydrates can significantly decrease the postprandial increase of blood glucose after a mixed carbohydrate diet by delaying the process of carbohydrate hydrolysis and absorption (CitationKwon et al., 2006; CitationOboh et al., 2010a). The control of postprandial hyperglycemia is an important strategy in the management of DM, especially type II diabetes, and reducing chronic complications associated with the disease (CitationKim et al., 2000; CitationAli et al., 2006). This is done by retarding the absorption of glucose through the inhibition of the carbohydrate-hydrolysing enzymes α-glucosidase and α-amylase in the digestive tract (CitationKim et al., 2000; CitationShim et al., 2003; CitationOboh et al., 2010a). Inhibitors of these enzymes could cause delay in carbohydrate digestion, prolong overall carbohydrate digestion time, reduce the rate of glucose absorption and consequently blunting the postprandial plasma glucose rise (CitationBhadari et al., 2008). Several drugs such as acarbose, miglitol, voglibose, nojirimycin and 1-deoxynojirimycin had been developed and are currently in use. These drugs could act by either blocking or inhibiting these enzymes, however, they come with financial constraints and their attendant side effects, hence, alternative treatments need to be evaluated. Therefore, effective, nontoxic and cheap natural dietary inhibitors of α-amylase and α-glucosidase with little or no side effects could be potentially promising and highly desirable. Moreover, there is strong evidence that dietary factors could be involved in the regulation and prevention of type 2 diabetes (CitationKwon et al., 2007).
In recent times, research on medicinal plant and plant foods for the management of diabetes has attracted the interest of scientists (CitationMcCue et al., 2005; CitationAli et al., 2006) and quite a number of plants are known to exert their antihyperglycemic activity via inhibition of carbohydrate hydrolyzing enzymes (CitationMatsumoto et al., 1993; CitationMatsui et al., 2001; CitationJohnston et al., 2003; CitationMcDougall et al., 2005; CitationCheplick et al., 2007; CitationOboh et al., 2010a; CitationPinto et al., 2010; CitationRanilla et al., 2010). Many of the health-promoting characteristics of plant food are partly linked to the presence of an array of important phenolic phytochemicals including phenolic acids and flavonoids (CitationChu et al., 2002; CitationSun et al., 2002; CitationCheplick et al., 2007; CitationOboh & Rocha, 2007; CitationOboh et al., 2008, Citation2010a,Citationb; CitationWang et al., 2010). Phenolic compounds or phenolic phytochemicals are secondary metabolites of plants and are present in diets (CitationBravo, 1998). They exhibit strong antioxidant, free radical scavenging activity, and play crucial role in health promotion and disease prevention (CitationScalbert et al., 2005; CitationOboh & Rocha, 2007). Spices are esoteric food adjuncts that have been used as flavoring agents and as preservatives for thousands of years. Spices have also been recognized to possess medicinal properties and their use in traditional systems of medicine has been on record for a long time. This study seeks to evaluate the inhibitory effects of the water extractable phytochemicals from some tropical spices [Monodora myristica (Africa nutmeg), Xylopia aethiopica (Ethiopian pepper), Syzygium aromaticum (Cloves), Piper guineense (Black pepper), Aframomum danielli (Bastered melegueta) and Afromomum melegueta (Alligator pepper or grains of paradise)] on some key enzymes (α-amylase and α-glucosidase) linked with type 2 diabetes as well as their inhibitory effect on sodium nitroprusside (SNP)-induced lipid peroxidation in rat pancreas with the aim of investigating their potential as dietary intervention in the management or control of postprandial hyperglycemia associated with type 2 diabetes.
Materials and methods
Materials
Fresh samples of some tropical spices (M. myristica, X. aethiopica, S. aromaticum, P. guineense, A. danielli and A. melegueta) were purchased from Akure main market in Ondo State, Nigeria. Authentication of the samples was carried out by Dr. A. E Ayodele, a plant taxonomist in the Department of Botany and Microbiology, University of Ibadan, Oyo State, Nigeria. The different parts of the spices used for the analyses are described in . All the chemicals used were of analytical grade, while the water was glass distilled.
Table 1. Description of some commonly consumed Nigerian species.
Handling and use of animal
Approval was obtained from the relevant University ethics committee responsible for the use of laboratory animals. The handling and the use of the animals were in accordance with NIH Guide for the care and use of laboratory animals. In the experiment, Wistar strain albino rats weighing 200–230 g were purchased from the breeding colony of Department of Veterinary Medicine, University of Ibadan, Nigeria. Rats were maintained at 25°C, on a 12 h light/12 h dark cycle, with free access to food and water. They were acclimatized under these conditions for two weeks before the commencement of the experiment.
Aqueous extract preparation
The inedible portions of the seeds, fruits, flower and leaves were removed from the edible portions; the edible portions were subsequently washed in distilled water. The fruits, flower and leaves were chopped into small pieces by table knife, air dried and milled. Each milled sample was soaked in 100 mL distilled water for about 24 h. The mixture was filtered and later centrifuged at 400g for 10 min to obtain a clear supernatant which was then used for subsequent analysis (CitationOboh et al., 2007).
