Abstract
Context: Mangiferin, a natural bioactive xanthone C-glycoside, is widely present in medicinal plants like the leaf of Mangifera indica L. (Anacardiaceae). It has been reported that mangiferin possesses a variety of biological activities, including antidiabetic, hepatoprotective, anti-inflammatory, antioxidant, and anticarcinogenic.
Objective: The hypouricemic effect and xanthine oxidoreductase (XOR) inhibitory activity of mangiferin were investigated here for the first time.
Materials and methods: The hypouricemic effect of mangiferin was investigated in normal and hyperuricemic mice induced by potassium oxonate. Mangiferin at a dose of 0.75–100.0 mg/kg was given intragastrically to mice. The serum urate levels were determined using the phosphotungstic acid method. The hepatic activities of xanthine dehydrogenase (XDH) and xanthine oxidase (XOD) in hyperuricemic mice were assayed using commercially available kits.
Results: The results showed that mangiferin at a dose of 1.5, 3.0, and 6.0 mg/kg significantly reduced the serum urate levels (148.7 ± 37.8, 142.2 ± 44.5, 121.7 ± 21.7 µmmol/L) in hyperuricemic mice, compared with untreated hyperuricemic mice (201.8 ± 71.2 µmmol/L). However, mangiferin did not decrease the serum urate levels in normal mice until mangiferin was up to 100 mg/kg. In addition, the hepatic activities of XDH in hyperuricemic mice were significantly decreased by mangiferin, while no changes of XOD were observed. Acute toxicity study in mice showed that mangiferin was very safe at a dose of up to 25 g/kg.
Discussion and conclusion: These findings demonstrate that mangiferin has the potential to be developed as a new therapeutic agent for the treatment of hyperuricemia and gout.
Introduction
Gout is a disorder caused by deposition of monosodium urate crystals in joints and other tissues as a result of extracellular urate supersaturation (CitationHall et al., 1967; CitationCampion et al., 1987). Hyperuricemia, a concentration of urate in serum above the limit of solubility (≤ 7.0 mg/dL), is the most important risk factor for the development of gout (CitationShoji et al., 2004) and occurs as a result of increased uric acid production, impaired renal uric acid excretion, or a combination of these mechanisms. Clinically reported, the key factor uric acid is related not only to an increased risk of gout, but also to an increased risk of cardiovascular disorder, nephrolithiasis, diabetes, obesity, and dyslipidemia (CitationFang & Alderman, 2000; CitationHayden & Tyagi, 2004; CitationNakagawa et al., 2006).
The treatment of gout entails several approaches. Apart from the symptomatic relief of pain and swelling associated with inflammation, drugs that could help to reduce the elevated uric acid level in the blood are employed as therapeutic agents. These include xanthine oxidase (XOD) inhibitors, of which allopurinol is the most often prescribed. Inhibitors of renal urate reabsorption such as benzbromarone are also employed as hypouricemic agents. However, these existing antihyperuricemic agents possess some undesirable effects such as hypersensitivity toward allopurinol (CitationBomalaski & Clark, 2004) and hepatotoxicity associated with benzbromarone (CitationSchlesinger, 2004). Fortunately, febuxostat, a novel nonpurine XOD inhibitor, has received approval for the treatment of hyperuricemia in gout patients in USA and Europe (CitationReinders & Jansen, 2010). Febuxostat is able to selectively inhibit XOD with a mode of mixed-type inhibition (CitationHair et al., 2008). However, drugs available for treatment of gout patients with hyperuricemia are still limited. In addition, hyperuricemia is present in 5–30% of the general population and seems to be increasing worldwide. Therefore, there is an obvious need for novel agents or therapeutic strategies that could act on the physiological regulation of uric acid levels and prevention of uric acid-related diseases.
Mangiferin (), a natural bioactive xanthone C-glycoside, is widely present in medicinal plants like the leaf of Mangifera indica L. (Anacardiaceae). It has been reported that mangiferin possesses a wide variety of biological activities, such as antidiabetic (CitationIchiki et al., 1998), hepatoprotective (CitationYoshikawa et al., 2002), anti-inflammatory, analgesic, antioxidant, and anticarcinogenic (CitationYoshimi et al., 2001; CitationGarcía et al., 2002). Herein, we reported the hypouricemic effect of mangiferin in the normal and hyperuricemic mice induced by potassium oxonate, as well as the activities of xanthine dehydrogenase/oxidase (XDH/XOD) in the mouse liver.
