Abstract
Context: Erythrina velutina (EV) Willd (Fabaceae–Faboideae) is a medicinal tree that is commonly used in Brazil for the treatment of several central nervous system disorders.
Objective: The anticholinesterase activity of EV is described in this work.
Methods: Concentration-response curves (0–1.6 mg/mL) for EV leaf aqueous extract (AE) and alkaloid-rich extracts (AKEs) were performed in vitro. Cholinesterase inhibition was examined in mouse brains, as the cholinesterase source, and in pure acetylcholinesterase (AChE) or butyrylcholinesterase (BuChE). Mice were treated with AE or AKE (100, 200, and 400 mg/kg, p.o.) and their brains were used for the measurement of cholinesterase activity (CA) ex vivo.
Results: CA was inhibited by AE (IC50 = 0.57 [0.43–0.75] mg/mL) and AKE (IC50 = 0.52 [0.39–0.70] mg/mL) in brain homogenates in a concentration-dependent manner. The ex vivo experiments indicated that AE (400 mg/kg, p < 0.05, 32.2 ± 3.9% of inhibition) and AKE (all doses: p < 0.05–p < 0.001, 29.6 ± 3.2% as the maximum inhibition) significantly inhibited CA in the central nervous system after oral administration. AE and AKE inhibited AChE and BuChE activities in a concentration-dependent manner (AE: IC50AChE = 0.56 [0.38–0.81] mg/mL, IC50BuChE = 2.95 [1.51–5.76] mg/mL, AKE: IC50AChE = 0.87 [0.60–12.5] mg/mL, IC50BuChE = 2.67 [0.87–8.11] mg/mL).
Discussion and conclusions: These data indicated that AE and AKE crossed the blood-brain barrier to inhibit CA in the brain. AE and AKE also exhibited a dual inhibitory action on acetyl- and BuChE.
Introduction
Erythrina velutina (EV) Willd (Fabaceae–Faboideae) is an ornamental and medicinal tree that is commonly used against a number of central nervous system disorders, such as anxiety, insomnia, convulsions, and nervous cough. The most popular use of EV is the oral administration of the aqueous extract (AE) (infusion or decoction) or a tincture (ethanol/water extract) that is prepared from freshly harvested stem bark (CitationTeske & Trentini, 1995; CitationMatos, 1997). EV leaf extracts have been used in several pharmacological studies instead of stem bark, because the use of stem bark represents a risk for the species survival. These leaf extracts have anxiolytic, sedative, and analgesic effects in rodents (CitationDantas et al., 2004; CitationMarchioro et al., 2005) and have no acute toxicity in rats at doses up to 5 g/kg (CitationCraveiro et al., 2008).
The turnover regulation of acetylcholine in synaptic junctions plays an important role in a number of diseases, including Alzheimer’s disease (AD). AD is a progressive neurodegenerative disorder that is characterized by deteriorations in memory and other cognitive functions. The inhibition of brain cholinesterase, which increases extracellular acetylcholine levels, is the major therapeutic target for combating AD cognitive impairment (CitationBallard et al., 2011).
Two different enzymes, acetylcholinesterase (EC 3.1.1.7, AChE) and butyrylcholinesterase (EC 3.1.1.8, BuChE), hydrolyze acetylcholine in vertebrates. These enzymes differ significantly in their substrate specificity, enzyme kinetics, expression and activity in different regions of the brain. BuChE activity progressively increases and AChE activity declines as the severity of dementia advances in AD patients. Therefore, drugs that inhibit both enzymes may be preferred over selective inhibitors of AChE for the treatment of AD patients (CitationLane et al., 2006). Currently, AD has not been successfully managed despite reaching epidemic proportions, and more effective and safe treatments are required (CitationBallard et al., 2011).
The potential for plants to yield new therapeutic agents has stimulated extensive research towards the discovery of new cholinesterase inhibitors from plant extracts, phytochemicals and their derivatives. For example, Melissa officinalis L. (Lamiaceae), Ginkgo biloba L. (Ginkgoaceae) and Salvia officinalis L. (Lamiaceae) have anticholinesterase activity and alleviate AD cognitive deficits (CitationAkhondzadeh et al., 2003a,Citationb; CitationMukherjee et al 2007; CitationWeinmann et al., 2010).