α-Amylase inhibition assay
The aqueous extracts dilution (500 µL) of spices and 500 µL of 0.02 mol/L sodium phosphate buffer (pH 6.9 with 0.006 mol·L−1 NaCl) containing hog pancreatic α-amylase (EC 3.2.1.1) (0.5 mg/mL) were incubated at 25°C for 10 min. Then, 500 µL of 1% starch solution in 0.02 mol·L−1 sodium phosphate buffer (pH 6.9 with 0.006 mol/L NaCl) was added to the reaction mixture. Thereafter, the reaction mixture was incubated at 25°C for 10 min and stopped with 1.0 mL of dinitrosalicylic acid color reagent. The mixture was then incubated in a boiling water bath for 5 min, and cooled to room temperature. The reaction mixture was then diluted by adding 10 mL of distilled water, and absorbance measured at 540 nm in the UV–Visible spectrophotometer (Model 6305; Jenway, Barloworld Scientific, Dunmow, United Kingdom). The α-amylase inhibitory activity was expressed as percentage inhibition (CitationBernfield, 1951).
Where,
Absref = Absorbance of reference
Abssam = Absorbance of sample
α-Glucosidase inhibition assay
Appropriate dilution of the aqueous extracts (50 µL) of spices and 100 µL of α-glucosidase solution (1.0 U/mL) in 0.1 mol/L phosphate buffer (pH 6.9) were incubated at 25°C for 10 min. Thereafter, 50 µL of 5 mmol/L p-nitrophenyl-α-d-glucopyranoside solutions in 0.1 mol/L phosphate buffer (pH 6.9) was added. The reaction mixture was then incubated at 25°C for 5 min, and then absorbance was measured at 405 nm in the UV–Visible spectrophotometer (Model 6305; Jenway, Barloworld Scientific, Dunmow, United Kingdom). The α-glucosidase inhibitory activity was expressed as percentage inhibition (CitationApostolidis et al., 2007).
Where,
Absref = Absorbance of reference
Abssam = Absorbance of sample
Lipid peroxidation assay
Preparation of tissue homogenates
The rats were decapitated under mild diethyl ether anaesthesia and the pancreas was rapidly excised, placed on ice and weighed. This tissue was subsequently homogenized in cold saline (1/10, w/v) with about 10 up and down strokes at approximately 1200 rpm in a Teflon glass homogenizer. The homogenate was centrifuged for 10 min at 3000× g to yield a pellet that was discarded, and a low-speed supernatant (S1) containing mainly water, proteins and lipids (cholesterol, galactolipid, individual phospholipids, gangliosides) was kept for lipid peroxidation assay (Belle et al., 2004).
Lipid peroxidation and thiobarbituric acid reactions
The lipid peroxidation assay was carried out by the modified method of CitationOhkawa et al. (1979). Briefly, 100 µL of S1 fraction was mixed with a reaction mixture containing 30 µL of 0.1 M Tris-HCl buffer (pH 7.4), extract (0–100 µL) and 30 µL of the pro-oxidant (7 µM sodium nitroprusside). The volume was made up to 300 µL by water before incubation at 37°C for 2 h. The color reaction was developed by adding 300 µL of 8.1% sodium duodecyl sulphate to the reaction mixture containing S1, followed by the addition of 600 µL of acetic acid/HCl (pH 3.4) and 600 µL of 0.8% thiobarbituric acid. This mixture was incubated at 100°C for 1 h. The absorbance of thiobarbituric acid reactive species produced were measured at 532 nm in UV–Visible spectrophotometer (Model 6305; Jenway, Barloworld Scientific, Dunmow, United Kingdom). Malondialdehyde (MDA) produced was expressed as % Control.
Phytochemical screening
The methods described by CitationTrease and Evans (1983) were used for phytochemical screening of spice extracts for the presence of bioactive compound.
Determination of total phenol content
The total phenol content was determined on the extracts using the method reported by CitationSingleton et al. (1999). Appropriate dilutions of the extracts were oxidized with 2.5 mL of 10% Folin–Ciocalteau’s reagent (v/v) and neutralized by 2.0 mL of 7.5% sodium carbonate. The reaction mixture was incubated for 40 min at 45°C and the absorbance was measured at 765 nm in the UV–Visible spectrophotometer (Model 6305, Barloworld Scientific, Dunmow, Essex, United Kingdom). The total phenol content was subsequently calculated using gallic acid as standard.
Determination of total flavonoid content
The total flavonoid content of the spice extracts was determined using a slightly modified method reported by CitationMeda et al. (2005). Briefly, 0.5 mL of appropriately diluted sample was mixed with 0.5 mL methanol, 50 µL of 10% AlCl3, 50 µL of 1 mol/L potassium acetate and 1.4 mL water, and allowed to incubate at room temperature for 30 min. Thereafter, the absorbance of the reaction mixture was subsequently measured at 415 nm in the Jenway UV–Visible spectrophotometer. The total flavonoid was calculated using quercetin as standard.