Materials and methods
Reagents
Potassium oxonate was obtained from Sigma-Aldrich (Shanghai, PR China; http://www.sigmaaldrich.com/china-mainland.html). Allopurinol and benzbromarone were the products of Guangdong P.D. Pharmaceutical Co., Ltd (Guangdong, PR China; http://www.pdpharm.com/index.asp). and Heumann Pharma GmbH (Nuremberg, Germany), respectively. Uric acid kit and XDH/XOD kit were purchased from Nanjing Jiancheng Bioengineering Institute (Nanjing, PR China) and R&D (Taiwan, PR China), respectively. Other reagents were of analytical grades made in PR China.
Preparation of mangiferin
Mangiferin (purity >90% by HPLC) was isolated from the leaves of M. indica according to the method described previously (CitationGe et al., 2011). The EtOH extract of the leaves of M. indica was partitioned between EtOAc and water to afford EtOAc-soluble portion. Then the fraction of water was passed through macroporous resin and eluted successively with H2O and 95% EtOH. The 95% EtOH fraction was subjected to subsequent silica gel, Sephadex LH-20, and Rp-18 silica gel column chromatography to yield mangiferin.
Animals
Male Kunming mice, weighing 18–22 g, were purchased from the Experimental Animal Center, Kunming Medical University (Certificate No. SCXK 2005-0008). The animals were housed on a constant 12 h light/dark cycle in a temperature controlled central animal facility (18–22°C) and allowed free access to solid food and tap water. They were allowed one week to adapt to their environment before being used for experiments. All procedures were carried out in accordance with the Institute Ethical Committee for Experimental Use of Animals.
Effects of mangiferin on the serum uric acid levels in normal mice
Male animals were divided into eight groups with ten mice in each. The mice were treated intragastrically with 0.5% sodium carboxymethyl cellulose (CMC-Na), mangiferin (1.0, 3.0, 10.0, 30.0, and 100.0 mg/kg), benzbromarone (6.0 mg/kg), and allopurinol (1.0 mg/kg) dissolved in 0.5% CMC-Na, respectively. All drugs were given once daily for 5 days. Blood samples were collected from the mice via orbit vein bleeding after exposure to ethylic ether. Serum uric acid levels were determined using the phosphotungstic acid method (CitationCarroll et al., 1971).
Hypouricemic effects of mangiferin in hyperuricemic mice
A hyperuricemic mouse model was induced using the uricase inhibitor potassium oxonate (CitationYonetani & Iwaki, 1983; CitationYamada et al., 1999; CitationKong et al., 2002). Male mice were divided into several groups. The normal control mice and untreated hyperuricemic control mice were treated with 0.5% CMC-Na, whereas other hyperuricemic mice were given with mangiferin, benzbromarone, or allopurinol dissolved in 0.5% CMC-Na, respectively. All drugs were given intragastrically based on body weight measured immediately prior to each dose. The last dosage was given at 1 h after injection i.p. with potassium oxonate (450 mg/kg) to increase the serum uric acid level. Blood samples were collected from the mice via orbit vein bleeding after exposure to ethylic ether. Serum uric acid levels were determined using the phosphotungstic acid method (CitationCarroll et al., 1971). Mouse liver was excised, frozen immediately and stored at −20°C for measurement of the XDH/XOD activities. Food was withdrawn from the animals 2 h prior to collection of blood sample.
Assays of liver XDH/XOD activities
The frozen tissue samples were homogenized in 9 volumes of 80 mM sodium pyrophosphate buffer (pH 7.4). The homogenate was then centrifuged at 3000 rpm for 10 min, the lipid layer was carefully removed and the resulting supernatant fraction was further centrifuged at 10,000 rpm for 60 min at 4°C. The supernatant was used to assay the XDH/XOD activities using commercially available kits.