Alkaloids, a type of plant metabolite, play a central role in AD treatment and drug development. Only five drugs have been licensed for the alleviation of AD cognitive symptoms. Two of these drugs, galantamine and rivastigmine, are alkaloids that exhibit anticholinesterase activity (Pulok et al., 2007; CitationBallard et al., 2011). The quinolizidine alkaloid, (−)-huperzine-A, which is isolated from Huperzia serrata (Thunb. ex Murray) Trevis (Huperziaceae) and Huperzia dalhousieana (Spring) Trevis (Huperziaceae), is under clinical evaluation for the treatment of AD cognitive symptoms (CitationWang et al., 2006). In addition, the natural indol alkaloid physostigmine is a cholinesterase inhibitor prototype that has been used for the development of synthetic cholinesterase inhibitors (Pulok et al., 2007).
Taking these into account, this study was conducted to explore the diverse flora of Brazil for new plants with anticholinesterase activity for the development of potential tools to combat AD cognitive symptoms. The anticholinesterase activity of an AE from EV leaves was investigated in mouse brains. The anticholinesterase activity of EV leaf alkaloid-rich extract (AKE) was also examined, because leaves of Erythrina species have high alkaloid content, and alkaloids are known to play a central role in the development of anticholinesterase medicines. Ex vivo experiments were used to investigate whether EV extracts are active in the central nervous system after oral administration. The mice were treated with AE or AKE, and brain cholinesterase activity (CA) was measured. In addition, the inhibition of AChE and BuChE enzymes by EV leaf extracts was characterized. The potential use of EV leaf extracts as a centrally active cholinesterase inhibitor of both AChE and BuChE enzymes is proposed.
Methods
Plant material
Fresh leaves of EV were collected in July 2005 in São Cristovão City (10°56′S, 37°05′W), Sergipe State, Brazil. The species was identified by biologist Adauto Souza Ribeiro, and a voucher specimen (number ASE01137) was deposited at the Herbarium of the Federal University of Sergipe, Brazil.
Leaf aqueous extract
Dried and powdered leaves (400 g) were infused with distilled water (1:5 w/v, 100°C), filtered and lyophilized in a VirTis bench-top freeze dryer to yield a dark brown powder (AE, 8.1%).
Alkaloid-rich extract
AE (15 g) was diluted in water (1:5 w/v) and acidified with 10% acetic acid. The suspension was filtered, and insoluble material was discarded. The filtrate was partitioned with CHCl3 to yield the acidic fraction after evaporation to dryness. The acidic water phase was rendered alkaline (pH 9) with NH4OH and extracted with CHCl3. The organic layers were combined, washed with water and concentrated under vacuum to recover the total alkaloid fraction of the EV leaves (AKE, 1.8%).
Test animals
Healthy male Swiss mice (25–30 g) were maintained in plastic boxes (five animals per cage). The animals were maintained in standard environmental conditions (12/12 h light/dark cycle) with free access to a pellet diet (Purina chow) and tap water. The animals were handled humanely in accordance with the guidelines of the European Convention for the Protection of Vertebrate Animals and Other Scientific Purposes ETS-123 (CitationEuropean Treaty Series, 2005). The Scientific and Bioethics Committee at the Federal University of Sergipe approved these studies (number 0067/2006).
Animal grouping and extract administration
A total of 40 animals were divided into 10 groups (A–J, n = 4) in a complete randomized design. The animals were deprived of food for 6 h prior to the treatments. Distilled water (vehicle, 0.1 mL/10 g) was administered orally to animals in group A (control) using a metal oropharyngeal cannula. Animals in groups B, C, and D were treated with 100, 200, and 400 mg/kg body weight of AE, respectively, following dilution in the vehicle. Groups E, F, and G received oral AKE diluted in water at doses of 100, 200, and 400 mg/kg body weight, respectively. Neostigmine bromide was used as a positive control and administered at doses of 0.1, 1.0, and 10.0 mg/kg (p.o.) body weight to animals in groups H, I, and J, respectively.
Cholinesterase activity
Enzyme activities were measured using the spectrophotometric method of CitationEllman et al. (1961) with slight modifications.