1,1-Diphenyl-2-picrylhydrazyl (DPPH) free radical scavenging ability
The free radical scavenging ability of the spice extracts against DPPH free radical was evaluated as described by CitationGyamfi et al. (1999). Briefly, an appropriate dilution of the spice extracts (1 mL) was mixed with 1 mL of 0.4 mmol/L methanol solution containing DPPH radicals. The mixture was left in the dark for 30 min and the absorbance was measured at 516 nm in the UV–Visible spectrophotometer (Model 6305; Jenway, Barloworld Scientific, Dunmow, United Kingdom). The DPPH free radical scavenging ability was subsequently calculated with respect to the reference, which contained all the reagents without the test sample.
Where,
Absref = Absorbance of reference
Abssam = Absorbance of sample
Data analysis
The result of the replicates were expressed as mean ± standard deviation (CitationZar, 1984). A one-way analysis of variance (ANOVA) and the least significance difference were carried out. Significance was accepted at p ≤ 0.05.
Results and discussion
The control of postprandial hyperglycemia is recognized to be an effective therapeutic approach in the management of type 2 diabetes and in reducing chronic vascular complications (CitationOrtiz-Andrade et al., 2007). Hence, inhibition of enzymes involved in the digestion of polysaccharides by dietary phytochemicals has shown promising potentials (CitationShim et al., 2003; CitationPinto et al., 2009; CitationOboh et al., 2010a).
α-Amylase and α-glucosidase are key enzymes involved in starch breakdown and intestinal absorption, respectively. In humans, the diabetogenic process may be caused by immune destruction of the β cells in the Islets of Langerhans in the pancreas and this is apparently mediated by white blood cell production of ROS (CitationOberley, 1988). Diabetes can be induced in animals by the drugs alloxan and streptozotocin; the mechanism of action of these two drugs is different, but both result in the production of ROS. Scavengers of oxygen radicals are effective in preventing diabetes in these animal models. Not only are oxygen radicals involved in the cause of diabetes, they also appear to play a role in some of the complications seen in long-term treatment of diabetes (CitationOberley, 1988). It is believed that inhibition of the enzymes involved in the digestion and uptake of carbohydrates can significantly decrease the postprandial increase of blood glucose level after a mixed carbohydrate diet and therefore can be an important strategy in the management of hyperglycemia linked to type 2 diabetes (CitationShim et al., 2003; CitationOrtiz-Andrade et al., 2007; CitationPinto et al., 2009). The ability of aqueous extract of some commonly consumed spices in Nigeria to inhibit α-amylase activity was investigated () and the result revealed that all the extracts inhibited α-amylase in a concentration-dependent manner (0–3 mg/mL); with the 50% inhibition concentration by (IC50) values shown in . However, X. aethiopica extract had the highest inhibitory activity on α-amylase (IC50 = 2.81 mg/mL), while A. melegueta extract showed the least inhibition against α-amylase (IC50 = 4.83 mg/mL). The trend in the determined α-amylase inhibitory activity followed similar trend with some earlier reports where plant phytochemicals in six Allium species inhibited α-amylase activity (CitationNickavar & Yousefian, 2009). The result agrees with our recent work, where red and white ginger inhibited α-amylase activity in vitro (CitationOboh et al., 2010a).
Figure 1. α-Amylase inhibitory activities of aqueous extracts of some tropical spices. Values represent mean ± standard deviation, n = 3. AD, A. danielli; AM, A. melegueta; MM, M. myristica; PG, P. guineense; SA, S. aromaticum; XA, X. aethiopica.
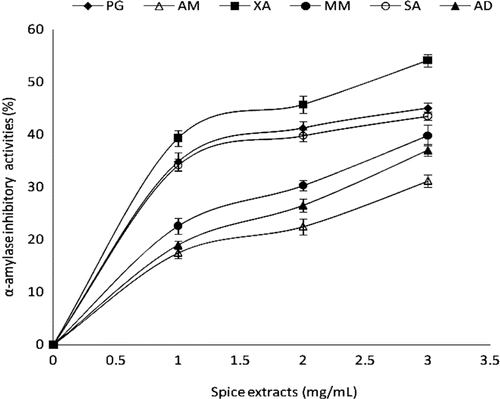
Subsequently, α-glucosidase inhibitory activities of the spice extracts was assessed and shown in . All the spice extracts inhibited α-glucosidase activities in a concentration-dependent manner (0–3 mg/mL). However, the highest inhibitory activity on α-glucosidase was shown by A. danielli (IC50 = 2.02 mg/mL), while M. myristica had the least α-glucosidase inhibitory activity (IC50 = 3.52 mg/mL). Also, the result of α-glucosidase inhibitory activities of spice extracts follow similar trend with recent reports on the inhibitory potentials of commonly used medicinal plants, herbs and spices in Latin America against key enzymes relevant to hyperglycemia (CitationRanilla et al., 2010) and that of common constituents from some traditional Chinese medicine used for DM (CitationYe et al., 2010).
Table 2. EC50 /IC50 values of DPPH radical scavenging ability, α-amylase and α-glucosidase inhibitory activity of aqueous extract of some tropical spices
Figure 2. α-Glucosidase inhibitory activities of aqueous extracts of some tropical spices. Values represent mean ± standard deviation, n = 3. AD, A. danielli; AM, A. melegueta; MM, M. myristica; PG, P. guineense; SA, S. aromaticum; XA, X. aethiopica.