Acute toxicity studies
Acute toxicity studies were performed on Kunming mice with both sexes. Control and treated groups consisted of 20 animals each, half male and half female. The treated group received intragastrically mangiferin (25 g/kg) and the control group received 0.5% CMC-Na. Animals were observed carefully at 30, 60, 120, 240, and 360 min after treatment. Mortality, body weights, and behavior of the mice were observed and recorded daily for 14 days. Possible macroscopic changes in the treated group were compared with those of the control group.
Statistical analysis
Values are expressed as mean ± SEM. Data were analyzed using one-way analysis of variance followed by the Dunnett’s multiple comparison tests using GraphPad Prism version 4.0 software to determine the level of significance. p < 0.05 was considered statistically significant.
Results
Effects of mangiferin on serum urate levels in normal mice
As shown in , no significant difference was observed in the serum urate levels of the normal mice that received mangiferin at the doses of 1.0, 3.0, 10.0, or 30.0 mg/kg, compared with the normal control group, there was no significance value. Administration of mangiferin at the dose of 100.0 mg/kg and allopurinol at 1.0 mg/kg significantly decreased the serum urate levels of the normal mice, compared with the untreated normal mice (p < 0.05).
Dose-dependent effects of mangiferin on serum urate levels in hyperuricemic mice
The dose-dependent effects of mangiferin on the serum uric acid levels in the hyperuricemic mice were investigated. illustrates the changes of the concentration of the serum urate in different experimental groups after administration of five doses. Uricase inhibitor potassium oxonate treatment caused hyperuricemia in mice by comparing with the results in normal control mice (p < 0.05). Mangiferin, given intragastrically at a dose of 1.5, 3.0, and 6.0 mg/kg (3.5, 7.0, 14.0 µmol/kg) twice a day, significantly decreased the serum urate levels of hyperuricemic mice, in a dose-dependent manner, when compared to untreated hyperuricemic mice (p < 0.05), which was slightly less than that of allopurinol and as potent as that of benzbromarone at the same molar dose. This result suggested that mangiferin has marked hypouricemic action.
Time-dependent effects of mangiferin on the serum urate levels in the hyperuricemic mice
As shown in , the normal control and hyperuricemic control mice were intragastrically administered with 0.5% CMC-Na. The other mice were dosed once daily for 1, 3, and 7 days at 3.0 mg/kg (7.0 µmol/kg) for mangiferin, 6.0 mg/kg (14.0 µmol/kg) for benzbromarone, and 1.0 mg/kg (7.0 µmol/kg) for allopurinol, respectively. The results showed that the hypouricemic effect of mangiferin was time-dependent in the hyperuricemic mice. One-day, three-day, or seven-day administration of mangiferin at a dose of 3.0 mg/kg effectively reduced the serum urate levels in mice compared to the hyperuricemic control group. A single dose of 3.0 mg/kg was able to reduce the serum urate levels, although it was not as effective as allopurinol at the same molar dose (p < 0.05). In addition, mangiferin was found to be as effective as benzbromarone at 6.0 mg/kg (14.0 µmol/kg) and the onset of mangiferin was shorter than that of benzbromarone.
Figure 4. Hypouricemic effects of intragastric mangiferin on serum urate levels in the hyperuricemic mice induced by uricase inhibitor potassium oxonate: a time-course study. Data are expressed as means ± SEM, n = 10. ap < 0.05 vs. control group; bp < 0.05 vs. hyperuricemic group; cp < 0.05 vs. allopurinol group (Dunnett’s test).
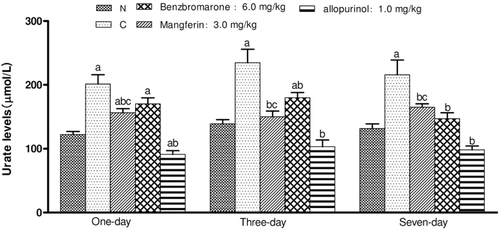
Effects of mangiferin on serum uric acid levels and liver XDH/XOD activities in the hyperuricemic mice
illustrates the mangiferin given by intragastric administration at the dosage of 3.0, 6.0, 15, 30 mg/kg, allopurinol at 10 mg/kg twice a day for 5 doses significantly attenuated the serum urate level of hyperuricemic mice, when compared to untreated hyperuricemic mice (p < 0.05). The hypouricemic action of mangiferin was slightly less than that of allopurinol and as potent as that of benzbromarone at the same molar dose, but there was no significant difference.