Inhibition of mouse brain cholinesterases (in vitro assays)
All animals from each group (n = 4) were decapitated. The brains were quickly removed, placed separately on an ice-cold plate and washed with iced buffer (0.5 M sodium phosphate, pH 7.4). The frontal cortex was removed immediately, homogenized in 1.0 mL of buffer, and centrifuged at 900g for 5 min. The resulting supernatant was used as the source of enzymes. All steps were performed at 4°C. AE and AKE were thoroughly diluted to 50 mg/mL in sodium phosphate buffer immediately prior to use. Neostigmine (10 nM–10 µM) or the extracts (0–1.6 mg/mL), the enzyme source (25 µL), 100 µL of Ellman’s reagent [10 mM; 5-50-dithio-bis(2-nitrobenzoic acid)], and 190 µL of propionylthiocholine iodide (75 mM) were combined and incubated at 25°C. Absorbance (412 nm) readings were obtained after 60 min of incubation at 25°C. The enzymatic reaction was stopped by the addition of 1.0 mL quinidine sulfate (0.5%). A blank reading was obtained for each reaction mixture without incubation. Each absorbance reading was performed in duplicate.
Inhibition of mouse brain cholinesterases (ex vivo experiments)
Brain homogenates (n = 4) were prepared from mice that were killed 4 h after the treatment with AE, AKE or neostigmine as explained above. The brain cortex of each animal was dissected and processed separately as described above. CA was measured in aliquots of brain homogenates (25 µL) by the addition of 100 µL of Ellman’s reagent and 190 µL of propionylthiocholine iodide (75 mM). The reaction mixture was incubated at 25°C. Absorbance (412 nm) readings were obtained after 60 min. The enzymatic reaction was stopped by the addition of 1.0 mL of quinidine sulfate (0.5%). A blank reading was obtained for each reaction mixture without incubation. Each absorbance reading was performed in duplicate.
Inhibition of AChE and BuChE activity
Electric eel AChE and horse serum BuChE were used in these experiments. Neostigmine (10 nM–10 µM) or the extracts (0–1.6 mg/mL), 50 µL of Ellman’s reagent, 50 µL of propionylthiocholine iodide (0.13 mM), 50 µL of the enzyme (AChE, 0.02 U/mL, BuChE, 0.03 U/mL), and AE or AKE (0–1.6 mg/mL) were combined and incubated at 25°C. Absorbance (412 nm) readings were obtained after 60 min. A blank reading was obtained for each reaction mixture without incubation. Each absorbance reading was performed in triplicate.
Protein assay
Total protein concentrations were determined as described by CitationBradford (1976). Bovine serum albumin was used as a standard.
Data analysis
The graphs shown here are plotted as cholinesterase activity (CA, %), although the data in the results section are expressed as cholinesterase inhibition (CI). The CI was calculated as 100% − CA%. The potencies (IC50) were determined by nonlinear regression. Data from the text and illustrations correspond to the mean [confidence interval] potency and mean ± SEM maximal inhibition (Emax). Values were compared using a Student’s one-tailed t-test or one-way ANOVA followed by Bonferroni’s test when appropriate. A significance level of 5% was used for all analyses. GraphPad Prism version 3.0 was used for all tests.
Results
Inhibitory effects of AE and AKE evaluated in vitro in mouse brains
The inhibition of cholinesterases by AE was significant and dose-dependent. Nonlinear regression revealed an IC50 of 0.57 [0.43–0.75] mg/mL (R2 = 0.79) for AE. The maximum inhibition (Emax) was 89.0 ± 1.5%. AKE prepared from AE inhibited brain CA in a significant and dose-dependent manner. AKE displayed an IC50 of 0.52 [0.39–0.70] mg/mL (R2 = 0.84) and an Emax of 92.2 ± 2.6%. No significant differences between the potency or Emax values of AE and AKE were observed (t-test, p > 0.05). Neostigmine, which was used as a positive control, inhibited cholinesterase in a concentration-dependent manner with an IC50 of 0.36 [0.25–0.48] µM (R2 = 0.88) and an Emax of 95.1 ± 0.8%. These results are shown in .