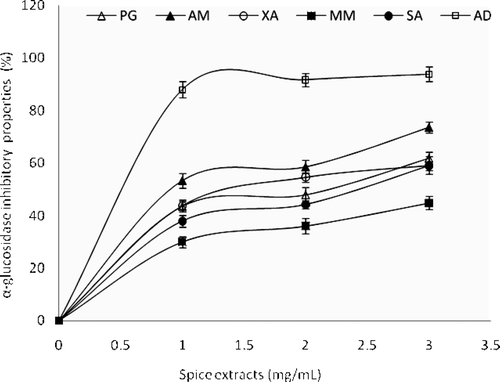
Furthermore, it is worth noting that all the spice extracts showed higher α-glucosidase inhibitory activities (IC50 = 2.02–3.52 mg/mL) than their respective α-amylase inhibitory activities (IC50 = 2.81-4.83 mg/mL). This agrees with previous reports that plant phytochemicals are mild inhibitors of α-amylase and strong inhibitors of α-glucosidase activities (CitationMcDougall et al., 2005; CitationKwon et al., 2007; CitationPinto et al., 2009; CitationOboh et al., 2010a). This action may pose an advantage over synthetic drugs presently in use for the management of postprandial blood glucose, such as acarbose, which strongly inhibit α-amylase. There are indications that excessive pancreatic amylase inhibition could result into abnormal bacterial fermentation of undigested saccharides in the colon (CitationHorii et al., 1986; CitationBischoff, 1994). Hence, stronger inhibition of α-glucosidase activity and mild inhibition of α-amylase activity of the spice extracts could minimize the major setback of currently used α-glucosidase and α-amylase inhibitory drugs with side effects such as abdominal distention, flatulence, meteorism and possibly diarrhea (CitationBischoff, 1994). Therefore, this study supports the assertion that natural inhibitors from some plant foods have lower inhibitory effect against α-amylase activity and stronger α-glucosidase inhibitory activity, and could be effectively used in the management of postprandial hyperglycemia with minimal side effects (CitationKwon et al., 2007; CitationOboh et al., 2010a; CitationPinto et al., 2010).
Increased oxidative stress is widely accepted participant in the development and progression of diabetes (CitationMaritim et al., 2003). Although, the mechanisms by which increased oxidative stress is involved in the diabetic complications are partly known, including glucose oxidation, non-enzymatic glycation of proteins, and the subsequent oxidative degradation of glycated proteins (CitationMaritim et al., 2003). Abnormally high levels of free radicals and the simultaneous decline of antioxidant defense mechanisms could lead to damage of cellular organelles and enzymes, increased lipid peroxidation and development of insulin resistance. Insulin resistance is a major contributor to progression of type 2 DM and its complications (CitationWeyer et al., 2001). This could have originated from certain oxidative stress related defect(s) in oxidative phosphorylation machinery and mitochondrial β-oxidation leading to excess accumulation of intracellular triglyceride in muscle and liver and subsequently insulin resistance (CitationRosca et al., 2005). The pancreatic β cells are no longer able to compensate for insulin resistance because of increased insulin production and impaired glucose tolerance, characterized by excessive postprandial hyperglycemia (CitationGerich, 2003). This assertion is in line with the work carried out by CitationEvans et al. (2003) that pancreatic β-cell dysfunction could result from prolonged exposure to high glucose, elevated free fatty acid levels, or a combination of both. The pancreatic β cells could be susceptible to ROS because they are low in antioxidant enzymes such as catalase, glutathione peroxidase, and superoxide dismutase. Therefore, the ability of the spice extracts to inhibit SNP-induced lipid peroxidation in isolated rat pancreas homogenates was carried out and presented in . SNP is a potent and rapidly acting intravenous hypotensive agent. It is a peripheral vasodilator that acts directly on the smooth muscle fibers of blood vessels (CitationKaisserlian et al., 2005). This direct action is thought to be due to the release of the nitroso group in form of nitric oxide (NO). NO has also been implicated as an important signaling molecule in the contraction-mediated glucose uptake pathway. Nevertheless, SNP may also cause cytotoxicity due to the release of cyanide, which produces a real complication (CitationVaron & Marik, 2000). Incubation of the rat pancreas in the presence of 7 µM SNP (induced) caused a significant increase (p < 0.05) in the MDA content of the pancreas (122.50%) as presented in . However, the introduction of the water extractable phytochemicals from the spices caused a significant decrease (p < 0.05) in the MDA content of sodium nitroprusside-stressed pancreas homogenates (14.17–94.38%) in a concentration-dependent manner (0.16–0.96 mg/mL) with Aframomum melegueta showing the highest inhibition of MDA production, while X. aethiopica had the least inhibition of MDA production in a concentration-dependent manner. The result of the inhibition of MDA production agrees with CitationOboh et al. (2010a), where red and white ginger extract inhibited some prooxidants induced lipid peroxidation in rat brain. The inhibition of SNP-induced lipid peroxidation in pancreas tissues by the spice extracts () could be attributed to the ability of the extractable phytochemicals to scavenge NO˙ radical produced by the SNP, thus protecting the pancreas against oxidative insults and possibly upregulating the antioxidant enzymes.