Table 1. Effects of mangiferin on serum urate levels and liver XDH/XOD activities in the hyperuricemic mice.
The effects of mangiferin on the activities of XDH/ XOD in the liver of hyperuricemic mice are shown in . It was found that the liver XDH activities were significantly inhibited by mangiferin after administration of 5 doses, while not by allopurinol. The reduction in enzyme activities was about 16 and 17% by mangiferin at the dose of 15, 30 mg/kg, respectively. In contrast, mangiferin did not decrease the activities of XOD in the liver of hyperuricemic mice. Administration of allopurinol markedly reduced the XOD activities by 24% in mouse liver.
Acute toxicity studies
An acute toxicity study revealed that animals treated intragastically with mangfrin at a dose of 25.0 g/kg did not show any significantly abnormal signs, behavioral changes, body weight changes, or macroscopic findings at any time of observation. There was no mortality observed during the 14 days of the experiments.
Discussion
Oxonate, a selectively competitive uricase inhibitor, blocks the effect of hepatic uricase, and produces hyperuricemia in rodents (CitationStavric et al., 1975; CitationHall et al., 1990). Oxonate-treated mice can serve as a useful animal model not only to evaluate drugs that affect serum urates but also to evaluate possible therapeutic agents in certain disorders associated with uric acid. The aim of the present study is to investigate hypouricemic effects of mangiferin on the serum uric acid levels in the normal and hyperuricemic mice induced by potassium oxonate. The effects of mangiferin on XDH/XOD activities in mouse liver were also investigated.
The results showed that oral administration of mangiferin was able to significantly decrease the serum urate levels of hyperuricemic mice in a dose-dependent manner. The hypouricemic action was slightly less than that of allopurinol and as potent as that of benzbromarone at the same molar dose. It is worth mentioning that mangiferin markedly reduced serum urate levels in hyperuricemic mice although it did not happen in normal animals until the dosage reached 100 mg/kg. In contrast, allopurinol exerted its hypouricemic action in both normal and hyperuricemic mice. Indeed, elevated levels of uric acid in the circulation could give rise to gout and possibly other pathological conditions (CitationLiese et al., 1999; CitationTomita et al., 2000; CitationPuddu et al., 2001). But, conversely, the antioxidant action of uric acid is also well documented, particularly its ability to inhibit DNA damage (CitationSingh et al., 1998; CitationBurkhardt et al., 2001). Thus, a low level of uric acid in the circulation does not only reflect an inadvertent consequence of steady-state purine metabolism, but might actually be serving some important biological purposes. Thus, excessive lowering of the uric acid level in the circulation beyond that of the normal range might even be counterproductive. This feature of mangiferin makes it an attractive candidate for the treatment of hyperuricaemia, particularly if the compound is to be taken on a long-term basis.
The onset of hypouricemic action of mangiferin appeared to be as fast as that of allopurinol with the onset time being less than 1 h after the administration, although the action was less potent than that of allopurinol. After 3 days or more of drug administration, benzbromarone was observed to reduce the serum urate levels in hyperuricemic mice, suggesting that the onset of hypouricemic action was slower than that of mangiferin.
Mammalian XOD (EC 1.1.3.22) and XDH (EC 1.1.1.204), are interconvertible forms of the same gene product (CitationIchida et al., 1993; CitationCazzaniga et al., 1994; CitationMinoshima et al., 1995), known as xanthine oxidoreductase (XOR). XOR is a highly versatile flavoprotein enzyme, ubiquitous among species (from bacteria to human) and within the various tissues of mammals. The enzymes catalyze the hydroxylation of hypoxanthine and xanthine, the last two steps in urate biosynthesis. Therefore, XDH/XOD has been recognized as an important target of drugs against hyperuricemia and gout. Allopurinol, a potent XOD inhibitor with a purine backbone, has been used clinically for more than 40 years. It is hydroxylated by the enzyme to alloxanthine (oxypurinol), which, once formed, coordinates tightly to the reduced form of the molybdenum center specifically, replacing the Mo-OH group of native enzyme. In the current study, mangiferin showed a significant inhibitory activity on XDH of the hyperuricemic mouse liver in a dose-dependent manner, but not on XOD, which was different from allopurinol. This observation suggested that the hypouricemic effect of mangiferin could be explained, at least in part, by blocking of liver of XDH activities. It is the first demonstration that the in vivo hypouricaemic action of mangiferin was associated with the ability to decrease liver XDH activities in animals orally administered with the compound. Notably, the inhibitory effect of mangiferin on the liver XDH activities of the hyperuricemic mice was not observed in the 3.0 and 6.0 mg/kg mangiferin treated groups. Obviously, the hypouricaemic action of mangiferin might involve other mechanisms apart from inhibiting the XDH activity, which needs further investigation.