Figure 1. In vitro anticholinesterase activity (CA) of aqueous extract (AE) (A), alkaloid-rich extract (AKE) (B), and neostigmine bromide (C) in the mouse cortex. Concentration-response curves were performed for AE (0–1.6 mg/mL), AKE (0–1.6 mg/mL), and the positive control neostigmine (10 nM–10 µM). Enzyme activity was measured using Ellman’s method with slight modifications. Mouse brain homogenates were used as the source of cholinesterase. Data are expressed as the percent CA vs. the log inhibitor concentration (mg/mL) and represent the means ± SEM of four independent experiments performed in duplicate. Graphs are plotted as the cholinesterase activity (CA, %), although the data in the results section are expressed as the cholinesterase inhibition (CI). The CI was calculated as 100% − CA%.
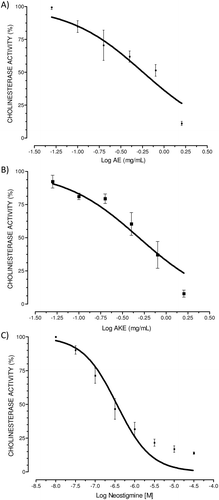
Inhibitory effects of AE and AKE evaluated using ex vivo experiments
AE significantly inhibited mouse cortical cholinesterase (p < 0.05, 32.2 ± 3.9% of inhibition) at a dose of 400 mg/kg after acute oral administration. AKE also significantly inhibited cortical cholinesterase after oral treatment at doses of 100 mg/kg (p < 0.05), 200 mg/kg (p < 0.05) and 400 mg/kg (p < 0.001). The percentages of inhibition were 16.5 ± 3.7%, 16.5 ± 6.8%, and 29.6 ± 3.2% for doses of 100, 200, and 400 mg/kg, respectively. Neostigmine bromide (0.1–10 mg/kg) significantly inhibited brain cholinesterases (p < 0.001, all tested doses). The percentages of inhibition were 35.4 ± 5.6%, 37.9 ± 5.6%, 50.5 ± 3.2% for doses of 0.1, 1.0, and 10 mg/kg, respectively. These results are shown in .
Figure 2. Ex vivo anticholinesterase activity of AE (A), AKE (B), and neostigmine bromide (C) in the mouse cortex. Mice were orally treated with AE or AKE (100, 200, and 400 mg/kg) or neostigmine as the positive control (0.1, 1.0, and 10.0 mg/kg). Enzyme activity was measured using Ellman’s method with slight modifications. Homogenates of mouse brains from animals that were sacrificed 4 h after each treatment were used as the source of cholinesterase. Data are expressed as the percent CA vs. the dose of the inhibitor (mg/kg) and represent the means ± SEM of four independent experiments performed in duplicate. Graphs are plotted as the cholinesterase activity (CA, %), although the data in the results section are expressed as the CI. The CI was calculated as 100% − CA%. *p < 0.05, **p < 0.01, ***p < 0.001 vs. the vehicle control, ANOVA, and post hoc Bonferroni tests.
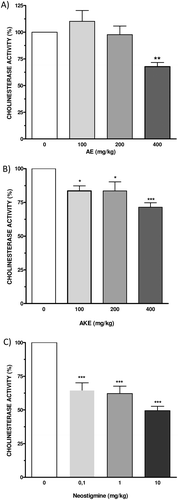
Inhibitory effects of AE and AKE on AChE and BuChE activities
The inhibitory effects of AE and AKE on electric eel AChE and horse serum BuChE were evaluated to better characterize the inhibition of ChE activity. AE and AKE inhibited AChE and BuChE in a concentration-dependent fashion (). The inhibitory action of AE on AChE was approximately 5.3 times stronger than on BuChE (AChE: IC50 = 0.56 [0.38–0.81] mg/mL, R2 = 0.99; BuChE: IC50 = 2.95 [1.51–5.76] mg/mL, R2 = 0.99). AKE also inhibited AChE (IC50 = 0.87 [0.60–12.5] mg/mL, R2 = 0.99) more effectively than BuChE (IC50 = 2.67 [0.87–8.11] mg/mL, R2 = 0.96). The inhibitory action of AKE on AChE was approximately 3.1 times stronger than on BuChE.