Figure 3. Inhibition of sodium nitroprusside (SNP) induced lipid peroxidation in rat pancreas by aqueous extracts of some commonly consumed spices. Values represent mean ± standard deviation, n = 3; Basal = lipid peroxidation without SNP as prooxidants and no spice extracts; Induced = Lipid peroxidation with SNP as prooxidants and no spice extracts. AD, A. danielli; AM, A. melegueta; MM, M. myristica; PG, P. guineense; SA, S. aromaticum; XA, X. aethiopica.
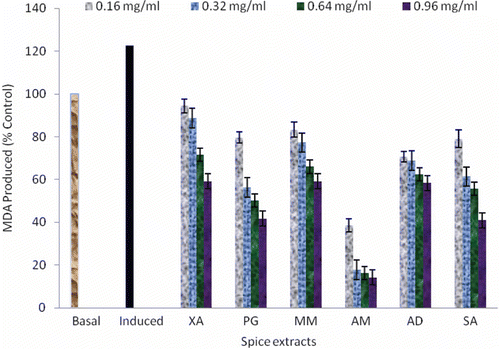
Free radical scavenging is one of the known mechanisms by which antioxidants inhibit lipid oxidation and DPPH is frequently used in its determination. The free radical DPPH possesses a characteristic absorption at 517 nm (purple in color), which decreases significantly on exposure to radical-scavengers (by providing hydrogen atoms or by electron donation). Therefore, the capacity of the spice extracts to scavenge the ‘‘stable’’ free radical DPPH radical was monitored and shown in . The extract concentration causing 50% inhibition (EC50) values obtained from the dose-response curve () revealed that S. aromaticum had the highest DPPH scavenging effects (15.47 mg/mL), while the least effect was exhibited by A. melegueta (17.38 mg/mL). The trend in the results of the ability of the individual spice extracts to inhibit the key enzymes linked to type 2 diabetes did not follow a similar trend with that of the spices in scavenging the DPPH radicals.
Figure 4. DPPH free radical scavenging ability of aqueous extract of some tropical spices. Values represent mean ± standard deviation, n = 3. AD, A. danielli; AM, A. melegueta; MM, M. myristica; PG, P. guineense; SA, S. aromaticum; XA, X. aethiopica.
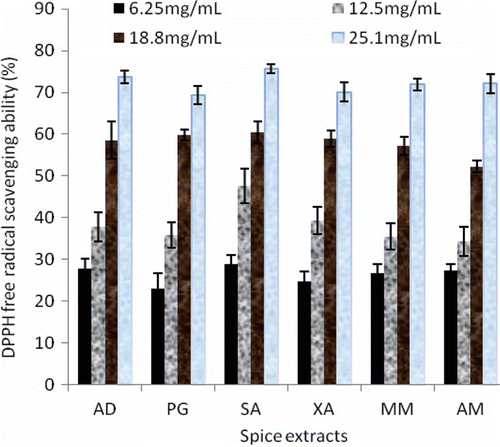
The presence of phytochemicals and phenolic compounds has been shown to contribute immensely to the protective potential against degenerative diseases, therapeutic effects essential to preventing diseases and nutritional quality of food and food products (CitationChu et al., 2002; CitationOboh et al., 2010b). Thus, the preliminary phytochemical screening tests may be useful in the detection of the bioactive principles and could subsequently lead to the development of functional foods and nutraceuticals. In order to ascertain the phytochemicals responsible for the biological activities of these spices in vitro, the spices were screened for the presence of various phytochemicals. The result of the phytochemical screening as shown in revealed the presence of flavonoid and cardiac glycoside in all the spices tested, tannin in X. aethiopica and S. aromaticum, phlobatanin, and anthraquinone in S. aromaticum and saponin in all spices under investigation (except in M. myristica). Previous studies have shown that these spices are rich in phenols and flavonoids, hence their disease preventing and health promoting abilities have been attributed to the presence of these phytochemicals (CitationFasoyiro et al., 2006; CitationOlonisakin et al., 2006; CitationWojdyło et al., 2007; CitationUwakwe & Nwaoguikpe, 2008; CitationEzekwesili et al., 2010; CitationDoherty et al., 2010). Dietary polyphenols are the most abundant antioxidants in human diets and represent a group of secondary metabolites which widely occur in fruits, vegetables, spices, wine, tea, extra virgin olive oil, chocolate and other cocoa products. They exhibit many biologically significant functions, such as protection against oxidative stress, and degenerative diseases (CitationBravo, 1998; CitationScalbert et al., 2005). Phenolic acids account for about one third of the total intake and flavonoids account for the remaining two thirds. Flavonoids are the most abundant polyphenols in human diets and flavanols (catechins plus proanthocyanidins), anthocyanins and their oxidation products have been shown to be the most abundant flavonoids in the diet (CitationScalbert et al., 2005). Phenolic compounds can remove free radicals, chelate metal catalysts, activate antioxidant enzymes, and reduce ions and radicals (CitationAmic et al., 2003). The result of the total phenol content of spices is presented in . A. melegueta had the highest total phenol content (2.28 mg/g), followed by A. danielli (1.65 mg/g) and M. myristica (0.60 mg/g) having the least total phenol content. These values were generally higher than the phenolic content reported for red and white ginger (CitationOboh et al., 2010a) except M. myristica that was lower. Also, the values were generally higher than that reported by CitationOboh et al. 2007 for hot pepper. In the same vein, the total flavonoid contents of the spices are also shown in with A. melegueta (0.55 mg/g) having the highest flavonoid content, followed P. guineense (0.41 mg/g), A. danielli (0.29 mg/g), S. aromaticum (0.26 mg/g), X. aethiopica (0.24 mg/g) and M. myristica (0.21 mg/g). The result of the phytochemical screening alongside the total phenol and total flavonoids contents showed clearly that all the spices tested are rich sources of polyphenols and flavonoids; which of late have aroused great interest owing to their antioxidant activities and enzyme inhibitory properties.