Conclusion
The present study showed that mangiferin significantly reduced the serum urate levels in the hyperuricemic mice caused by potassium oxonate. These hypouricemic effects of mangiferin could be explained, at least partly, by inhibiting the hepatic activities of XDH. More importantly, mangiferin, as a natural product, did not show any toxic reactions in mice at a dose of 25.0 g/kg, indicating that it might be much safer than allopurinol. Therefore, mangiferin is worthy of further development as a potential hypouricemic agents.
Declaration of interest
The work is supported by the funds from NSFC (30960453) and Yunnan Provincial Science Foundation (20080C021, 2008IF009). The authors declare no conflicts of interest.
References
- Bomalaski JS, Clark MA. (2004). Serum uric acid-lowering therapies: Where are we heading in management of hyperuricemia and the potential role of uricase. Curr Rheumatol Rep, 6, 240–247.
- Burkhardt S, Reiter RJ, Tan DX, Hardeland R, Cabrera J, Karbownik M. (2001). DNA oxidatively damaged by chromium(III) and H(2)O(2) is protected by the antioxidants melatonin, N(1)-acetyl-N(2)-formyl-5-methoxykynuramine, resveratrol and uric acid. Int J Biochem Cell Biol, 33, 775–783.
- Campion EW, Glynn RJ, DeLabry LO. (1987). Asymptomatic hyperuricemia. Risks and consequences in the Normative Aging Study. Am J Med, 82, 421–426.
- Carroll JJ, Coburn H, Douglass R, Babson AL. (1971). A simplified alkaline phosphotungstate assay for uric acid in serum. Clin Chem, 17, 158–160.
- Cazzaniga G, Terao M, Lo Schiavo P, Galbiati F, Segalla F, Seldin MF, Garattini E. (1994). Chromosomal mapping, isolation, and characterization of the mouse xanthine dehydrogenase gene. Genomics, 23, 390–402.
- Fang J, Alderman MH. (2000). Serum uric acid and cardiovascular mortality the NHANES I epidemiologic follow-up study, 1971–1992. National Health and Nutrition Examination Survey. JAMA, 283, 2404–2410.
- García D, Delgado R, Ubeira FM, Leiro J. (2002). Modulation of rat macrophage function by the Mangifera indica L. extracts Vimang and mangiferin. Int Immunopharmacol, 2, 797–806.
- Ge DD, Zhang Y, Liu EW, Wang T, Hu LM. (2011). Chemical constituents of Mangifera indica leaves (I). Chin Trad Herb Drugs, 42, 428–431.
- Hair PI, McCormack PL, Keating GM. (2008). Febuxostat. Drugs, 68, 1865–1874.
- Hall AP, Barry PE, Dawber TR, McNamara PM. (1967). Epidemiology of gout and hyperuricemia. A long-term population study. Am J Med, 42, 27–37.
- Hall IH, Scoville JP, Reynolds DJ, Simlot R, Duncan P. (1990). Substituted cyclic imides as potential anti-gout agents. Life Sci, 46, 1923–1927.
- Hayden MR, Tyagi SC. (2004). Uric acid: A new look at an old risk marker for cardiovascular disease, metabolic syndrome, and type 2 diabetes mellitus: The urate redox shuttle. Nutr Metab (Lond), 1, 10.
- Ichida K, Amaya Y, Noda K, Minoshima S, Hosoya T, Sakai O, Shimizu N, Nishino T. (1993). Cloning of the cDNA encoding human xanthine dehydrogenase (oxidase): Structural analysis of the protein and chromosomal location of the gene. Gene, 133, 279–284.