Figure 3. Inhibitory effects of AE or AKE (A), and neostigmine bromide (B) on electric eel acetylcholinesterase activity and horse serum butyrylcholinesterase activity. Enzyme activity was measured using Ellman’s method with slight modifications. Data are expressed as the percent CA vs. the log of the inhibitor concentration (mg/mL or M) and represent the means ± SEM of two independent experiments performed in triplicate. Graphs are plotted as the cholinesterase activity (CA, %), although the data in the results section are expressed as the CI. The CI was calculated as 100% − CA%.
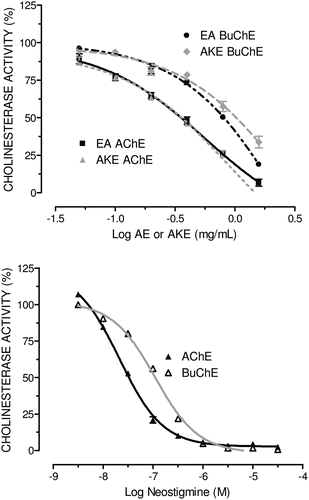
Discussion
The present study investigated the anticholinesterase activity of the Brazilian medicinal plant, EV. In vitro assays were used to demonstrate whether the plant AE inhibited mouse brain cholinesterase. AE significantly reduced the CA in a concentration-dependent manner.
We hypothesized that the alkaloids found in the AE could partially account for the anticholinesterase activity of EV, because several alkaloids inhibit cholinesterase and because Erythrina species are well known for their alkaloid content. The leaf AKE, which was prepared from AE, significantly inhibited mouse brain cholinesterase in a concentration-dependent manner in vitro. No significant differences in the IC50 values for AE and AKE were observed, indicating that they have similar potencies as cholinesterase inhibitors. These results suggest that the anticholinesterase activity of EV is located primarily in the alkaloid fraction.
Aging societies worldwide have been confronted with a dramatic increase in neurodegenerative diseases, such as AD. Clinical experience has demonstrated that cholinesterase inhibitors are valuable therapeutic tools in the treatment of the cognitive symptoms of AD. However, drugs must cross the blood-brain barrier to act within the central nervous system and to combat the cognitive symptoms of AD (CitationBallard et al., 2011). The anticholinesterase activity of AE and AKE was evaluated in an ex vivo experiment to examine whether EV leaf extracts acted centrally. In these studies, mice treated with oral AE exhibited significant CI in the brain. This result indicates that active EV compounds in the AE penetrated into the central nervous system to act centrally. Furthermore, AKE demonstrated significant anticholinesterase activity in the ex vivo experiment, reinforcing the hypothesis that EV alkaloids play an essential role in anticholinesterase activity.
The activity of AChE can decrease by as much as 85% during the course of severe AD, but BuChE activity remains unaltered or may increase. Therefore, the use of mixed AChE and BuChE inhibitors or BuChE-selective inhibitors to combat AD cognitive symptoms is a rational approach. Selective inhibitors of BuChE are not available for clinical testing, but experimental evidence of the therapeutic benefits of dual BuChE and AChE inhibitors in AD patients has been reported previously (CitationLane et al., 2006). A dual inhibitory effect of AE and AKE was detected in the work presented here.
People who develop AD are more likely to suffer from agitation and anxiety than the general population. Therefore, it is equally important to properly treat both behavioral and psychological symptoms to improve the quality of life and well-being of AD patients (CitationMega et al., 1996). The use of phytopharmaceuticals for dementia therapy represents an important strategy because of their multifaceted approach. Anxiolytic and sedative effects for several extracts from EV leaves have been reported over the last decade (CitationDantas et al., 2004; CitationMarchioro et al., 2005). The central anticholinesterase activity and the previously reported sedative and anxiolytic effects of EV leaves strongly suggest that this species can be used to combat both the cognitive and agitation symptoms in AD patients.
Conclusions
In summary, the results of this work indicate that EV had anticholinesterase activity. In addition, EV-active compounds in the aqueous and alkaloid leaf extracts crossed the blood-brain barrier and inhibited cholinesterase in the central nervous system of mice. Our results suggest that EV leaves possess therapeutic potential as a centrally active anticholinesterase medicine. However, further studies are required to establish the therapeutic value of EV leaves for memory improvement. Therefore, the potential use of EV leaf extracts as a dual inhibitor of AChE and BuChE is proposed.