Table 3. Phytochemical constituents of some tropical spices.
Table 4. Phenolic distribution of some tropical spices (mg/g).
Conclusions
The results of the enzyme (α-amylase and α-glucosidase) inhibitory assays and that of the antioxidant assays did not follow a similar trend with the phenolic contents of the spices. The high inhibitory effect of aqueous extracts of the spices on α-amylase and α-glucosidase activities could be attributed to the presence of some non-phenolic phytochemicals that are potent enzyme inhibitors, exhibiting an additive or synergistic effect with the phenolics present in the spices. Furthermore, these spices may exert their anti-diabetic properties through the mechanism of enzyme inhibition, free radicals scavenging ability and prevention of lipid peroxidation.
Declaration of interest
The authors report no conflicts of interest.
References
- Abesundara KJ, Matsui T, Matsumoto K. (2004). α-Glucosidase inhibitory activity of some Sri Lanka plant extracts, one of which, Cassia auriculata, exerts a strong antihyperglycemic effect in rats comparable to the therapeutic drug acarbose. J Agric Food Chem, 52, 2541–2545.
- Ali H, Houghton PJ, Soumyanath A. (2006). α-Amylase inhibitory activity of some Malaysian plants used to treat diabetes; with particular reference to Phyllanthus amarus. J Ethnopharmacol, 107, 449–455.
- Amic D, Davidovic-Amic D, Beslo D, Trinajstic N. (2003). Structure-radical scavenging activity relationship of flavonoids. Croat Chem Acta, 76, 55–61.
- Apostolidis E, Kwon YI, Shetty K. (2007). Inhibitory potential of herb, fruit, and fungal-enriched cheese against key enzymes linked to type 2 diabetes and hypertension. Innov Food Sci Emerg Technol, 4, 46–54.
- Bellé NA, Dalmolin GD, Fonini G, Rubin MA, Rocha JB. (2004). Polyamines reduces lipid peroxidation induced by different pro-oxidant agents. Brain Res, 1008, 245–251.
- Bernfield P. (1951). Enzymes of starch degradation and synthesis. Adv Enzymol, 12, 379–428.
- Bhadari MR, Jong-Anarakkan N, Hong G, Kawabata J. (2008). α-Glucosidase and α-amylase inhibitory activities of Nepalese medicinal herb Pakhanbhed (Bergenia ciliata Haw). Food Chem, 106, 247–252.
- Bischoff H. (1994). Pharmacology of alpha-glucosidase inhibition. Eur J Clin Invest, 24, 3–10.
- Bravo L. (1998). Polyphenols: Chemistry, dietary sources, metabolism, and nutritional significance. Nutr Rev, 56, 317–333.
- Brownlee M. (2005). The pathobiology of diabetic complications: A unifying mechanism. Diabetes, 54, 1615–1625.
- Brownlee M, Cerami A. (1981). The biochemistry of the complications of diabetes mellitus. Annu Rev Biochem, 50, 385–432.
- Cheplick S, Kwon Y, Bhowmik P, Shetty K. (2007). Clonal variation in raspberry fruit phenolics and relevance for diabetes and hypertension management. J Food Biochem, 31, 656–679.
- Chu YF, Sun J, Wu X, Liu RH. (2002). Antioxidant and antiproliferative activities of common vegetables. J Agric Food Chem, 50, 6910–6916.
- Doherty VF, Olaniran OO, Kanife UC. (2010). Antimicrobial activities of Aframomum melegueta (Alligator pepper). Int J Biol, 2, 126–131.
- Evans JL, Goldfine ID, Maddux BA, Grodsky GM. (2003). Are oxidative stress-activated signaling pathways mediators of insulin resistance and β-cell dysfunction? Diabetes, 52, 1–8.
- Ezekwesili CN, Nwodo OFC, Eneh FU, Ogbunugafor HA. (2010). Investigation of the chemical composition and biological activity of Xylopia aethiopica Dunal (Annonacae). Afr J Biotechnol, 9, 7352–7356.