- Ichiki H, Miura T, Kubo M, Ishihara E, Komatsu Y, Tanigawa K, Okada M. (1998). New antidiabetic compounds, mangiferin and its glucoside. Biol Pharm Bull, 21, 1389–1390.
- Kong L, Zhou J, Wen Y, Li J, Cheng CH. (2002). Aesculin possesses potent hypouricemic action in rodents but is devoid of xanthine oxidase/dehydrogenase inhibitory activity. Planta Med, 68, 175–178.
- Liese AD, Hense HW, Löwel H, Döring A, Tietze M, Keil U. (1999). Association of serum uric acid with all-cause and cardiovascular disease mortality and incident myocardial infarction in the MONICA Augsburg cohort. World Health Organization Monitoring Trends and Determinants in Cardiovascular Diseases. Epidemiology, 10, 391–397.
- Minoshima S, Wang Y, Ichida K, Nishino T, Shimizu N. (1995). Mapping of the gene for human xanthine dehydrogenase (oxidase) (XDH) to band p23 of chromosome 2. Cytogenet Cell Genet, 68, 52–53.
- Nakagawa T, Hu H, Zharikov S, Tuttle KR, Short RA, Glushakova O, Ouyang X, Feig DI, Block ER, Herrera-Acosta J, Patel JM, Johnson RJ. (2006). A causal role for uric acid in fructose-induced metabolic syndrome. Am J Physiol Renal Physiol, 290, F625–F631.
- Puddu PE, Lanti M, Menotti A, Mancini M, Zanchetti A, Cirillo M, Angeletti M, Panarelli W; Gubbio Study Research Group. (2001). Serum uric acid for short-term prediction of cardiovascular disease incidence in the Gubbio population study. Acta Cardiol, 56, 243–251.
- Reinders MK, Jansen TL. (2010). Management of hyperuricemia in gout: Focus on febuxostat. Clin Interv Aging, 5, 7–18.
- Schlesinger N. (2004). Management of acute and chronic gouty arthritis: Present state-of-the-art. Drugs, 64, 2399–2416.
- Shoji A, Yamanaka H, Kamatani N. (2004). A retrospective study of the relationship between serum urate level and recurrent attacks of gouty arthritis: evidence for reduction of recurrent gouty arthritis with antihyperuricemic therapy. Arthritis Rheum, 51, 321–325.
- Singh S, Farhan Asad S, Hadi SM. (1998). Uric acid inhibits l-DOPA-CU(II) mediated DNA cleavage. Neurosci Lett, 258, 69–72.
- Stavric B, Clayman S, Gadd RE, Hébert D. (1975). Some in vivo effects in the rat induced by chlorprothixene and potassium oxonate. Pharmacol Res Commun, 7, 117–124.
- Tomita M, Mizuno S, Yamanaka H, Hosoda Y, Sakuma K, Matuoka Y, Odaka M, Yamaguchi M, Yosida H, Morisawa H, Murayama T. (2000). Does hyperuricemia affect mortality? A prospective cohort study of Japanese male workers. J Epidemiol, 10, 403–409.
- Yamada H, Kotaki H, Furitsu H, Sawada Y, Iga T. (1999). Mechanism of the uricosuric action of the anti-inflammatory drug E3040 used to treat inflammatory bowel disease I: Study using a rat model of hyperuricemia. Biopharm Drug Dispos, 20, 77–83.
- Yonetani Y, Iwaki K. (1983). Effects of uricosuric drugs and diuretics on uric acid excretion in oxonate-treated rats. Jpn J Pharmacol, 33, 947–954.
- Yoshikawa M, Ninomiya K, Shimoda H, Nishida N, Matsuda H. (2002). Hepatoprotective and antioxidative properties of Salacia reticulata: Preventive effects of phenolic constituents on CCl4-induced liver injury in mice. Biol Pharm Bull, 25, 72–76.
- Yoshimi N, Matsunaga K, Katayama M, Yamada Y, Kuno T, Qiao Z, Hara A, Yamahara J, Mori H. (2001). The inhibitory effects of mangiferin, a naturally occurring glucosylxanthone, in bowel carcinogenesis of male F344 rats. Cancer Lett, 163, 163–170.