Acknowledgments
The authors gratefully acknowledge Professor Adauto Souza Ribeiro for the identification of the plant material. The authors are also grateful for the financial support of student scholarships that were provided to authors Wanderson P. Santos and Ana Carla S. Carvalho by the Conselho Nacional de Desenvolvimento Tecnológico (CNPq), an agency linked to the Brazilian Ministry of Science and Tecnologia (MCT). The contents are solely the responsibility of the authors and do not necessarily represent the views of the funding agency.
Declaration of interest
The authors report no conflicts of interest.
References
- Akhondzadeh S, Noroozian M, Mohammadi M, Ohadinia S, Jamshidi AH, Khani M. (2003a). Salvia officinalis extract in the treatment of patients with mild to moderate Alzheimer’s disease: A double blind, randomized and placebo-controlled trial. J Clin Pharm Ther, 28, 53–59.
- Akhondzadeh S, Noroozian M, Mohammadi M, Ohadinia S, Jamshidi AH, Khani M. (2003b). Melissa officinalis extract in the treatment of patients with mild to moderate Alzheimer’s disease: A double blind, randomised, placebo controlled trial. J Neurol Neurosurg Psychiatr, 74, 863–866.
- Ballard C, Gauthier S, Corbett A, Brayne C, Aarsland D, Jones E. (2011). Alzheimer’s disease. Lancet, 377, 1019–1031.
- Bradford MM. (1976). A rapid and sensitive method for the quantitation of microgram quantities of protein utilizing the principle of protein-dye binding. Anal Biochem, 72, 248–254.
- Craveiro ACS, Carvalho DMM, Nunes RS, Fakhouri R, Rodrigues SA, Teixeira-Silva F. (2008). Toxicidade aguda do extrato aquoso de folhas de Erythrina velutina em animais experimentais. Brazilian J Pharmacog, 18, 739–743.
- Dantas MC, De Oliveira FS, Bandeira SM, Batista JS, Silva CD Jr, Alves PB, Antoniolli AR, Marchioro M. (2004). Central nervous system effects of the crude extract of Erythrina velutina on rodents. J Ethnopharmacol, 94, 129–133.
- Ellman GL, Courtney KD, Andres V Jr, Feather-Stone RM. (1961). A new and rapid colorimetric determination of acetylcholinesterase activity. Biochem Pharmacol, 7, 88–95.
- European Treaty Series (2005). European convention for the protection of vertebrate animals used for experimental and other scientific purposes. European Treaty Series, Strasbourg, ETS-123. Available at: http://conventions.coe.int/Treaty/en/Treaties/html/123.htm. Accessed on 30 June 2011.
- Lane RM, Potkin SG, Enz A. (2006). Targeting acetylcholinesterase and butyrylcholinesterase in dementia. Int J Neuropsychopharmacol, 9, 101–124.
- Marchioro M, Blank M, de F, Mourão RH, Antoniolli AR.. (2005). Anti-nociceptive activity of the aqueous extract of Erythrina velutina leaves. Fitoterapia, 76, 637–642.
- Matos FJA. (1997). O formulário fitoterápico do professor Dias da Rocha, Universidade Federal do Ceará Edições, Fortaleza, Brazil.
- Mega MS, Cummings JL, Fiorello T, Gornbein J. (1996). The spectrum of behavioral changes in Alzheimer’s disease. Neurology, 46, 130–135.
- Mukherjeea PK, Kumarb V, Malb M, Houghtona PJ. (2007). Acetylcholinesterase inhibitors from plants. Phytomedicine, 14, 289–300.
- Teske M, Trentini AMM. (1995). Herbarium: Conpêndio de Fitoterapia, Herbarium Laboratório Botânico, Curitiba, Brazil.
- Weinmann S, Roll S, Schwarzbach C, Vauth C, Willich SN. (2010). Effects of Ginkgo biloba in dementia: Systematic review and meta-analysis. BMC Geriatr, 10, 14.
- Wang R, Yan H, Tang XC. (2006). Progress in studies of huperzine A, a natural cholinesterase inhibitor from Chinese herbal medicine. Acta Pharmacol Sin, 27, 1–26.