- Fasoyiro SB, Adegoke GO, Idowu OO. (2006). Characterization and partial purification of antioxidant component of ethereal fractions of Aframomum danielli. World J Chem, 1, 1–5.
- Gerich JE. (2003). Clinical significance, pathogenesis, and management of postprandial hyperglycemia. Arch Intern Med, 163, 1306–1316.
- Gyamfi MA, Yonamine M, Aniya Y. (1999). Free-radical scavenging action of medicinal herbs from Ghana: Thonningia sanguinea on experimentally-induced liver injuries. Gen Pharmacol, 32, 661–667.
- Horii S, Fukase H, Matsuo T, Kameda Y, Asano N, Matsui K. (1986). Synthesis and α-d-glucosidase inhibitory activity of N-substituted valiolamine derivatives as potential oral antidiabetic agents. J Med Chem, 29, 1038–1046.
- Johnston KL, Clifford MN, Morgan LM. (2003). Coffee acutely modifies gastrointestinal hormone secretion and glucose tolerance in humans: Glycemic effects of chlorogenic acid and caffeine. Am J Clin Nutr, 78, 728–733.
- Kaisserlian CE, Razzouq N, Astier A, Paul M. (2005). Sodium nitroprusside stability at 1 μg/mL in aqueous solutions. Eur J Hosp Pharm Sci, 11, 88–90.
- Kawamura M, Heinecke JW, Chait A. (1994). Pathophysiological concentrations of glucose promote oxidative modification of low density lipoprotein by a superoxide-dependent pathway. J Clin Invest, 94, 771–778.
- Kim JS, Kwon CS, Son KH. (2000). Inhibition of α-glucosidase and amylase by luteolin, a flavonoid. Biosci Biotechnol Biochem, 64, 2458–2461.
- Kwon YI, Jang HD, Shetty K. (2006). Evaluation of Rhodiola crenulata and Rhodiola rosea for management of type II diabetes and hypertension. Asia Pac J Clin Nutr, 15, 425–432.
- Kwon YI, Apostolidis E, Kim YC, Shetty K. (2007). Health benefits of traditional corn, beans, and pumpkin: In vitro studies for hyperglycemia and hypertension management. J Med Food, 10, 266–275.
- Maritim AC, Sanders RA, Watkins JB 3rd. (2003). Diabetes, oxidative stress, and antioxidants: A review. J Biochem Mol Toxicol, 17, 24–38.
- Matsui T, Ueda T, Oki T, Sugita K, Terahara N, Matsumoto K. (2001). α-glucosidase inhibitory action of natural acylated anthocyanins. 2. α-glucosidase inhibition by isolated acylated anthocyanins. J Agric Food Chem, 49, 1952–1956.
- Matsumoto N, Ishigaki F, Ishigaki A, Iwashina H, Hara Y. (1993). Reduction of blood glucose levels by tea catechin. Biosci Biotechnol Biochem, 57, 525–527.
- McCue P, Kwon YI, Shetty K. (2005). Anti-diabetic and anti-hypertensive potential of sprouted and solid-state bioprocessed soybean. Asia Pac J Clin Nutr, 14, 145–152.
- McDougall GJ, Shpiro F, Dobson P, Smith P, Blake A, Stewart D. (2005). Different polyphenolic components of soft fruits inhibit α-amylase and α-glucosidase. J Agric Food Chem, 53, 2760–2766.
- Meda A, Lamien CE, Romito M, Millogom J, Nacoulma OG. (2005). Determination of the total phenolic, flavonoid and proline contents in Burkina Faso honey, as well as their radical scavenging activity. Food Chem, 91, 571–577.
- Nickavar B, Yousefian N. (2009). Inhibitory effects of six Allium species on α-amylase enzyme activity. Iranian J Pharm Res, 8, 53–57.
- Niedowicz DM, Daleke DL. (2005). The role of oxidative stress in diabetic complications. Cell Biochem Biophys, 43, 289–330.
- Oberley LW. (1988). Free radicals and diabetes. Free Radic Biol Med, 5, 113–124.
- Oboh G, Puntel RL, Rocha JBT. (2007). Hot pepper (Capsicum annuum Tepin and Capsicum chinense Habanero) prevents Fe2+-induced lipid peroxidation in brain-in vitro. Food Chem, 102, 178–185.
- Oboh G, Rocha JBT. (2007). Antioxidant in foods: A New Challenge for Food Processors: Leading Edge Antioxidants Research. New York US: Nova Science Publishers Inc; 35–64.
- Oboh G, Raddatz H, Henle T. (2008). Antioxidant properties of polar and non-polar extracts of some tropical green leafy vegetables. J Sci Food Agric, 88, 2486–2492.
- Oboh G, Akinyemi AJ, Ademiluyi AO, Adefegha SA. (2010a). Inhibitory effects of aqueous extract of two varieties of ginger on some key enzymes linked to type-2 diabetes in vitro. J Food Nutr Res, 49, 14–20.
- Oboh G, Akomolafe TL, Adefegha SA, Adetuyi AO. (2010b). Antioxidant and modulatory effect of ethanolic extract of Madagascar Harungana (Harungana madagascariensis) bark on cyclophosphamide induced neurotoxicity in rats. J Food Drug Anal, 18, 171–179.
- Ogbera O. (2007). Burden of diabetic illness in an urban hospital in Nigeria. Trop Doct, 37, 153–154.
- Ogbera AO, Chinenye S, Onyekwere A, Fasanmade O. (2007). Prognostic indices of diabetes mortality. Ethn Dis, 17, 721–725.
- Ohkawa H, Ohishi N, Yagi K. (1979). Assay for lipid peroxides in animal tissues by thiobarbituric acid reaction. Anal Biochem, 95, 351–358.
- Olonisakin A, Oladimeji MO, Lajide L. (2006). Chemical composition and antibacterial activity of steam distilled oil of Ashanti Pepper (Piper guineense) fruits (berries). Electr J Environ Agric Food Chem, 5, 1531–1535.
- Ortiz-Andrade RR, García-Jiménez S, Castillo-España P, Ramírez-Avila G, Villalobos-Molina R, Estrada-Soto S. (2007). α-Glucosidase inhibitory activity of the methanolic extract from Tournefortia hartwegiana: An anti-hyperglycemic agent. J Ethnopharmacol, 109, 48–53.
- Pinto Mda S, Ranilla LG, Apostolidis E, Lajolo FM, Genovese MI, Shetty K. (2009). Evaluation of antihyperglycemia and antihypertension potential of native Peruvian fruits using in vitro models. J Med Food, 12, 278–291.
- Pinto MD, Kwon YI, Apostolidis E, Lajolo FM, Genovese MI, Shetty K. (2010). Evaluation of red currants (Ribes rubrum L), black currants (Ribes nigrum L.), red and green gooseberries (Ribes uva-crispa) for potential management of type 2 diabetes and hypertension using in vitro models. J Food Biochem, 34, 639–660.
- Ranilla LG, Kwon YI, Apostolidis E, Shetty K. (2010). Phenolic compounds, antioxidant activity and in vitro inhibitory potential against key enzymes relevant for hyperglycemia and hypertension of commonly used medicinal plants, herbs and spices in Latin America. Bioresour Technol, 101, 4676–4689.
- Rosca MG, Mustata TG, Kinter MT, Ozdemir AM, Kern TS, Szweda LI, Brownlee M, Monnier VM, Weiss MF. (2005). Glycation of mitochondrial proteins from diabetic rat kidney is associated with excess superoxide formation. Am J Physiol Renal Physiol, 289, 420–430.
- Scalbert A, Manach C, Morand C, Rémésy C, Jiménez L. (2005). Dietary polyphenols and the prevention of diseases. Crit Rev Food Sci Nutr, 45, 287–306.
- Shim YJ, Doo HK, Ahn SY, Kim YS, Seong JK, Park IS, Min BH. (2003). Inhibitory effect of aqueous extract from the gall of Rhus chinensis on α-glucosidase activity and postprandial blood glucose. J Ethnopharmacol, 85, 283–287.
- Singleton V L, Orthofer R, Lamuela-Raventos RM. (1999). Analysis of total phenols and other oxidation substrates and antioxidants by means of Folin–Ciocalteu reagent. Method in Enzymol, 299, 152–178.
- Sun J, Chu YF, Wu X, Liu RH. (2002). Antioxidant and antiproliferative activities of common fruits. J Agric Food Chem, 50, 7449–7454.
- Trease GE, Evans W C. (1983). Pharmacognosy. 12th edition. London, Britain: Bailliere, Tindal, pp 469–470.
- Uwakwe AA, Nwaoguikpe RN. (2008). In vitro antisickling effects of Xylopia aethiopica and Monodora myristica. J Med Plants Res, 2, 119–124.
- Varon J, Marik PE. (2000). The diagnosis and management of hypertensive crises. Chest, 118, 214–27.
- Wang H, Du YJ, Song HC. (2010). α-glucosidase and α-amylase inhibitory activities of guava leaves. Food Chem, 123, 6–13.
- Weyer C, Funahashi T, Tanaka S, Hotta K, Matsuzawa Y, Pratley RE, Tataranni PA. (2001). Hypoadiponectinemia in obesity and type 2 diabetes: Close association with insulin resistance and hyperinsulinemia. J Clin Endocrinol Metab, 86, 1930–1935.
- Wojdyło A, Oszmian’ski J, Czemerys R. (2007). Antioxidant activity and phenolic compounds in 32 selected herbs. Food Chem, 105, 940–949.
- World Health Organization Consultation. Definition, Diagnosis and Classification of Diabetes Mellitus and its Complications, Part 1: Diagnosis and Classification of Diabetes Mellitus. Report of a WHO Consultation. Geneva: World Health Organization; 1999.
- Ye X, Song C, Yuan P, Mao R. (2010). α-Glucosidase and α-amylase inhibitory activity of common constituents from traditional Chinese medicine used for diabetes mellitus. Chin J Nat Med, 8, 349–352.
- Zar JH. (1984). Biostatistical Analysis. USA: Prentice-Hall, Inc.; 620.